16 Mechanical Ventilation of the Neonate
Note 1: This book is written to cover every item listed as testable on all Entry Level Examination (ELE), Written Registry Examination (WRE), and Clinical Simulation Examination (CSE).
The listed code for each item is taken from the National Board for Respiratory Care’s (NBRC) Summary Content Outline for CRT (Certified Respiratory Therapist) and Written RRT (Registered Respiratory Therapist) Examinations (http://evolve.elsevier.com/Sills/resptherapist/). For example, if an item is testable on both the ELE and WRE, it will simply be shown as (Code: …). If an item is only testable on the ELE, it will be shown as (ELE code: …). If an item is only testable on the WRE, it will be shown as (WRE code: …).
Note 2: A review of the most recent Entry Level Examinations (ELE) has shown an average of one question (out of 140), or <1% of the exam, will cover continuous positive airway pressure (CPAP) or mechanical ventilation of the neonate. A review of the most recent Written Registry Examinations (WRE) has shown an average of two questions (out of 100), or 1% of the exam, will cover continuous positive airway pressure (CPAP) or mechanical ventilation of the neonate. Of the 10 tested scenarios of the Clinical Simulation Examination, expect one neonatal patient and one pediatric patient. CPAP or mechanical ventilation may be involved in both situations. Be sure to review neonatal and pediatric assessment items in Chapter 1.
MODULE A
1. Initiate and adjust an elevated baseline pressure: continuous positive airway pressure (CPAP) breathing (Code: IIID2d) [Difficulty: ELE: R, Ap; WRE: An]
a. Physiologic effects
Continuous positive airway pressure (CPAP) and positive end-expiratory pressure (PEEP) increase the patient’s functional residual capacity (FRC). In neonates, the most common cause of a decreased FRC is infant respiratory distress syndrome (RDS). This condition is caused by the lack of surfactant in the lungs of the premature neonate. The neonate with RDS has relatively airless lungs that are prone to atelectasis. This results in hypoxemia. In addition, each tidal volume breath requires a greater than normal inspiratory effort (Figure 16-1). The restoration of FRC in the neonate increases its Pao2, decreases the percentage of shunt, narrows the alveolar to arterial difference in oxygen, and reduces its work of tidal volume breathing. CPAP must be used with caution in neonates with persistent pulmonary hypertension (PPHN) of the newborn. An excessive amount of pressure in the alveoli compresses the capillary bed. This decreases pulmonary blood flow, which in turn increases blood flow through the patent ductus arteriosus and worsens the problem.
b. Indications, contraindications, and hazards
CPAP is indicated for any condition that results in an unacceptably low Pao2 secondary to a decreased FRC. Some neonates respond so well to CPAP that mechanical ventilation is not needed. In addition, CPAP has been used to keep open the airways of infants with tracheal malacia or other conditions in which the airways collapse abnormally. In general, contraindications include any CPAP-related condition that results in a worsening of the patient’s original status. Some neonates cannot tolerate CPAP and progressively hypoventilate as the pressure level is increased. Clinical judgment is needed to decide how high the PaCO2 should be allowed to rise before discontinuing the CPAP and beginning mechanical ventilation. In general, the PaCO2 should not be greater than 50 torr as long as the pH is at least 7.25. An absolute contraindication is apnea resulting in hypoxemia and hypotension. These infants should be mechanically ventilated. Box 16-1 gives a complete listing of indications, contraindications, and hazards.
c. Initiation
Before starting CPAP, a set of baseline arterial blood gases should be taken. Transcutaneous oxygen monitoring or pulse oximetry may be substituted in some clinical situations if oxygenation is the only parameter that must be measured. The neonate’s vital signs should also be recorded. Assemble the CPAP circuit and pressure device. The decision must be made whether to apply the CPAP above the epiglottis (Figures 16-2 and 16-3) or to intubate the infant and apply the CPAP within the trachea. Nasal CPAP (NCPAP) or nasopharyngeal tube CPAP (NP-CPAP) are both widely used to apply pressure from above the epiglottis. The neonate or infant must have an endotracheal tube placed to apply CPAP within the trachea. Among the factors to be considered are the neonate’s gestational age and weight, the amount of secretions that need to be suctioned, the pulmonary problem, and the likelihood of mechanical ventilation eventually becoming necessary. More mature and larger infants with few secretions and relatively stable pulmonary conditions will most likely have CPAP applied above the epiglottis by nasal prongs or nasopharyngeal tube. In contrast, less mature and smaller infants (less than 1000 to 1200 g) who need suctioning and have relatively unstable pulmonary conditions will probably be intubated. Mechanical ventilation can then be easily started if needed.
d. Monitor and adjust alarm settings (ELE Code: IIIG3g) [Difficulty: ELE: R, Ap, An]
Equipment should have visual and audible alarms and include the following features:
e. CPAP adjustment
Blood gases and vital signs must be evaluated at the starting CPAP level. The heart rate, blood pressure, and respiratory rate should be stable or improved. Wait at least 10 minutes after a change in CPAP before getting an arterial blood gases sample. See Table 16-1 for the recommended blood gas limits. In general, the Pao2 should be kept between 60 and 70 torr, PaCO2 less than 50 to 55 torr, and pH at least 7.25. If the Pao2 is too low and the patient’s vital signs are acceptable, the CPAP may be increased in a step of 1 to 2 cm water. The vital signs and blood gases should then be reevaluated. In addition, the neonate’s work of breathing can be indirectly assessed. Improved lung function will be demonstrated by seeing decreased respiratory rate, retractions, expiratory grunting, and nasal flaring. If necessary, the process of adding CPAP and reassessing the patient can be continued. The maximum CPAP level in a neonate is generally held to be 10 cm water; the maximum CPAP level in an infant is generally held to be 15 cm water.
TABLE 16-1 Commonly Recommended Blood Gas Goals for CPAP and Mechanical Ventilator Therapy
Age of Neonate | ||
---|---|---|
Less Than 72 Hours | Greater Than 72 Hours | |
Pao2 (torr) | 60-70 | 50-70 |
Ptco2 (torr) | Greater than 50,* less than 90* | Greater than 40,* less than 90* |
Spo2 | 92%-96% | 92-96% |
PaCO2 (torr) | 35-45† | 45-55 |
PtcCO2 (torr) | May be used after correlation with PaCO2 as discussed in Chapter 3. | |
pH | 7.25-7.45 | 7.25-7.45 |
NOTE: Keep the Pao2 no greater than 80 torr in the premature neonate to reduce the risk of retinopathy of prematurity.
* Ptco2 values may be used after they have been shown to correlate within 15% of the Pao2 from an arterial blood gas.
† With CPAP, this value may be increased to 50 to 55 torr as long as the pH is at least 7.25.
f. Independently initiate weaning from CPAP (Code: IIIF2i12) [Difficulty: ELE: R, Ap; WRE: An]
As the patient improves, it is necessary to reduce the CPAP level so as not to cause pulmonary barotrauma. The pressure level can be reduced in steps of about 2 cm water. The vital signs and blood gases should be reassessed after each step. The apparatus is usually removed when the CPAP level is down to 2 to 4 cm water. The infant is then placed into an oxyhood at the same oxygen percentage as before or 5% to 15% higher. If the infant has an endotracheal tube that is needed for suctioning or a secure airway, the pressure is usually left at 2 to 4 cm water. After extubation, the infant is placed into an oxyhood as before. Alternatively, the neonate may be weaned to a high flow nasal cannula and then to a traditional, low-flow nasal cannula. See Chapter 6 for more discussion on the high-flow nasal cannula.
MODULE B
1. Select a mechanical ventilation
a. Recommend changing the type of ventilator to be used on the patient (Code: IIIG3i) [Difficulty: ELE: R, Ap; WRE: An]
2. Adjust the ventilator settings.
a. Initiate and adjust continuous mechanical ventilator settings (Code: IIID2b) [Difficulty: ELE: R, Ap; WRE: An]
b. Recommend changes in mechanical ventilation to modify ventilator techniques (Code: IIIG3e) [Difficulty: ELE: R, Ap; WRE: An]
d. Sensitivity
1. Observe the patient for signs of ventilator-patient dyssynchrony (Code: IIIE5b) [Difficulty: ELE: R, Ap; WRE: An]
2. Independently adjust the ventilator to improve patient synchrony (Code: IIIF2i1) [Difficulty: ELE: R, Ap; WRE: An]
There is no sensitivity control on traditional continuous flow TPTV-type neonatal ventilators. The IMV mode is used with these units. Newer ventilators have the ability to sense a neonate’s respiratory effort and trigger a machine delivered breath. Some units sense a change in flow through the ventilator circuit when the neonate inspires. Other ventilators make use of electrocardiogram-type leads on the neonate’s chest to sense a change in electrical impedance (skin resistance) when the neonate makes an inspiratory effort. A child on a volume-cycled ventilator should have the sensitivity set at about −1 to −2 cm water pressure. No matter which method the ventilator uses to sense the patient’s respiratory effort, the neonate or child should not have to work hard to trigger a machine tidal volume.
f. I : E ratio
3. Indications for mechanical ventilation
All authors agree that apnea is an absolute indication for mechanical ventilation. A general indication is any condition that causes respiratory failure. This is usually documented by unacceptable arterial blood gases. Box 16-2 lists indications for mechanical ventilation.
BOX 16-2 Common Indications for the Initiation of Mechanical Ventilation
RESPIRATORY FAILURE
Although technically impractical to measure the time constant of ventilation at the bedside, the concept is important because it relates to two important clinical considerations during mechanical ventilation. First, it relates to the pressure that develops at the alveolar level as the tidal volume is delivered. For each time constant, progressively more of the peak inspiratory pressure (PIP) is applied within the alveoli (Figure 16-4). As can be seen, at three time constants, 95% of the PIP is applied to the alveoli. At five time constants, virtually the entire PIP is applied at the alveolar level. Second, the time constant relates to how rapidly the lung recoils to baseline (FRC) during an exhalation. As shown in Figure 16-4, it takes three time constants to exhale 95% and five time constants to completely exhale.
4. Initiation and adjustments based on the patient’s condition
a. Patients with normal cardiopulmonary function
Patients with normal cardiopulmonary function may need mechanical ventilation because of apnea from anesthesia, paralysis, or a neurologic condition. The initial TPTV ventilator parameters for this type of patient are listed in Box 16-3. Once mechanical ventilation is established, it is important to evaluate the patient’s blood gases, vital signs, breath sounds, and any other pertinent clinical information before changing any ventilator parameters.
BOX 16-3 Common Mechanical Ventilator Parameters for Neonates with Normal Lungs
As the patient recovers and begins to breathe spontaneously, it will probably be necessary to reduce the ventilator-delivered minute volume. This encourages the child to breathe more because the final goal is to completely wean and extubate the patient. The most accepted way to reduce the ventilator-delivered minute volume is to reduce the ventilator rate. A reduction of about 10% is a good starting place but must be tailored to meet the patient’s needs. The tidal volume is maintained as originally set. Obtain a set of blood gases in 10 to 20 minutes (or follow the transcutaneous or pulse oximetry values), and check the patient’s vital signs to see how well the adjustment is tolerated.
CALCULATION OF ESTIMATED TIDAL VOLUME DURING TPTV MECHANICAL VENTILATION
= inspiratory flow rate on the ventilator in mL/sec
It must be emphasized that this is only a calculated tidal volume. Leaks in the system, a decrease in the patient’s compliance, or an increase in the patient’s resistance decreases the true tidal volume. Conversely, an increase in the patient’s compliance or a decrease in the patient’s resistance increases the true tidal volume. Also, if the pressure limit is reached before the inspiratory time is completed, less volume than expected will be delivered. This is because part of the inspiratory time is spent as an inflation hold and no additional tidal volume is delivered (Figure 16-5). Finally, the infant must be completely passive during the delivery of the breath.
b. Patients with decreased lung compliance and normal airway resistance such as infant respiratory distress syndrome (RDS)
Although RDS in infants weighing less than 1000 g is the most common cause of decreased lung compliance with normal airway resistance, stiff lungs are also found in patients with other lung conditions such as pneumonia and pulmonary edema (Figure 16-6). The greatest challenge presented in the care of these infants is to oxygenate them without causing oxygen toxicity or pulmonary barotrauma. Common recommendations for the initial ventilator settings are listed in Box 16-4. As discussed earlier, blood gases, vital signs, and so forth must be monitored after the infant is placed on the ventilator. Any further adjustments can then be determined and evaluated by another set of blood gases and vital signs.
BOX 16-4 Common Mechanical Ventilator Parameters for Neonates with Low Compliance and Normal Resistance
The issue of time constants of ventilation helps to better explain the various options available for adjusting the ventilator. As presented earlier, the time constant of ventilation (Tc or time constant of the respiratory system TRS) is calculated as the product of compliance and resistance. For example, using the following values for a mechanically ventilated neonate with RDS, its time constant is calculated as
1. Administer oxygen
2. Increased inspiratory flow
Increasing the tidal volume should result in an increased Pao2 and is likely to reduce the PaCO2. If the pressure limit is reached, the delivered tidal volume is held in the lungs for the duration of the inspiratory time. This acts as an inflation hold and should also increase the Pao2. The mean airway pressure is raised by holding the tidal volume in the lungs. (See Figure 16-7 for the pressure waveforms seen during low-flow and high-flow conditions.) Under high-flow conditions, the pressure waveform takes on a characteristic square shape (square wave) because the pressure limit is reached. This pattern of ventilation is currently widely used with these types of patients.
3. Increased inspiratory time leading to inverse ratio ventilation
As with increasing the flow, increasing the inspiratory time increases the tidal volume until the pressure limit is reached. If the pressure limit is reached, any increased inspiratory time acts as inflation hold. This also raises the mean airway pressure and should result in an increased Pao2. (See Figure 16-5 for the pressure waveform change as the inspiratory time is increased.)
4. Initiate and adjust an elevated baseline pressure: positive end-expiratory pressure (PEEP) (Code: IIID2d) [Difficulty: ELE: R, Ap; WRE: An]
Positive end-expiratory pressure (PEEP) increases the patient’s functional residual capacity (FRC) by preventing a complete exhalation to the baseline (atmospheric) pressure. Because PEEP increases the FRC, it is more effective at increasing the Pao2 than increasing the tidal volume, inflation hold, or an inverse I : E ratio. See Table 16-1 for the recommended guidelines for arterial blood gas values in neonates. If the patient needs more than 50% oxygen to keep the desired Pao2, PEEP should be started; unless there is a contraindication to its use. Often, the proper level of therapeutic PEEP can result in an acceptable Pao2 with no more than 50% oxygen being inhaled.
5. Increased pressure limit
To deliver a larger tidal volume, it may become necessary to increase the pressure limit when it is reached by the peak inspiratory pressure (PIP). It may also be necessary to raise the pressure limit to restore the original tidal volume after the addition of PEEP. This is because the original tidal volume is reduced when PEEP is added and the PIP reaches the pressure limit. This is shown in Figure 16-8. To restore the original tidal volume, the pressure limit (and PIP) must be increased by the same amount as the added PEEP. It is important to remember that as PEEP is reduced, the pressure limit must also be reduced by the same amount. This maintains the original tidal volume.
7. Initiate and select appropriate settings for high-frequency ventilation (HFV) (Code: IIID4) [Difficulty: ELE: R, Ap; WRE: An]
High-frequency ventilation (HFV) involves the use of a ventilator respiratory rate that is much greater than commonly needed. The FDA defines HFV as a rate of more than 150/min. Because of this FDA definition of HFV, conventional neonatal TPTV ventilators are not considered high-frequency ventilators. Several manufacturers have developed ventilators capable of rates significantly greater than 150/min. One of these must be used when HFV is indicated. The FDA has also set some guidelines for the appropriate use of HFV. See Box 16-5 for the clinical indications for HFV. The most commonly encountered problem is with a premature neonate with infant respiratory distress syndrome (RDS), a homogenous lung disease causing low compliance. If the RDS patient is failing despite optimal conventional mechanical ventilation and has a mean airway pressure of greater than 15 cm water, high-frequency ventilation is indicated.
BOX 16-5 Common Clinical Indications for the Use of High-Frequency Ventilation in Infants and Children
Ventilator manufacturers have developed two different technologies for delivering FDA-defined HFV. The first is called high-frequency jet ventilation (HFJV). With HFJV, small jets of gas are directed down the patient’s endotracheal tube. These gas jets entrain additional gas into the tube. The two combined volumes of gas make up the patient’s tidal volume. The patient exhales passively because of normal lung recoil. The second method is called high-frequency oscillation (HFO) or high-frequency oscillatory ventilation (HFOV). With HFO, a pumping device (piston, diaphragm, or sound speaker) actively pushes a small tidal volume into the patient from a continuous flow of gas (called bias flow) passing through the circuit and by the endotracheal tube. HFO technology can deliver very high rates because the patient actively exhales the delivered tidal volume. This active exhalation is created by the back stroke of the pumping device that pulls the tidal volume out of the patient’s airways and lungs. See Table 16-2 for a comparison of HFV methods and characteristics.
Initial HFV settings depend on the patient’s diagnosis, clinical situation, current conventional ventilator settings, and the type of high-frequency ventilator to be used. Generally speaking, the initial HFV settings include a tidal volume large enough to see chest movement and a continuation of the patient’s original mean airway pressure, PEEP level, and oxygen percentage. The I : E ratio should be set at 1 : 2 and the high-frequency rate should be between 10 and 15 Hertz (Hz) depending on the infant’s body weight. (A Hz is defined as 1 respiratory cycle/sec; 60 respiratory cycles/min. For example, a rate of 600/min is 10/sec or 10 Hz.) After the patient is stabilized on HFV, arterial blood gases should be drawn and analyzed, vital signs assessed, and a chest radiograph obtained. In a patient without a pulmonary air leak, a chest radiograph finding of lung expansion to T8 to T9 on the right hemidiaphragm, without intercostal bulging, is believed to show the best lung volume. Once established, the HFV rate is seldom changed. Adjustments in tidal volume are made by increasing or decreasing what is referred to as either drive pressure (with HFJV) or amplitude (with HFO). Increasing drive pressure/amplitude increases the tidal volume and decreases the carbon dioxide level. The patient’s oxygenation should also improve as the mean airway pressure is increased. Decreasing drive pressure/amplitude decreases the tidal volume and increases the carbon dioxide level. If the patient’s oxygenation should decrease too much, additional therapeutic PEEP may be needed. See Table 15-1 for high-frequency ventilation guidelines with infant and adult patients. See Figure 16-9 for a protocol on initiation and adjustment of HFO. Figure 16-10 shows a protocol for weaning from HFO.
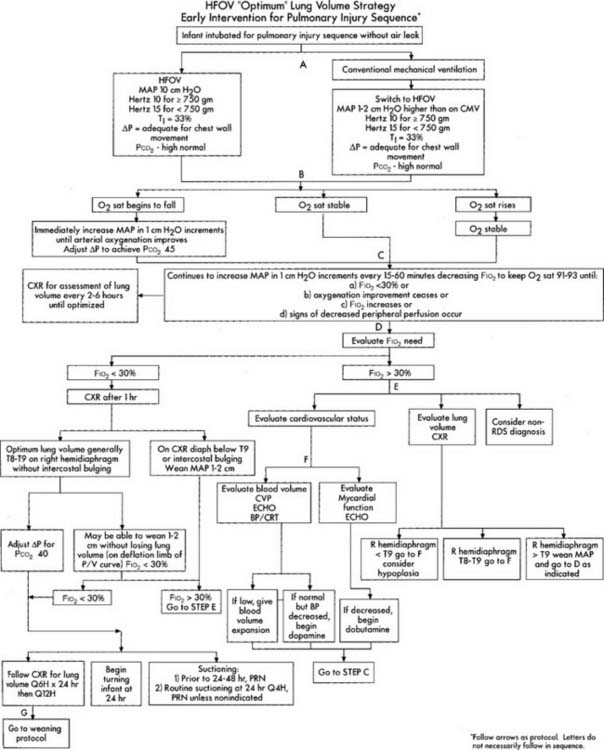
Figure 16-9 Optimum lung volume HFOV strategy flowchart.
(From Minton S, Gerstmann D, Stoddard R: Cardiopul Rev, Yorba Linda, Calif, 1995, Sensormedics Corp. PN 770118-001.)
c. Patients with increased airway resistance and normal lung compliance such as meconium aspiration syndrome (MAS)
Although meconium aspiration is a commonly seen cause of increased airway resistance, it is also seen in infants with excessive airway secretions or bronchospasm. These neonates usually have normal lung compliance and their clinical problem is getting enough air into and out of the lungs. The meconium or other obstruction causes uneven airflow and results in hypoxemia, air trapping, auto-PEEP, and an increased risk of barotrauma or volutrauma. Because of these issues, there are two key clinical goals. The first is to minimize turbulence during inspiration by reducing the inspiratory flow rate as much as possible. The second is to give a long enough expiratory time to prevent air trapping. Common recommendations for the ventilator settings are listed in Box 16-6. As discussed earlier, blood gases, vital signs, and so forth must be monitored after the infant is placed on the ventilator. Listen to the breath sounds to detect the end of exhalation and a pause before the start of the next inspiration. This is to ensure that the exhalation has been complete and there is no air trapping that would lead to auto-PEEP. Any further adjustments can then be determined and evaluated by another set of blood gases and vital signs.
Tc compliance (.005 L/cm water) × resistance(150 cm water/L/second because the infant is intubated and has an obstructive problem.)
d. Patients with persistent pulmonary hypertension of the newborn
4. Doppler echocardiography
Any infant who shows signs of PPHN, such as positive results to any of these tests or the need for more than 70% oxygen to prevent hypoxemia, should be considered for hyperventilation therapy. The goal is to reduce pulmonary artery hypertension and thus reduce shunting and improve the Pao2. This is accomplished by hyperventilating the patient to his or her critical PaCO2. Box 16-7 lists common ventilator parameters used to treat persistent pulmonary hypertension. Once the proper ventilator settings are found and the blood gas goals are met, the patient is maintained at this level for 1 or more days. It is often necessary to pharmacologically paralyze the infant with pancuronium bromide (Pavulon) or a similar drug to ensure that the patient’s breathing is synchronized with the ventilator. This is especially important when high ventilator rates are needed. After about 24 hours, the ventilator is adjusted to increase the PaCO2 by 1 to 2 torr. If the peak inspiratory pressure is greater than 45 cm water it should be reduced first. A blood gas is drawn to see if the Pao2 is stable and if the PaCO2 increased the desired small amount. If this first reduction in support is tolerated, it may be slowly followed by further reductions. Each step back from hyperventilation should be small so that the PaCO2 increases only by 1 to 2 mm Hg each time.
BOX 16-7 Common Mechanical Ventilator Parameters to Treat a Neonate with Persistent Pulmonary Hypertension
5. Inhaled nitric oxide
BOX 16-8 Common Mechanical Ventilator Parameters for Neonates with Bronchopulmonary Dysplasia
5. Monitor the mean airway pressure to evaluate the patient’s response to respiratory care (ELE Code: IIIE9) [Difficulty: ELE: R, Ap, An]
A number of ventilator control adjustments influence the patient’s mean airway pressure. (See Figure 16-11 for several examples of airway pressure tracings based on ventilator control changes.) If the patient’s compliance and resistance are stable, the Paw will be increased by the following: (1) an increased inspiratory flow, (2) an increased pressure limit (assuming the pressure limit has been previously reached), (3) an increase in PEEP, (4) an increased inspiratory time (assuming no change in ventilator rate and a decreased expiratory time), and (5) a decreased expiratory time (assuming an increased ventilator rate). These ventilator adjustments should result in an increased Pao2 and possibly a decreased PaCO2. Measure blood gases to be sure of the patient’s response.
If the patient’s compliance and resistance are stable, the Paw will be decreased by the following:
6. Ventilator alarm systems
7. Ventilator flow, volume, and pressure graphic waveforms
a. Select ventilator graphics e.g., waveforms, scales (Code: IIID3) [Difficulty: ELE: R, Ap; WRE: An]
b. Perform the procedure to measure ventilator graphics (e.g., pressure or volume) (WRE Code: IB9o) [Difficulty: WRE: R, Ap, An]
c. Interpret ventilator graphics e.g., pressure/volume (WRE Code: IB10o) [Difficulty: WRE: R, Ap, An]
Ventilator flow, volume, and pressure waveforms offer much clinically useful information. For example, expiratory flow can be monitored to look for air trapping and auto-PEEP. As discussed earlier, this is a concern if the neonate has obstructive airway disease or if a high respiratory rate is being used. Volume can be monitored as an indicator of improving or worsening lung compliance. Pressure can also be monitored as it relates to improving or worsening lung compliance. Less pressure is needed to deliver the desired tidal volume if the patient’s lung compliance is improving. Conversely, more pressure is needed to deliver the desired tidal volume if the patient’s lung compliance is worsening. Chapter 15 and this chapter include examples of waveform tracings.
d. Recommend changes in mechanical ventilation based on ventilator graphics (Code: IIIG3h) [Difficulty: ELE: R, Ap; WRE: An]
8. Prevent procedure-associated hypoxemia by oxygenating the patient before and after suctioning and equipment changes (ELE Code: IIID9) [Difficulty: ELE: R, Ap, An]
Chapter 13 discusses the suctioning procedure and steps that should be taken to prevent hypoxemia during suctioning. Older children can be given 100% oxygen during suctioning or equipment changes. Children younger than 6 months of age should have their oxygen percentage increased by 10% to 20% for the procedure.
MODULE C
1. Ventilators
As discussed in Chapter 15, the literature produced by the manufacturers and the descriptions used in many standard texts break the various ventilators into more categories than used by the NBRC. To avoid confusion, this text uses the NBRC’s more simplified terminology. A pneumatically powered ventilator is defined here as powered by compressed gas. It may be electrically controlled with electrical alarm systems. Fluidic ventilators are defined here as being pneumatically powered and partially or completely controlled by fluidic methods. Fluidic controls make use of compressed gas for cycling and other ventilator functions. Both of these types of neonatal ventilators use compressed air and oxygen that go to an air-oxygen mixer (blender) to determine the inspired oxygen. The gas then goes to a flow meter where the continuous flow per minute through the ventilator circuit is set. Depending on the ventilator, either compressed air or oxygen are used to drive the other control functions in fluidic ventilators.
a. Manipulate pneumatic ventilators by order or protocol (Code: IIA6a) [Difficulty: ELE: R, Ap; WRE: An]
b. Manipulate fluidic ventilators by order or protocol (Code: IIA6a) [Difficulty: ELE: R, Ap; WRE: An]
c. Manipulate electric ventilators by order or protocol (Code: IIA6a) [Difficulty: ELE: R, Ap; WRE: An]
d. Manipulate a microprocessor ventilator by order or protocol (Code: IIA6a) [Difficulty: ELE: R, Ap; WRE: An]
e. Manipulate high-frequency ventilators by order or protocol (WRE code: IIA6c) [Difficulty: WRE: R, Ap]
2. Put the equipment together and make sure that it works properly
The following are needed for proper assembly of a HFJV: (1) a pressurized oxygen source, (2) a pressurized air source, (3) an air-oxygen proportioner (blender), (4) a pressure regulator for controlling the patient’s peak pressure, (5) an injector line to add the jet volume to the endotracheal tube (Figure 15-20), and (6) an intravenous infusion pump to supply a steady drip of water to the jet ventilator injector line for nebulization.
2. Manipulate ventilator breathing circuits by order or protocol (ELE code: IIA11c) [Difficulty: ELE: R, Ap, An]
a. Get the necessary equipment for the procedure
Conventional neonatal ventilators use a circuit that has all of the standard features of an adult circuit as discussed in Chapter 15. Commonly, disposable single-patient-use corrugated plastic circuits are used. They are inexpensive and meet the needs of most patients. Condensation is drained out into water traps that are placed at low points in the natural draping of the inspiratory and expiratory limbs of the circuit. If excessive moisture is a problem or if the gas temperature must be maintained within a narrow range, a heated-wire circuit may be used. These types of circuits are built with a heated wire either loosely threaded through the lumen or coiled within the tubing itself. They are integrated with the humidification system and a servo unit for automatic temperature regulation. Because of the added wiring, these disposable circuits are considerably more expensive than the simple circuits used with most patients. A heat-moisture exchanger (HME) is usually only used with children because the tidal volume must be greater than the dead space of the HME. If a HME is used for passive humidification, it is added between the patient’s endotracheal tube and the Y of the ventilator circuit.’
When high flow rates and ventilating pressures are needed, as in HFV, a smooth bore and low compressible volume circuit is used instead of the standard disposable type. A special circuit made of noncompliant tubing is needed with the SensorMedics 3100A ventilator. In addition, to complete an HFJV circuit, a Hi-Lo Jet endotracheal tube or special endotracheal tube adapter is needed to deliver the jetted gas and add the entrained gas for the tidal volume. These are shown in Chapter 15.
3. Manipulate continuous positive airway pressure (CPAP) devices (Code: IIA2) [Difficulty: ELE: R, Ap; WRE: An]
a. Get the necessary equipment for the procedure
Nasal CPAP is associated with the hazards of gastric distension and reflux aspiration. These occur when the airway pressure forces air into the stomach. A gastric tube is usually inserted to vent the air out. There are two different devices for administering nasal CPAP. A nasopharyngeal tube is actually an endotracheal tube that has been cut shorter (see Figure 16-2, B). Select a tube that is the largest that can be easily inserted into the patient. Nasal prongs come in short and long versions (see Figure 16-2, A); both types involve prongs that fit into both nostrils. The prongs come in different diameters so that the proper size can be found to fit the internal diameter of the infant’s nares.
b. Put the equipment together and make sure that it works properly
Follow the manufacturer’s instructions for assembly of the CPAP device and CPAP circuit. See Figures 16-2 and 16-3 as well as the illustration of the CPAP circuit in Chapter 15 for general assembly guidance.
4. Manipulate a continuous positive airway pressure (CPAP) circuit by order or protocol (ELE code: IIA2) [Difficulty: Ap]
a. Get the necessary equipment for the procedure
The general discussion and an illustration related to CPAP systems was presented in Chapter 15. Freestanding neonatal systems are similar to those used with adults. All currently available neonatal ventilators offer a CPAP mode. This allows the practitioner to easily switch the intubated neonate from IMV to CPAP without the need to set up new equipment. Humidification options are similar to those for mechanical ventilation. A heated Cascade-type or wick-type humidifier can be used to provide moisture to the inhaled gas.
5. Manipulate humidification equipment by order or protocol (ELE code: IIA3) [Difficulty: ELE: R, Ap, An]
a. Get the necessary equipment for the procedure
The general discussion of humidification equipment was presented in Chapters 8 and 15. See the figures there and the general discussion for set-ups in ventilator and CPAP breathing circuits. Both heated humidifiers and heat-moisture exchangers are used with pediatric patients. Heated passover-type humidifiers systems are preferred with neonates.
c. Troubleshoot any problems with the equipment
Again, see Chapters 8 and 15 for assembly and troubleshooting of humidification systems. With a Cascade-type of passover-type humidifier, the gas temperature is usually maintained close to the neonate’s body temperature to maintain a neutral thermal environment. Make sure that the water level is kept in the recommended range to properly humidify the gas.
6. Perform quality control procedures for mechanical ventilators (Code: IIC5) [Difficulty: ELE: R, Ap; WRE: An]
Follow the manufacturer’s guidelines for quality control procedures on mechanical ventilators. The microprocessor ventilators usually have a software package that performs self-diagnostic tests on the unit. If a problem is found it is displayed on the monitor. Obviously the ventilator should deliver the volume, flow, and pressure that is set on the control. Do not use a ventilator that fails a quality control check.
MODULE D
1. Analyze the available information to determine the patient’s pathophysiologic state (Code: IIIH1) [Difficulty: ELE: R, Ap; WRE: An]
Refer to Chapter 1 or earlier in this chapter for a detailed discussion on neonatal assessment. A brief discussion of the most common neonatal pulmonary conditions requiring mechanical ventilation was presented earlier in this chapter.
2. Determine the appropriateness of the prescribed therapy and goals for the patient’s pathophysiologic state (Code: IIIH3) [Difficulty: ELE: R, Ap; WRE: An]
a. Recommend changes in the therapeutic plan when indicated (Code: IIIH4) [Difficulty: ELE: R, Ap; WRE: An]
b. Recommend sedation and/or use of muscle relaxant(s) (Code: IIIG1c) [Difficulty: ELE: R, Ap; WRE: An]
c. Recommend discontinuing treatment or procedure based on the patient’s response (Code: IIIG1i) [Difficulty: ELE: R, Ap; WRE: An]
3. Recommend the treatment of a pneumothorax (Code: IIIG1b) [Difficulty: ELE: R, Ap; WRE: An]
A chest radiograph and physical examination of the neonate will reveal if abnormal air or fluid is found around the lung(s) or heart. When transillumination of the chest is performed, any free air around the lung will be identified through the chest wall as a halo of light with a nonuniform shape. A nontension pneumothorax of greater than 10% is often also treated by inserting a pleural chest tube. If a patient has a tension pneumothorax, a pleural chest tube must be inserted to remove the air and relieve the pressure within the chest. A pleural chest tube is also placed to remove blood or other fluid from the pleural space. Chapter 18 has specific information that relates to treating a pneumothorax.
If a neonate is being ventilated on a constant volume ventilator, the amount of air that is lost through the pleural chest tube can be calculated. This is done by subtracting the measured exhaled volume from the measured inhaled volume. For example:
It is not possible to measure tidal volume when the neonate is ventilated by any other means. In a case like this, it is only possible to make a qualitative judgment on pleural air leak. In other words, if air is seen to bubble out through the pleural drainage system, an air leak is present. When the air stops bubbling, the pleural tear has healed. See Chapter 18 for a complete discussion on pleural drainage systems.
4. Weaning from the ventilator
b. Initiate and modify weaning procedures (Code: IIID7 and IIIF2i12) [Difficulty: ELE: R, Ap; WRE: An]
5. Extubation
b. Perform extubation (Code: IIIB9) [Difficulty: ELE: R, Ap; WRE: An]
The following additional steps are generally taken to ensure an appropriate, safe extubation:
AARC Clinical Practice Guideline: application of continuous positive airway pressure to neonates via nasal prongs or nasopharyngeal tube. Respir Care. 1994;39:817.
AARC Clinical Practice Guideline: application of continuous positive airway pressure to neonates via nasal prongs, nasopharyngeal tube, or nasal mask—2004 revision and update. Respir Care. 2004;49(9):1100.
AARC Clinical Practice Guideline: care of the circuit and its relation to ventilator-associated pneumonia. Respir Care. 2003;48:869.
AARC Clinical Practice Guideline: evidence-based guidelines for weaning and discontinuing ventilatory support. Respir Care. 2002;47:69.
AARC Clinical Practice Guideline: humidification during mechanical ventilation. Respir Care. 1992;37:887.
AARC Clinical Practice Guideline: neonatal time-triggered, pressure-limited, time-cycled, mechanical ventilation. Respir Care. 1994;39:808.
AARC Clinical Practice Guideline: patient-ventilator system checks. Respir Care. 1992;37:882.
AARC Clinical Practice Guideline: removal of the endotracheal tube—2007 revision & update. Respir Care. 2007;52:81.
AARC Clinical Practice Guideline: surfactant replacement therapy. Respir Care. 1994;39:824.
AARC Clinical Practice Guideline: ventilator circuit changes. Respir Care. 1994;39:797.
Aloan CA, Hill TV. Respiratory care of the newborn and child, ed 2. Philadelphia: JB Lippincott-Raven, 1997.
Avery ME, Tooley WH, Keller JB, et al. Is chronic lung disease in low birth weight infants preventable? A survey of eight centers. Pediatr. 1987;79:26.
Barnhart SL, Czervinske MP. Perinatal and pediatric respiratory care. Philadelphia: WB Saunders, 1995.
Betit P, Thompson J. Neonatal and pediatric respiratory care. In Wilkins RL, Stoller JK, Kacmarek RM, editors: Egan’s fundamentals of respiratory care, ed 9, St Louis: Mosby, 2009.
Boros SJ, Matalon SV, Ewald R, et al. The effect of independent variations in inspiratory-expiratory ratio and end expiratory pressure during mechanical ventilation in hyaline membrane disease: the significance of mean airway pressure. J Pediatr. 1977;91:794.
Branson RD, Hess DR, Chatburn RL. Respiratory care equipment, ed 2. Philadelphia: Lippincott Williams & Wilkins, 1999.
Burgess WR, Chernick V. Respiratory therapy in newborn infants and children, ed 2. New York: Thieme, 1986.
Cairo JM, Pilbeam SP. Mosby’s respiratory care equipment, ed 8. St Louis: Mosby, 2009.
Carlo WA, Martin RJ. Principles of neonatal assisted ventilation. Pediatr Clin North Am. 1986;33:221.
Cavanagh K. High frequency ventilation of infants: an analysis of the literature. Respir Care. 1990;35:815.
Chang DW. Clinical application of mechanical ventilation, ed 2. Albany, NY: Delmar, 2001.
Chatburn RL. High frequency ventilation: a report on a state of the art symposium. Respir Care. 1984;29:839.
Chatburn RL. Principles and practice of neonatal and pediatric mechanical ventilation. Respir Care. 1991;36:569.
Chatburn RL, Lough MD. Mechanical ventilation. In Lough MD, Doershuk CF, Stern RC, editors: Pediatric respiratory therapy, ed 3, St Louis: Mosby, 1985.
Chatburn RL, Waldemar AC, Lough MD. Clinical algorithm for pressure-limited ventilation of neonates with respiratory distress syndrome. Respir Care. 1983;28:1579.
Coghill CH, et al. Neonatal and pediatric high-frequency ventilation: principles and practice. Respir Care. 1991;36:596.
Gagnon C, Simoes J. The management of the mechanically ventilated infant receiving pancuronium bromide (Pavulon). Neonatal Network. Dec 1985:20-24.
Goldberg RN, Bancalari E. Bronchopulmonary dysplasia: clinical presentation and the role of mechanical ventilation. Respir Care. 1986;31:591.
Goldberg RN, Bancalari E. Therapeutic approaches to the infant with bronchopulmonary dysplasia. Respir Care. 1991;36:613.
Goldsmith JP, Karotkin EH. Assisted ventilation of the neonate, ed 2. Philadelphia: WB Saunders, 1996.
Hess DR, Kacmarek RM. Essentials of mechanical ventilation. New York: McGraw-Hill, 1996.
HIFI Study Group. High-frequency oscillatory ventilation compared with conventional mechanical ventilation in the treatment of respiratory failure in preterm infants. N Engl J Med. 1989;320:88.
Jobe A. Surfactant treatment for respiratory distress syndrome. Respir Care. 1986;31:467.
Koff PB, Eitzman DV, Neu J. Neonatal and pediatric respiratory care, ed 2. St Louis: Mosby, 1993.
MacIntyre NR, Branson RD. Mechanical ventilation, ed 2. Philadelphia: WB Saunders, 2009.
Milner AD, Hoskyns EW. High frequency positive pressure ventilation in neonates. Arch Dis Child. 1989;64:1.
Miyagawa CI. Sedation of the mechanically ventilated patient in the intensive care unit. Respir Care. 1987;32:792.
Nicks JJ, Becker MA. High-frequency ventilation of the newborn: past, present, and future. Respir Ther. 4(4), 1991.
Pilbeam SP, Cairo JM. Mechanical ventilation: physiological and clinical applications, ed 4. St Louis: Mosby, 2006.
Schmidt JM. Nitric oxide therapy in neonates: adding to the arsenal. Respir Ther. 1994;7:37.
Smith I. The impact of surfactant on neonatal intensive care unit management. Respir Ther. 1991;4(4):22-26.
Spear ML, Spitzer AR, Fox WW. Hyperventilation therapy for persistent pulmonary hypertension of the newborn. Neonatal Intensive Care: Perinatology/Neonatology. 1985;9:27.
Tobin MJ. What should the clinician do when a patient “fights the ventilator”? Respir Care. 1991;36:395.
Whitaker K. Comprehensive perinatal and pediatric respiratory care, ed 3. Albany, NY: Delmar, 2001.
White GC. Equipment theory for respiratory care, ed 4. Albany, NY: Delmar, 2005.
SELF-STUDY QUESTIONS FOR THE ENTRY LEVEL EXAM See page 601 for answers
STUDY QUESTIONS FOR THE WRITTEN REGISTRY EXAM See page 626 for answers