Chapter 27 Mechanical ventilation
Mechanical ventilation for acute respiratory failure (ARF) is now a routine aspect of patient management in the intensive care unit (ICU). The 1952 Copenhagen polio epidemic introduced the notion of organised areas (ICU) for the provision of positive-pressure ventilation,1 which was usually applied through a tracheostomy that had been inserted to allow suction of secretions. However, methods of ventilatory assistance without intubation had proliferated prior to the polio epidemic (both negative-pressure chest wall devices and positive-pressure face mask devices), and current trends are to an increased use of non-invasive ventilation (NIV) in patients with respiratory failure.2
A PHYSIOLOGICAL APPROACH
Inertial work is negligible, and usually ignored. Further, Equation (1) does not explicitly describe the elastic work required to initiate inspiration when intrinsic positive end-expiratory pressure (PEEPi) is present.
Because volume is constant in Equation (1), it can be simplified to:
MODES OF VENTILATION
CONTROLLED MECHANICAL VENTILATION
The simplest form of positive-pressure breath occurs in a relaxed subject, and the ventilator provides a constant gas flow during inspiration. The volume delivered will depend upon the inspiratory time (Ti), and Pao during inspiration will reflect Ers and Rrs (Figure 27.1). Expiration is a passive, and usually exponential, decline in volume to the relaxation volume of the respiratory system, equal to the functional residual capacity (FRC).
CMV is the most basic form of mechanical ventilation; however, it is an extremely useful baseline, and is still commonly used. A preset minute ventilation is made up from a fixed respiratory rate (f) and tidal volume (VT). Provided that there are not large variations in alveolar dead space, this maintains a preset alveolar ventilation (VA) and CO2 clearance. Consequently, CMV is useful in conditions where there is alveolar hypoventilation (e.g. respiratory muscle weakness), when PaCO2 needs to be maintained in a fixed range (e.g. raised intracranial pressure) or when the work of breathing must be minimised (e.g. severe cardiorespiratory failure). Because CMV may not match respiratory drive, and spontaneous, supported or assisted breaths are not possible during CMV, sedation, and sometimes muscle paralysis, may be needed. CMV is usually combined with PEEPe, which can recruit collapsed lung and reduce intrapulmonary shunt. The components are discussed below.
TIDAL VOLUME (vT)
Although traditional CMV VT has been 12–15 ml/kg, this may result in excessive lung stretch, particularly in patients with acute lung injury (ALI), leading to ventilator-induced (VILI) – also described as ventilator-associated – lung injury (VALI).3 The basis for this larger VT can be traced back to progressive atelectasis and intrapulmonary shunt, when physiologic VT was used during general anaesthesia. This could be reversed by larger VT ventilation or intermittent sigh breaths.4 In patients with ALI, VT of 6 ml/kg versus 12 ml/kg predicted body weight (i.e. often 4–5 ml/kg versus 9–10 ml/kg true weight) reduced mortality from 40% to 31%.5 Consequently, lower VT should be strongly considered during CMV, and other forms of ventilatory assistance in patients with ALI. However, greater levels of PEEP are usually required; similar data are not available for other respiratory diseases. Indeed, although the reduction in VT is particularly applicable to ALI, excessive lung stretch will be less likely in other patient cohorts that are able to ventilate a greater proportion of the lung (see Chapter 29).
RESPIRATORY RATE (f)
The desired minute ventilation can be selected from the product of VT and f. Common CMV rates are 10–20 breaths/min in adults. Sufficient expiratory time (Te) must be allowed to minimise dynamic hyperinflation and PEEPi. Although high f (up to 35 breaths/min) were allowed in the Acute Respiratory Distress Syndrome (ARDS) Network protocol, and the low-VT, low-mortality group had a mean f of ∼30 breaths/min,5 laboratory data suggest a possible additive role of high f in VILI.6
INSPIRATORY FLOW PATTERN
There are no convincing outcome data differentiating these different modes of CMV and PCV. Although the peak airway pressure (Ppk) is lower with PCV than constant-flow CMV, the alveolar distending pressure, which is usually inferred from the plateau pressure (Pplat), is no different provided that Ti and VT are the same.7 During PCV, Pres is dissipated during inspiration so Ppk and Pplat are equal, and during CMV, Pres accounts for the difference between Ppk and Pplat (Figure 27.2). Similarly, different CMVI patterns will alter Ppk without changing Pplat or mean airway pressure (Pmean) when Ti and VT are constant. In ARDS patients, comparing VCV and PCV, there is no difference in haemodynamics, oxygenation, recruited lung volume or distribution of regional ventilation;7,8 however, PCV may dissipate viscoelastic strain earlier.8 However, high may cause or exacerbate VILI,9 which may explain why some animal models have found PCV to be injurious compared to VCV, as PCV inherently has a high early
.
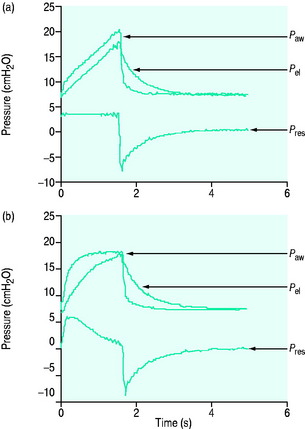
Figure 27.2 Actual pressure–time data from a patient with acute lung injury ventilated with volume-controlled ventilation (panel A), and then with pressure-controlled ventilation (panel B); tidal volume, I:E ratio and respiratory rate are constant. The airway pressure (Paw, bold line) has been broken down to its components, Pel and Pres (see Figure 27.1). Although there is no inspiratory pause, there is marked similarity between panel A and Figure 27.1, with the inspiratory difference between Paw and Pel due to a constant Pres. In panel B, the decelerating inspiratory flow pattern seen with pressure-controlled ventilation results in dissipation of Pres by end-inspiration. Consequently, during pressure-controlled ventilation Paw ≈ Pplat obtained during volume-controlled ventilation. In other words, for the same ventilator settings there is no difference in the elastic distending pressure.
Pressure-regulated volume control (PRVC) is a form of CMV where the VT is preset, and achieved at a minimum pressure using a decelerating flow pattern.
INSPIRATORY PAUSE
An end-inspiratory pause allows examination of the decay in Ppk, and measurement of Pplat. If Ti and V are maintained constant there is no improvement in oxygenation, and a more rapid will be needed. This may alter the distribution of ventilation. Theoretically, an end-inspiratory pause will allow a 32% dissipation of the total energy loss within the respiratory system,10 which may significantly reduce the forces driving expiration, and this could exacerbate gas trapping in patients with severe airflow limitation.
INSPIRATORY TIME, EXPIRATORY TIME, I:E RATIO
The combination of VT and inspiratory will determine Ti, which in combination with f sets Te. A typical Ti is 0.8–1.2 s; with a VT of 500 ml and inspiratory
of 0.5 l/s, the Ti is 1.0 s. During spontaneous ventilation, the distribution of ventilation at low
is determined by regional E, and at high inspiratory
by regional R, leading to greater ventilation of non-dependent lung. In patients with severe airflow limitation high inspiratory flow rates may be used in order to prolong Te and minimise dynamic hyperinflation.11
The I:E ratio is usually set at or below 1:2 to allow an adequate Te for passive expiration. During inverse-ratio ventilation (IRV), the I:E ratio is greater than 1:1. The putative benefits of a prolonged Ti include recruitment of long time-constant alveoli, and a short Te results in gas trapping and PEEPi. Early reports of clinical benefit did not control for PEEPi, and when total PEEP, f and VT are constant,7,8 pressure control (PC) IRV tends to reduce PaCO2 but does not improve oxygenation, may have deleterious haemodynamic effects and may exaggerate regional overinflation.8
POSITIVE END-EXPIRATORY PRESSURE
PEEP is an elevation in the end-expiratory pressure upon which all forms of mechanical ventilation may be imposed. When PEEP is maintained throughout the respiratory cycle in a spontaneously breathing subject, the term ‘constant positive airway pressure’ (CPAP) is used. The primary role of PEEP is to maintain recruitment of collapsed lung, increase FRC and minimise intrapulmonary shunt. PEEP may also improve oxygenation by redistributing lung water from the alveolus to the interstitium, and although there is no direct effect of PEEP to reduce extravascular lung water, this may occur in patients with left ventricular failure due to a reduction in venous return and left ventricular afterload. Further, inadequate PEEP may contribute to VILI by promoting tidal opening and closing of alveoli.3 PEEP levels of 5–15 cmH2O are commonly used, and levels up to 25 cmH2O may be required in patients with severe ARDS. Although a large multicentre study found no benefit of higher PEEP levels when VT was 6 ml/kg,12 individual titration may need to be considered.
PEEP titration in ARDS is complex (see Chapter 29), and should aim to improve oxygenation and minimise VILI. Since PEEP reduces venous return, cardiac output and O2 delivery may fall despite an improvement in PaO2; indeed, this concept has been used to optimise PEEP in ARF.13 However, in addition to recruitment, increasing PEEP may lead to overinflation of non-dependent alveoli which are already aerated at end-expiration.14,15 This will be less likely if alveolar distending pressure is kept < 30–35 cmH2O, or the change in driving pressure is < 2 cmH2O when VT is constant.16
PEEPe is applied by placing a resistance in the expiratory circuit (Figure 27.3), with most ventilators using a solenoid valve. Independent of the technique, a threshold resistor is preferred since it offers minimal resistance to flow once its opening P is reached. This will minimise expiratory work, and avoid barotrauma during coughing or straining.
SIGH
Many ventilators have the ability to deliver a breath intermittently at least twice VT. Sighs may reduce atelectasis, in part through release of pulmonary surfactant,17 resulting in recruitment and improved oxygenation in ARDS.18 However, if sighs or recruitment manoeuvres are used, care must be taken to avoid recurrent excessive lung stretch.
ASSIST-CONTROL VENTILATION (ACV)
During ACV, in addition to the set f, patient effort can trigger a standard CMV breath (Figure 27.4). This allows greater patient comfort; however, there may be little reduction in respiratory work compared to an unassisted breath at low , because the respiratory muscles continue to contract through much of the breath.19 The equivalent PCV breath is termed pressure assist-control ventilation (PACV). Differences between triggering modes will be discussed below, in the section on patient–ventilator interaction.
INTERMITTENT MANDATORY VENTILATION (IMV), SYNCHRONISED IMV (SIMV)
IMV was introduced over 20 years ago to aid weaning from CMV by allowing the patient to take unimpeded breaths while still receiving a background of controlled breaths. Proposed advantages include a reduction in sedation, lower mean intrathoracic pressure with less barotrauma and adverse haemodynamic consequences, improved intrapulmonary gas distribution, continued use of respiratory muscles and faster weaning. During SIMV Ti is partitioned into patient-initiated and true spontaneous breaths to avoid breath-stacking. However, during spontaneous breaths the work of breathing imposed by the endotracheal tube, circuit and ventilator must be overcome.20 In weaning studies comparing SIMV with T-piece trials and pressure support ventilation (PSV), SIMV is the slowest.21 Many clinicians add PSV during gradual reduction in respiratory rate with SIMV to overcome the added respiratory work imposed by the circuit and endotracheal tube; however, this approach has not been formally compared with other weaning techniques.
PRESSURE SUPPORT VENTILATION
During PSV, each patient-triggered breath is supported by gas flow to achieve a preset pressure, usually designated to be above the PEEPe. This can be explained by referring to the equation of motion where:
During PSV, Pao is the targeted variable by the ventilator, which leads to a significant and important reduction in Pmus and work of breathing.22