7 Mechanical Support of Cardiopulmonary Function
Extracorporeal Membrane Oxygenation, Ventricular Assist Devices, and the Intraaortic Balloon Pump
Pearls
• Signs of critically low cardiac output requiring mechanical circulatory support include: mixed venous oxygen saturation less than 40%, increased ventricular end-diastolic filling pressure, cardiac index less than 2 L/min per m2 body surface area (BSA), persistent metabolic acidosis, oliguria, poor peripheral perfusion, increased inspired oxygen requirements, and poor ventricular function by echocardiogram.
• Mechanical circulatory support (MCS) is indicated when low cardiac output persists despite maximal medical treatment, including afterload reduction combined with inotropes/catecholamines, diuretics, a phosphodiesterase-III inhibitor, fluid and transfusion management, and mechanical ventilation.
• In the pediatric population, ventricular assist devices (VADs) are used to support the failing heart as a “bridge to recovery” or “bridge to transplant.”
• MCS should be anticipated and every attempt should be made to initiate support before the development of end-organ dysfunction or circulatory collapse.
• Persistent low filling of a left ventricular assist device (LVAD) pump with rising right atrial pressure is an indication of right-sided heart failure. An echocardiogram is indicated to evaluate ventricular function and check for a pericardial effusion.
• The oxygen index (OI) is often used to identify possible indication for extracorporeal membrane oxygenation (ECMO) for children with respiratory failure.
• Alveolar-arterial (A-a) gradient of 600 to 624 mm Hg is a clinical indication for ECMO. This is computed as follows (NOTE: 47 mm Hg = partial pressure of water vapor):
• Signs to change venovenous (VV) ECMO to venoarterial (VA) ECMO include: persistent hypoxia in the presence of hypotension and poor cardiac output on maximal ventilator and inotropic support, nonperfusing arrhythmias, or cardiac arrest.
Introduction
The child with life-threatening cardiac or respiratory failure that is refractory to maximal medical support may require MCS. Over the last few years, substantial advances in pediatric MCS have occurred, with expanding indications for use, greater availability of devices suitable for pediatrics, and improved outcomes.16,20
ECMO and centrifugal ventricular assist devices remain the mainstay of MCS for children.23 MCS is not a treatment but rather a therapeutic support to provide adequate tissue oxygen delivery and maintain end-organ perfusion and function while the reversible disease responds to treatment. This support has many potential complications and should be used in appropriate patients with specific criteria for initiation.
Use of MCS in the pediatric population requires knowledge of the pathophysiology and treatment of cardiac and respiratory failure (see Chapters 6 to 9). In addition, the critical care nurse and the ECMO specialist require advanced training in MCS, because they must be familiar with the function of the support device, components of the circuit or console, potential complications, and troubleshooting for the device.
Device Selection
The selection of MCS device is influenced by the indication for the device, the duration of support anticipated, and the devices available. Devices are categorized for short- or long-term support. ECMO, centrifugal VADs, and intraaortic balloon pumps (IABPs) are considered short-term modalities. Pulsatile and rotary-axial devices are typically considered long-term modalities.11
Pediatric MCS offers a potential “bridge to transplant” or a “bridge to recovery.” The term destination therapy is used to describe long-term support for patients who are not heart transplant candidates and those whose heart function is unlikely to recover. Currently, no devices are approved by the U.S. Food and Drug Administration (FDA) for destination therapy in children. Three devices approved by the FDA for destination therapy in adults are the HeartMate VE/XVE LVAS and HeartMate II (Thoratec Corporation, Pleasanton, Calif.), and the AbioCor Implantable replacement heart (Abiomed, Danvers, Mass.).11,55
Extracorporeal membrane oxygenation
ECMO remains the most common form of MCS in the pediatric population. ECMO support evolved directly from cardiopulmonary bypass devices beginning with development of the membrane oxygenator in the 1950s.13,41 In the 1970s, intraoperative cardiopulmonary bypass was successfully used for infants during surgical correction of congenital heart disease.3 In 1972, successful ECMO support of a 24-year-old trauma victim with acute respiratory distress syndrome by Hill et al.37 led to a multicenter National Institutes of Health clinical trial of ECMO versus medical management of adult acute respiratory distress syndrome that failed to show a survival advantage with ECMO support, but did lead to widespread improvement in mechanical ventilation in adults.
In 1976, Bartlett et al.6 reported the first successful use of ECMO for treatment of severe neonatal respiratory distress. Since that time, ECMO has been used successfully in the treatment of neonates with meconium aspiration, congenital diaphragmatic hernia, pneumonia, sepsis, or persistent pulmonary hypertension.57 ECMO is now used as rescue therapy for pediatric patients with severe refractory respiratory or heart failure unresponsive to maximal conventional treatment.
In 1989, the ECMO centers formed a national organization, the Extracorporeal Life Support Organization to coordinate clinical research, develop ECMO guidelines, and maintain a national registry of all ECMO centers and cases. ECMO follow-up data have documented the cost effectiveness of ECMO compared with conventional support and improved patient outcomes with the use of ECMO.49
ECMO Terminology
The two types of ECMO support are VA and VV ECMO. VA ECMO provides both cardiac and respiratory support, whereas VV ECMO provides only pulmonary support. The differences between the VA and VV ECMO are summarized in Table 7-1.
VA ECMO | VV ECMO |
Achieves higher PaO2 | Achieves lower PaO2 |
Requires lower perfusion rates | Requires higher perfusion rates |
Bypasses pulmonary circulation | Maintains pulmonary blood flow |
Decreases pulmonary artery pressures | Elevates mixed venous PO2 |
Provides cardiac support to assist systemic circulation | Does not provide cardiac support to assist systemic circulation |
Requires arterial cannulation | Requires only venous cannulation |
ECMO, Extracorporeal membrane oxygenation; PaO2, partial pressure of arterial oxygenation; PO2, partial pressure of oxygen; VA, venoarterial; VV, venovenous.
Venoarterial ECMO
Circuit
The VA ECMO circuit is composed of polyvinyl chloride tubing attached to venous and arterial cannulas (Fig. 7-1). The venous cannula is inserted into the internal jugular vein and advanced through the superior vena cava and right atrium to the tricuspid valve. The arterial cannula is placed into the right common carotid artery with the tip of the cannula advanced to the innominate artery. Postoperative cannulation immediately after cardiac surgery is often accomplished through the median sternotomy incision with direct cannulation of the right atrial appendage and the aorta. In adolescent or adult patients, the femoral artery and vein are often used for VA ECMO cannulation.
Hemodynamic Changes During Venoarterial ECMO
ECMO Support with Single Ventricle Physiology
In patients with single ventricle physiology or shunt-dependent pulmonary blood flow, controversy exists about management of the shunt during MCS. There is debate regarding whether the shunt should be completely closed, partially closed, or left open during MCS. The challenge is to maintain balance between systemic and pulmonary circulation. If the shunt is completely closed during support, there is a risk of pulmonary infarction. If the shunt is open during ECMO, increased ECMO flow may be needed to maintain adequate pulmonary and systemic blood flow and systemic oxygen delivery.53
There are limited data regarding the role of ECMO after stage 1 palliation (and its variants) for hypoplastic left heart syndrome. ECMO is a useful tool for treatment of potentially reversible conditions, such as acute shunt thrombosis and transient depressed ventricular function53; ECMO may be used electively in the immediate postoperative period. However, the use of ECMO after the Norwood procedure remains controversial because the infant’s anatomy is complicated, the therapy is expensive, and survival rate is low.18,51,53,57
Venovenous ECMO
Hemodynamic Changes during Venovenous ECMO
Although there is no difference in the rate of survival, or rates of intracranial hemorrhage and infarction, seizures and brain death in pediatric respiratory patients for VV versus VA ECMO, VV ECMO is associated with significantly more cannula problems, higher incidence of CPR on ECMO, and use of inotropic support. In addition, there is also a trend toward more renal failure and use of hemofiltration on VV ECMO.62
Indications and Contraindications of ECMO Support
The indications and contraindications differ for neonatal, pediatric, and cardiac ECMO. Although specific inclusion and exclusion criteria exist, the use of ECMO remains a center specific decision and has to be considered on a case-by-case basis. The inclusion criteria for neonatal and pediatric ECMO are summarized in Box 7-1.
Box 7-1 Inclusion Criteria for Neonatal and Pediatric Respiratory ECMO Support
Pediatric
• Potentially reversible etiology for pulmonary failure
• Oxygenation index >40 and worsening respiratory failure despite maximal ventilator support
• Ventilator support for <14 days
• No other major contraindication to ECMO support such as severe central nervous system abnormality, ongoing hemorrhagic condition or coagulopathy, or multiple organ system failure in >3 organ systems
Neonatal Respiratory Failure
Contraindications for neonatal ECMO include the presence of irreversible and uncontrolled bleeding or coagulopathy, IVH greater than grade II, birth weight <2 kg, or gestational age <34 weeks, lethal chromosomal abnormality or congenital malformation incompatible with life, and duration of mechanical ventilation >14 days.43
With more extensive use of therapies such as high-frequency ventilation, surfactant, and nitric oxide, there has been a marked decline in the need for neonatal ECMO.38 If patients do not respond to these therapies, ECMO should be offered in a timely manner.
Pediatric Respiratory Failure
There is less consensus regarding indications for ECMO support for pediatric patients than for neonates with respiratory failure. Severe respiratory failure in children has many etiologies. A child is generally considered to be a candidate for ECMO if death is believed to be nearly certain despite maximal conventional therapy, and the lung disease is believed to be reversible and other organ systems are intact.29 In children, the OI has not been shown to be as predictive of mortality as it is in neonates. Many centers use the criteria of OI >40 and rising, hypercarbia and pH <7.1, a PaO2:FiO2 ratio less than 100 and falling, ventilator support for less than 14 days, and no contraindications such as significant neurologic or hemorrhagic conditions, or failure of more than three organ systems.43 With the use of lung protective strategies and adjunctive therapies such as high-frequency ventilation, surfactant, and nitric oxide, it has become harder to establish criteria for initiation of ECMO support. Contraindications for pediatric ECMO are similar to those in the neonatal period; these include diagnoses incompatible with life, intractable hemorrhage or coagulopathy, or severe central nervous system abnormality.
Cardiac Failure
Cardiac ECMO support has steadily increased over the past decade.24 Although isolated left ventricular failure is relatively rare in children, right ventricular failure, pulmonary hypertension, and hypoxemia are often associated with circulatory collapse in children with congenital heart disease. The most common causes of circulatory failure in infants and children are cardiovascular surgery (postcardiotomy), end-stage cardiomyopathy, and acute myocarditis. The most common indications for ECMO in cardiac patients are severe hypoxia, severe pulmonary artery hypertension, cardiogenic shock, cardiac arrest, and failure to wean from cardiopulmonary bypass after surgical repair.
Contraindications for the use of cardiac ECMO include incurable malignancy, advanced and presumed irreversible multisystem organ failure, extreme prematurity, and severe central nervous system abnormality or hemorrhage.59 In addition, if a patient will not be a transplant candidate, the patient should be carefully evaluated before ECMO. During the past 10 years, many contraindications have been removed from the list or labeled as relative rather than absolute contraindications.
Weaning from ECMO
There are two basic approaches to weaning patients from VA ECMO. Neither method has been shown to be superior to the other.32
Weaning VV ECMO is slightly different than weaning VA ECMO. When weaning VV ECMO, after the ventilator settings are increased, both of the membrane oxygenator gas ports are isolated from ambient air. Eventually, the blood entering and exiting the membrane oxygenator is in equilibrium and reflects typical venous values. This eliminates the need to clamp the venous cannula and allows a longer trial off VV ECMO.32
ECMO Troubleshooting
ECMO Circuit Emergencies
• Is blood ejecting from the circuit?
• Is air being pumped in the circuit?
• Is the pump delivering forward flow?
• Does the post-oxygenator blood appear red?
• Is the sweep gas being delivered and vented from the oxygenator?
If there is no squirting blood or air being pumped, the patient can be maintained on ECMO while the problem is corrected. ECMO circuit emergencies and problem troubleshooting are summarized in Table 7-2.
Problem | Signs and Symptoms | Response |
Clots in circuit | Dark zones or streaks seen; can cause bleeding due to coagulopathy (decreased platelets or fibrinogen unresponsive to transfusion) | Monitor circuit, monitor for coagulopathy, change circuit |
Oxygenator failure | Failure to remove CO2 or add adequate levels of oxygen in spite of increasing sweep gases and FiO2; may see blood or serum leaking from gas exhaust port | Remove air and debubble circuit if present; check that all gas lines are intact and not leaking to rule out gas line failure; replace oxygenator if it has failed |
Air in venous side of circuit | Bubbles seen | Correct source of problem, remove air |
Air in arterial side of circuit | Bubbles seen | Remove patient from ECMO by clamping arterial and venous lines to patient; place patient in Trendelenburg position; replace/repair the component identified as cause; remove all air, recirculate, and then return to ECMO |
Power failure | Pump stops with no AC power | Plug into emergency hospital power; use battery or UPS; hand crank |
Accidental decannulation | With partial removal on venous side, air entrainment can be seen; with complete decannulation, cannula will be out of the body with bleeding from cannulation site and possible pumping of air or blood | Cease ECMO and stop pump; put direct pressure on site; call surgeon for immediate replacement of cannula and to control bleeding; replace volume losses with available blood products and crystalloid |
Water heater failure | Patient exhibits hypothermia; bradycardia with reflex hypertension may be seen with pallor | Obtain new water heater and connect to circuit |
Raceway rupture | Blood spurts out of damaged tubing in pump head | Cease ECMO; turn pump off; replace raceway segment with new tubing; clean pump head; place new raceway segment into the pump head and recirculate; return to ECMO |
Cracks in tubing or connectors or loose stopcocks | On venous side [negative pressure side] air will entrain into the circuit. On positive pressure side of the circuit, blood will leak out | Tighten connections or replace cracked tubing or connectors; cease ECMO; stop pump; cut out and replace the damaged segments |
AC, Alternating current; ECMO, extracorporeal membrane oxygenation; UPS, universal power source.
Ventricular assist devices
The options for MCS for infants and children with cardiac failure are limited. There are currently no devices approved by the FDA for specific use in infant patients. However, several devices have been used successfully to support infants and children through the following: off-label use of devices approved by the FDA for use in adults, compassionate use of investigational adult VADs, the use of devices that have received an FDA Humanitarian Device Exemption, the use of devices fabricated from FDA-approved components (such as ECMO circuits), and devices that are approved on a case-by-case basis by the FDA for emergency use.7,8,12,20–22,27,28,31,44,56 Table 7-3 lists ventricular assist devices currently being used in the pediatric population under various exemptions or adult approved devices used in older children.2,26,30,35,42,45,46,61 Additional information regarding VADs used in children is included in Evolve Table 7-1 in the Chapter 7 Supplement on the Evolve Website.
Components and Function of VAD Support
A VAD is a heart pump that can be used to support the right ventricle (called a right ventricular assist device [RVAD]), the left ventricle (called an LVAD), or both ventricles (called a BiVAD). Most VADs have three major components: a pump (located inside or outside the body), a control system, and an energy source. The control system and energy source are found outside the body. The energy source can be a battery or compressed air (pneumatic).23
In the pediatric population, most VAD pumps are extracorporeal (outside the body) and connected to inflow and outflow cannulae (Fig. 7-2). The critical care nurse should understand the components of the VAD and VAD function.
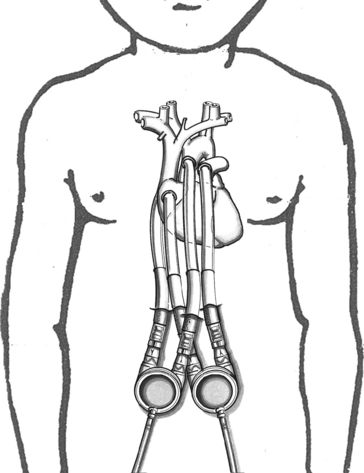
Fig. 7-2 Extracorporeal pneumatic biventricular assist device (Berlin Heart EXCOR).
(Redrawn from an illustration of the Berlin EXCOR by Berlin Heart, Berlin, Germany.)
The placement of the VAD cannula differs if the device is a bridge to recovery, compared with a bridge to transplantation. If the VAD support is serving as a bridge to recovery, the inflow cannula is often connected to the patient’s atrium.19 This cannulation is technically easier and spares the ventricle further injury. However, ventricular cannulation enables higher flow rates, so this form of cannulation is used when VAD support is used as a bridge to transplant, because ventricular injury is not a concern.
Ventricular Assist Device Flow and Function
Most VAD pumps used in children are either displacement pumps (pulsatile or pneumatic devices) or rotary pumps (continuous flow devices). The pulsatile or pneumatic pumps mimic the natural contraction (pumping action) of the heart. Flow rates depend on preload and the size of the external pump. Average flows for an infant-sized pump (12 or 15 mL) are 0.5 to 1.3 L/min and for a child-sized pump (25 or 30 mL) are 1.3 to 3.3 L/min.4 The external pump size can be changed to accommodate the child’s growth and increased stroke volume.
The most common continuous flow devices are axial or centrifugal pumps. Both types have a central rotor containing permanent magnets. Controlled electric currents that run through coils in the pump housing apply forces to the magnets causing the rotors to turn. Axial flow rates vary depending on the size of the device implanted. The child-sized device has been reported to provide an average flow of 0.3 to 2.5 L/min.4 The pediatric centrifugal Bio-Medicus Bio-pump (Medtronic, Inc. Minneapolis, MN) has both a 50- and 80-mL pump head size to accommodate infants <10 kg and children >10 kg, respectively. The 50-mL pump can provide flow up to 1.5 L/min and the 80-mL pump can provide >2 L/min.27
All VADs are preload dependent—the amount of blood returning to the heart is the amount of blood pumped to the body. The VAD is sensitive to impedance to flow, so hypertension and mechanical obstruction must be corrected. Both the LVAD and RVAD allow blood to bypass the failing ventricle. This decompresses that ventricle, decreases myocardial work, and reduces oxygen demand while maintaining adequate systemic perfusion to sustain end-organ function. VAD support has been shown to improve myocardial contractility. It also reverses beta receptor downregulation (documented to occur with heart failure), restoring myocardial response to the inotropic effects of adrenergic stimulation.48 VAD support can also normalize chamber geometry and reduce myocardial fibrosis, hypertrophy, and disruption in cytoskeletal proteins.10,34
Types of Ventricular Assist Device Pumps
Continuous Flow Pumps
Centrifugal Pump
The centrifugal pump is external, requires cannulation via a thoracotomy or sternotomy, and can be used for single or biventricular support. The most common centrifugal pump is the Bio-Medicus Bio-Pump (Medtronic, Inc. Minneapolis, Minn). The Bio-Pump uses two magnetically coupled, polycarbonate rotator cones that spin to create centrifugal force along a vertical axis.11 The rotors are shaped to accelerate the blood circumferentially and thus cause it to move toward the outer rim of the pump. The constrained vortex pump design creates subatmospheric pressure at the tip of the cone, establishing suction in the venous cannula.40 Blood enters at the apex of the cone and is ejected tangentially at the base of the cone (Fig. 7-3). The cone design retains any small air bubbles.
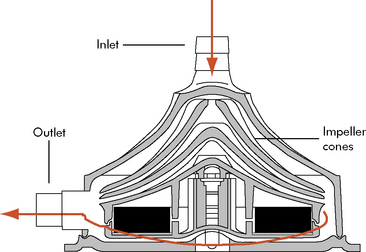
Fig. 7-3 Bio-Medicus centrifugal pump.
(From Karl TR, Horton SB, and Brizard C: Postoperative support with the centrifugal pump ventricular assist device (VAD). Seminars in Thoracic and Cardiovascular Surgery: Pediatric Cardiac Surgery Annual 9:83–91, 2006.)
The pump output is proportional to revolutions per minute and is adjusted according to the venous return. Spins averaging 10,000 to 20,000 rpm will create a blood flow of 5 to 6 L/min in larger pumpheads.36 This type of pump can support neonates and older children with postoperative cardiac failure but competent lung function.36,58
The centrifugal VADs include Bio-Medicus Bio-pump, Levitronix CentriMag (Levotronix, Waltham, Mass.), RotaFlow (Jostra, Hirrlingen, Germany), and the Capiox Terumo (Terumo Cardiovascular Systems, Ann Arbor, Mich.). The Levitronix CentriMag is marketed in Europe, but available in the United Stated only as an investigational device.11 The advantage to this device is that it can be attached to cardiopulmonary bypass cannula already in place. However, cannula adaptors may be needed for smaller patients.
Axial Flow Pump
Axial flow pumps reflect recent industry efforts to develop smaller, lighter, quieter, implantable pediatric VADs. Axial flow pumps are designed to assist the left ventricle as a bridge to transplant or destination therapy. The pump has a magnetically levitated impeller that rotates in a cylindrical chamber so that blood accelerates toward the rotor’s axis, usually 7 to 11.5 cm in length, with spins of 7500 to 12,000 rpm (Fig. 7-4). A flexible motor cable supplies power to the implanted pump. The axial flow pumps have been used successfully in 5 to 16 year olds with BSA ≥0.7 m2.
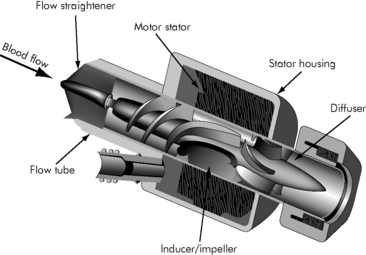
Fig. 7-4 Axial continuous flow pump
(Redrawn from an illustration of the Axial Flow Pump, Micromed DeBakey VAD, by Micromed Technology, Houston, Texas.)
Advantages of the axial pumps are small size, ease of implantation and explantation, low rates of infection, and minimal formation of thrombus.16 The disadvantages are nonpulsatile flow, limited sizes for children, and need for ventricular apical cannulation.16
The MicroMed DeBakey VAD/MicroMed DeBakey VAD Child (MicroMed Cardiovascular, Houston, Texas), HeartMate II LVAS (Thoratec, Pleasanton, Calif.), and Jarvik 2000 (Jarvik Heart, New York, N.Y.) are currently used axial flow VADs for adults and selected children with a BSA >0.7 m2. An infant (3-15 kg) and child (15-25 kg) Jarvik 2000 are being developed through the National Heart, Lung and Blood Institute (NHLBI) Circulatory Assist Program.4
Pulsatile Pumps
Pulsatile VADs contain a reservoir. Blood is ejected by the pump either electronically through the movement of pusher plates or with compressed air movement of the bladder. These devices propel blood in synchrony with the patient’s ventricular ejection, producing pulsatile arterial blood flow. These devices are paracorporeal systems and consist of a pneumatic compressor-operated diaphragm pump with inflow and outflow valves. A transparent polyurethane pump housing allows inspection for potential thrombus development. The external pump position enables fast and safe pump changes if required.54
The Berlin Heart Excor Pediatric VAD (Berlin Heart, Berlin, Germany), Medos HIA-VAD (Medos Medizintechnik, Aachen, Germany) and the Thoratec VAD (Thoratec) provide both single and biventricular support. The Berlin Heart and Medos HIA-VAD are available in the United States under compassionate use appeal to the FDA. The Thoratec device can only be used in patients with BSA >0.8 m2.11
Indications and Contraindications for Ventricular Assist Device Support
The clinical indications for pediatric VAD support are severe ventricular failure, shocklike state, or the progression of multiorgan failure resulting from acute fulminant myocarditis, cardiomyopathy, postcardiotomy failure, posttransplantation graft failure, and end-stage congenital heart disease.19 Signs of critically low cardiac output or ventricular failure include: mixed venous oxygen saturation (SvO2) <40%, increased ventricular end-diastolic filling pressure, cardiac index <2 L/min per m2 BSA, persistent metabolic acidosis, oliguria, poor peripheral perfusion, increasing FiO2 requirement, signs of beginning renal and hepatic failure, and significantly impaired ventricular function by echocardiogram (Box 7-2). VAD support is indicated when all medical treatment options have been exhausted, including afterload reduction combined with catecholamines, diuretics, a phosphodiesterase-III inhibitor, fluid and transfusion management, and mechanical ventilation. In addition, any residual correctable contributing lesion should be ruled out in patients with congenital heart disease.
Patients are evaluated on an individual basis for VAD support. The overall decision for VAD support should be made earlier rather than later to improve chances for end-organ recovery and survival. Contraindications to VAD support include extreme prematurity, irreversible multisystem organ failure, incurable malignancy, and severe central nervous system damage.20
Weaning From VAD Support
Before weaning a pulsatile pneumatic device, improvement in systolic myocardial function is verified by transthoracic echocardiogram or cardiac catheterization. Weaning protocols including the need for additional anticoagulation are device-specific and should be reviewed before initiating the weaning process. The device is removed in the operating suite, with or without the use of temporary cardiopulmonary bypass support. Support can be converted to ECMO support or to a centrifugal VAD pump with later weaning as described previously.1
Termination or withdrawal of VAD support may be indicated if myocardial function does not recover. Withdrawal of support requires careful communication with family members (see Chapters 2 and 3).
Complications of Ventricular Assist Device Support
Potential complications of VAD support include: bleeding requiring reoperation, embolism (clot or air), hemolysis, infection, and mechanical failure.19 Bleeding and embolism are the most common complications following VAD insertion. Mild to moderate bleeding is common and is most often caused by anticoagulation, a coagulopathy, or surgical bleeding. Excessive bleeding requiring massive transfusions often results in pulmonary and multiorgan dysfunction and can be fatal.
Troubleshooting Ventricular Assist Device Support
Troubleshooting VAD support requires knowledge of the type of device (continuous flow or pulsatile), type of support (single or biventricular support), and make of device. Common problems and potential causes are listed in Table 7-4 and are addressed under Nursing Care.
Problem | Potential Cause |
Low LA pressure |
ASD, Atrial septal defect; DIC, disseminated intravascular coagulation; HIT, heparin-induced thrombocytopenia; LA, left atrium; LVAD, left ventricular assist device; PFO, patent foramen ovale; RA, right atrium; RV, right ventricle.
(From Reddy M and Hanley FL. Mechanical support of the myocardium. In Chang AC et al., editors. Pediatric cardiac intensive care. Baltimore, 1998, Williams and Wilkins, pp. 345–349.)
The paracorporeal pneumatic VADs with small pump volumes, designed specifically for infants and children, have been used in Europe since 1992. The Berlin Heart Excor VAD is an extracorporeal device made in a wide range of pump sizes from 10 to 80 mL; it can provide medium- to long-term circulatory support for pediatric patients ranging from 2.5-kg infants to adolescents (see Fig. 7-2).
In 2002, the NHLBI recognized the limitations of circulatory support devices in small children. The NHLBI awarded contracts to five research institutes to develop and evaluate circulatory assist devices for children.4 Information about the devices and program locations is available in Evolve Table 7-1 in the Chapter 7 Supplement on the Evolve Website.
Intraaortic balloon pump
IABP counterpulsation is frequently used in adults for managing acute left ventricular dysfunction after myocardial infarction or cardiac surgery.50 The first reported use of an IABP in pediatrics was in 1980.52 Support for the failing pediatric myocardium has primarily focused on the use of ECMO and VAD. Despite the availability of pediatric-sized catheters, the use of IABP in infants and children is not widespread, with use at a limited number of centers.14,39,50 The use of IABP in infants and children remains limited for a variety of reasons, including technical difficulty inserting the catheters in the infant or small child, the limited availability of smaller volume catheters, the greater distensibility of the pediatric vasculature, and the difficulty in balloon timing with rapid heart rates in pediatric patients.
Components and Function
The IABP augments cardiac output by inflating during diastole and by deflating immediately before systole. As the balloon inflates, it displaces a volume equal to the balloon volume, producing augmentation of the diastolic pressure and increased coronary artery perfusion. Counterpulsation requires precise balloon inflation at the onset of diastole and aortic valve closure, to augment diastolic and coronary flow, and rapid balloon deflation at the onset of systole and aortic valve opening so that it produces a fall in left ventricular impedance/afterload as the left ventricle begins to eject (Fig. 7-5).
Indications and Contraindications
Indications and contraindications for IABP use have been well established for adults. However, balloon insertion is more invasive in children and requires more consideration and preparation. IABP use is most common in children with left ventricular dysfunction after cardiac surgery or associated with myocarditis, cardiomyopathy, Kawasaki disease, persistent ventricular arrhythmias, or severe sepsis.33
Timing of Inflation and Deflation
The IABP uses helium or carbon dioxide to rapidly inflate and deflate the balloon. The balloon inflates at the onset of cardiac diastole and deflates at the onset of systole. The standard method of balloon inflation timing is by triggering the R wave from the ECG while monitoring the arterial waveform pressure. The inflation and deflation points are adjusted to maximize afterload reduction and diastolic augmentation. It is helpful to record an arterial waveform tracing during a 1:2 heart rate-to-counterpulsation ratio, comparing the assisted and unassisted pulse to assess the effectiveness of the timing of counterpulsation.50 Balloon inflation and deflation can also be timed using M-mode echocardiography.50 The transducer is positioned parasternal to obtain simultaneous images of both the aortic valve and the balloon. During imaging, the balloon inflation and deflation points are adjusted to coincide with aortic valve closure and opening, respectively.
Complications
Complications reported during pediatric IABP therapy include limb ischemia, infection, balloon rupture, vessel perforation, thrombocytopenia, aortic dissection, and mesenteric, renal, or cerebral ischemia.33,50
Nursing care of the pediatric patient requiring MCS
The nursing care plan in Box 7-3 is designed for pediatric patients on ECMO and VAD support. Interventions are separated if they differ for ECMO or VAD support.
Box 7-3 Nursing Care of the Pediatric Patient During Mechanical Circulatory Support
Potential for Hemorrhage and other Complications Related to MCS Malfunction
• ECMO device failure/disruption can result from raceway rupture, inadvertent decannulation, power failure
• VAD device failure/disruption can result from console malfunction or power failure
Nursing Interventions
• Frequently inspect circuits for cracks or stress on the raceway and make planned repairs or replacements.
• Frequently inspect and test alarms and pressure monitors.
• Assess electrical outlets and connections on a routine basis (per hospital protocol).
• Keep emergency resuscitation medications at bedside.
• Keep appropriate emergency equipment (e.g., metal tubing clamps, hand crank, bulb syringes) available
• Have backup ECMO circuit/pump and VAD console available and charged.
Potential Inadquate Cardiac Output Related to
• Tamponade or pericardial effusion
• Myocardial dysfunction (cardiac stun)
Nursing Interventions
• Assess child’s systemic perfusion, including temperature, color of mucous membranes, nail beds, skin, quality of peripheral pulses, and capillary refill time.
• Notify provider of signs of poor systemic perfusion.
• Monitor for evidence of bleeding: excessive chest tube output (>3 mL/kg per hour), oozing from cannulation site and sternal patch (if closure delayed).
• Monitor for signs of coagulopathies.

• Monitor for signs of cardiac tamponade in patients receiving MCS:


• Measure and record hourly urine output; report output <0.5 mL/kg per hour.
• Monitor heart rate and rhythm; ensure that heart rate is appropriate for the patient’s age and condition. Report clinically significant arrhythmias to the provider.
• Monitor patient’s arterial blood pressure; report hypotension or hypertension.
• Monitor cardiac index; report decrease and any cardiac index <2.5 L/min per m2 BSA.
• Monitor arterial blood gases and serum lactate; report acidosis or abnormalities.
• During ECMO, obtain simultaneous arterial and SvO2 measurements. If difference is increasing, the child’s cardiac output is probably falling; if difference is decreasing, the child’s cardiac output probably is rising. Adjust ECMO flow per protocol or order.
• Monitor patient for normal MAP (per age). If SVR or MAP is elevated:


Potential for Inadequate Intravascular Volume Related to
Expected Outcome
• Patient demonstrates adequate intravascular volume with adequate ECMO/VAD filling
• Hematocrit within normal limits per age and orders or protocol
• Adequate CVP or RA pressure and pulmonary capillary wedge pressure (PCWP) or left atrial (LA) pressure
• Urine output 0.5-2.0 mL/kg per hour
• Chest tube output <3 mL/kg per hour
• Adequate systemic perfusion, moist mucous membranes, and good skin turgor
Nursing Intervention
• Record and calculate total fluid intake and output, including blood drawn for laboratory analysis.
• Measure CVP/RA and PCWP/LA pressures and support to optimize perfusion per orders.
• Draw blood sample for Hct immediately after ECMO/VAD insertion and then per orders. If Hct is low, administer packed red blood cells (PRBCs) per order.
• Draw blood samples for coagulation studies (ACT, PLT, PT, aPTT) immediately after ECMO/VAD insertion and then per orders. Adjust heparin infusion per standing orders for VAD and ECMO device. If platelet count is low, replace with platelets per orders. Keep ACT between 140 and 180 sec for VAD devices and between 200 and 240 sec for ECMO.
• Milk chest tubes gently and tap firmly to keep free of clots. Notify the provider if chest tube output is >3 mL/kg per hour for 3 h or 5 mL/kg per hour for 1 h.
• For patients with delayed sternal closure, notify the provider if the sternal patch fills or bulges.
• Discuss with a provider the possibility of surgical bleeding in the presence of excessive chest tube output and the absence of coagulopathy.
• Assess patient’s hydration and report signs of inadequate hydration. Signs of adequate hydration are
• Avoid any nonessential invasive procedures (e.g., peripheral IV removal) while the patient is receiving heparin therapy, because the procedure may cause uncontrolled bleeding at site.
Potential Impairment in Gas Exchange Related to
Expected Outcomes
• Patient will demonstrate adequate gas exchange as evidenced by satisfactory clinical appearance and arterial blood gases, appropriate pulse oximetry and exhaled carbon dioxide, and normal lung compliance with full lung expansion and no evidence of atelectasis.
• For the patient requiring VAD/ECMO support, SvO2 of 70-75% and PaO2 of 50-70 mmHg will be maintained.
Nursing Interventions
• Monitor for signs of hypoxemia, including tachycardia, increased spontaneous respiratory rate, compromise in systemic perfusion, deterioration in level of consciousness, decreased hemoglobin saturation, rise in exhaled CO2, deterioration in PaO2 or pH.
• Long-term VAD use should be weaned to extubation once the patient’s condition is stable, with regular pulmonary toilet incorporated into daily routine.
• Draw arterial, venous, and pump (for ECMO) blood gases immediately after ECMO/VAD insertion and then per orders. Venous and arterial pH, PaO2, and PaCO2 should be within normal limits.
• Adjust sweep gas and ECMO flow per protocol or MD order. Increasing the sweep gas decreases PaCO2 levels, and increasing the ECMO flow rate elevates the PaO2 levels.
• Auscultate lung sounds and monitor chest expansion.
• Verify appropriate endotracheal tube depth of insertion or adjust if needed.
• Monitor for and notify provider of any change in PIP, PEEP, and exhaled tidal volume.

• Suction via endotracheal tube per unit protocol and as needed. Monitor color, consistency and quantity of secretions.
• Change the patient’s body position every 1-2 h as tolerated.
Potential Alteration in Cerebral Perfusion and Function Related to
Nursing Interventions
• Assess neurologic function before and immediately after initiation of MCS.
• Correct hypotension, hypoxemia, and acidosis as soon as possible.
• Monitor NIRS cerebral tissue oxygenation (normal range >50%). Notify provider of decrease.
• Verify performance and report results of cranial ultrasound examinations daily for first 3 days of ECMO (or per protocol).
• Maintain ACT in desired range for VAD (140-180 sec) or ECMO (200-240 sec)
• Monitor ECMO/VAD circuit and tubing for clot or air formation; notify provider if present.
• Check coagulation studies and administer anticoagulation therapy as indicated for MCS.
Potential Alteration in Renal Function and Urine Output Related to
Nursing Intervention
• Measure urine output every hour and notify provider if hourly total is < 0.5 mL/kg per hour

• If urine is red or rust color, provide intravenous fluids to maintain urine flow and prevent RBC accumulation in glomeruli causing acute tubular necrosis or renal failure.
• If hyperkalemia develops during VAD, administer calcium, glucose, and insulin, or sodium polystyrene sulfonate (Kayexalate) enema per orders (see Chapter 12 and Table 12-4).
• Prepare for peritoneal dialysis, hemodialysis, or modified hemofiltration (depending on circulatory support) per orders as indicated (see Chapter 13).
• If renal impairment is suspected, closely monitor serum creatinine, BUN, and K+ concentrations.
• If renal impairment is present, evaluate doses of drugs that require renal excretion.
Potential Infection Related to
Nursing Interventions
• Ensure that every provider washes hands before and after patient contact.
• Ensure appropriate sterile and aseptic techniques for procedures or dressing changes.
• Maintain occlusive dressings over all invasive monitoring catheters and an open chest.
• Change dressings and intravenous tubing per unit policy.
• Monitor patient for signs and symptoms of infection.
• Administer antibiotics as ordered.
• If infection is present, monitor for evidence of sepsis (tachycardia, blood pressure instability, thrombocytopenia, temperature instability).
Potential Child and Family Knowledge Deficit Related to
• Child’s cardiovascular disease
• Initiation and requirement of mechanical circulatory support device
Nursing Interventions
• Ascertain what the patient and family have been told about the disease, therapy, and prognosis.
• Ask parents how they think child can best be prepared for MCS (as appropriate).
• Assess and document the child’s and the family’s learning needs and barriers to learning.
• Prepare the child and family for clinical course of mechanical circulatory support including:
• Encourage the child and the family to ask questions and participate in care when appropriate.
• Record specific teaching information (including terminology used) so that the healthcare team can use consistent terms and reinforce identical information.
Potential Child and Family Anxiety Related to
Nursing Interventions
• Maintain open communication with patient and family.
• Answer all questions honestly at the appropriate level for patient and family understanding.
• Prepare patient (as age appropriate) and family for procedures. Include a child life specialist as appropriate for the child’s age and clinical condition.
• Encourage family participation in patient care as appropriate.
• Encourage the child’s expression of feelings and emphasize acceptability of expression.
• Assess the family’s resources and refer to appropriate hospital support services (See Chapter 2)
Pediatric Critical Care Unit (PCCU) Setup
The PCCU bedside setup for MCS is the same for a postoperative cardiac surgery patient (see Chapter 8). The bed selection, bed location, and equipment needed (IV pumps, ventilator) should be prearranged, if possible, to optimize space for easy movement during care of the patient. Although equipment for MCS can vary depending on the selected device, all extension cords and drive lines must be well secured to minimize accidental falls or disconnection.
Admission or Initiation of MCS and Staffing
ECMO support can be initiated in the operating suite, or cannulation can be performed at the bedside. Although there are many variations in staffing, in most ECMO centers two providers are assigned to the patient: a critical care nurse provides all patient care and an ECMO specialist monitors and cares for the ECMO circuit. ECMO specialists are specially trained nurses, respiratory care practitioners, or perfusionists who have attended an ECMO training class. The ELSO published guidelines for the training of these specialists, including detailed knowledge of the pathophysiology of common diseases, ECMO physiology, and immediate treatment for patient events and ECMO emergencies. (For more information, see http://www.elso.med.umich.edu/)
Postoperative Care
Near-Infrared Spectroscopy (NIRS)
The cerebral rSO2 value will vary depending on the level of sedation or agitation and the location of the sensor. It will also be affected by factors influencing cerebral blood flow such as increased intracranial pressure (can decrease blood flow), changes in PaCO2 (hypocarbia produces cerebral vasoconstriction and decreased cerebral blood flow, and hypercarbia produces cerebral vasodilation and increased blood flow) and severe hypoxemia (produces cerebral artery dilation and attempts to increase cerebral blood flow). The target cerebral rSO2 is greater than 50%.25
The somatic tissue oxygenation should be higher than cerebral tissue oxygenation, because the kidneys receive 25% of cardiac output at any time. The target difference between somatic and cerebral rSO2 should be greater than 10%, with somatic readings approximately 10% to 15% points higher than cerebral readings.25
NIRS monitoring can be a valuable tool during ECMO support to identify a compromise in tissue oxygenation that may result from reversible problems with cannula placement, and it can assist in the evaluation of systemic oxygen delivery and readiness for decannulation. If a change in cannula position or a circuit malfunction occurs, the flow deficit will produce a fall in rSO2. Furthermore, if the rSO2 falls significantly during weaning and clamping of the ECMO circuit, then additional time on ECMO will be needed. If the rSO2 remains stable in a patient with other signs of adequate systemic perfusion, the patient is likely to be sufficiently stable for decannulation.25
Sedation and Analgesia
Neuromuscular blockade may be needed for the hemodynamically unstable patient receiving MCS. Pancuronium (0.1 mg/kg per dose) or a Cis-atracurium infusion (0.2 mg/kg per hour) can be used. Minimal use of neuromuscular blockade is advantageous to allow spontaneous movement and assess for seizure activity. For further information, see Chapter 5.
Respiratory Assessment
Potential respiratory complications include pneumothorax or hemothorax, pleural effusions, and pulmonary hemorrhage. Needle aspiration or chest tube insertion are options for emergent decompression of a pneumothorax or hemothorax or drainage of a pleural effusion. If pulmonary hemorrhage develops, management consists of minimizing the target ACT, increasing the platelet count, and minimizing suctioning. If bleeding becomes more severe, epinephrine (0.1 mL/kg of 10,000 dilution) may be instilled into the trachea and positive end expiratory pressure instituted. See Chapter 9 for information regarding mechanical ventilation.
Cardiac Assessment and Support
Inappropriate Vascular Resistance
Elevated systemic vascular resistance can result from hypervolemia or from the initiation of MCS. Nonpulsatile device flow leads to the release of renin, which stimulates the renin-angiotensin system, causing arteriolar constriction.47 Hypertension can cause bleeding in the patient receiving anticoagulation. Vigilant blood pressure control is essential to reduce the incidence of IVH. In addition, the nurse must assess for and treat agitation and pain as potential causes of hypertension.
Native Myocardial Dysfunction
During VA ECMO, the pump provides nonpulsatile flow, so systemic perfusion will improve if the native myocardium ejects some blood.60 Left ventricular ejection facilitates decompression of the left heart, reducing the need for left atrial venting, and may potentially reduce the risk of intracardiac thrombosis formation from blood stasis. It is challenging to identify the optimal pump flow rate to optimize cardiac output in the presence of right ventricular failure caused by hypoxia and pulmonary hypertension. Before weaning of ECMO support, the native myocardium may require inotropic support.
Troubleshooting VAD Pump and Console
As more VADs are placed in children as a bridge to transplant, longer support durations are inevitable. Consequently, VAD dysfunction may become more prevalent. The VAD-trained critical care nurse should examine all external device components such as cannulas, drive lines, battery support, and all mechanical and electrical connections. The nurse should be familiar with the VAD alarms and troubleshooting techniques (see Table 7-4).
Hematologic Assessment
Thrombosis and bleeding are the most common complications of MCS.60 Patients receiving MCS are susceptible to bleeding from all surgical sites and should be monitored closely (e.g., cannula sites, incisions, chest tubes). All saturated dressings should be measured and included in the total output calculation. All bodily fluids such as stool, urine, gastric drainage, and sputum should be checked for blood. The nurse should monitor the child’s abdominal girth and assess for increased abdominal distension and firmness. In addition, the nurse should try to avoid any procedure that may produce bleeding such as heel sticks, venipunctures or injections, insertion of a nasogastric tube, or urinary catheter or rectal probe insertion.
The patient’s hematocrit, fibrinogen, and platelet counts are routinely monitored, and appropriate blood products are administered as needed. Blood product replacement protocols may vary among institutions. In general, the target platelet count is >100,000/mm3, fibrinogen 100 mg/dL, and hematocrit >35%. If possible, blood transfusions should be limited for patients awaiting transplant, because frequent exposure can increase the panel reactive antibody, which can complicate matching for a future transplantation.11 Blood products should be irradiated, depleted of leukocytes, and negative for cytomegalovirus.11 If excessive nonsurgical bleeding is encountered, aminocaproic acid, aprotinin, and recombinant factor VII may be administered.
Anticoagulation
Heparin-Induced Thrombocytopenia (HIT)
A potential problem with the use of heparin is HIT. HIT occurs in approximately 5% of patients who receive heparin.15 HIT is an immune response with an immune complex (called heparin-platelet factor IV complex) formed between administered heparin and platelet factor IV on the surfaces of platelets. The immune system responds to this complex as a foreign substance and forms antibodies, causing platelet aggregation, platelet damage, and ultimately thrombotic complications and thrombocytopenia. Testing for HIT is done by checking the platelet count and serologic or functional assays for heparin-platelet factor 4 (PF 4) antibodies.
The treatment for HIT is removal of all sources of heparin. Because most patients receiving MCS will require anticoagulation, a direct thrombin inhibitor such as argatroban or lepirudin can be used in place of heparin.5
Infection
Patients receiving MCS support are at high risk for nosocomial infection secondary to surgical incisions or open chest, cannulae sites, invasive monitoring catheters, drainage tubes, mechanical ventilation, and compromised nutrition and immune status. Early indicators of infection may absent or difficult to detect during MCS. Thrombocytopenia results from platelet destruction by the ECMO circuit or VAD pump. In addition, core body temperature can be affected by the ECMO heat exchanger.47 The white blood cell count with differential should be obtained daily, and routine blood, urine, and endotracheal tube cultures should be obtained to monitor for infection. Patients with unexplained hemodynamic instability, coagulopathy, elevated white blood cell count, or fever should be promptly treated for suspected sepsis pending culture results (see Chapter 6).60
Prophylactic broad-spectrum intravenous antibiotics are administered before mechanical support and are continued after insertion. Cannulation through an open sternum poses a greater risk for mediastinitis. Therefore, in patients with an open sternum, antibiotic coverage is broadened to include a second- or third-generation cephalosporin in combination with a course of vancomycin or oxacillin.9,60
Psychological Support
Parents often face overwhelming feelings of anxiety, fear, and helplessness, yet can remain hopeful for successful therapy.17 Age-appropriate explanations of components and functions can gradually introduce the device to the patient and family. The nurse also provides crucial emotional support during the recovery period.
Commonly used drugs
The most commonly used drugs during MCS are ones that optimize cardiac and pulmonary function, such as inotropes, antihypertensive or afterload reducing agents, and pulmonary vasodilators. A balance between hemostasis and anticoagulation is required after initiation of MCS. Multiple antifibrinolytics and anticoagulants can be used depending on postinsertion bleeding, development of coagulopathies, and device-specific coagulation to prevent thrombus formation. Other commonly used drugs during MCS include analgesics, sedatives, antibiotics, diuretics, electrolyte replacement, and resuscitation drugs (in case of mechanical failure and emergent removal from ECMO). Table 7-5 includes a list of commonly used drugs with doses and cautions. See Chapter 6 for further description of resuscitation.
Drug | Dose | Caution |
Anticoagulation* | ||
Heparin sodium | IV infusion: 20 units/kg per hour | May cause thrombocytopenia or HIT; hemorrhage is the most common complication; monitor platelet count, PTT, hematocrit, and ACT |
Adjust dose by 2-4 units/kg per hour every 4-8 h as required | ||
Aspirin | For Blalock-Taussig (subclavian to pulmonary artery) shunts: 3-5 mg/kg per day up to 40-81 mg/day | At high serum concentrations, may cause GI intolerance, respiratory alkalosis, and pulmonary edema |
For prosthetic heart valves: 6-20 mg/kg per day | ||
Aminocaproic acid (Amicar) | IV infusion: 33.3 mg/kg per hour | May cause hypotension, bradycardia, and arrhythmias |
Argroban | Initial dose: 2 mcg/kg per minute | May be used for anticoagulation in patients diagnosed with HIT; may cause hypotension, ventricular tachycardia, and bradycardia |
Measure aPTT after 2 h; adjust dose until aPTT is 1.5-3 times baseline | ||
Recombinant activated factor VII (rFVIIa; NovoSeven) | 90 mcg/kg q2 h until hemostasis is achieved or judged ineffective | May cause hypertension or hemorrhage; monitor for signs/symptoms of thrombosis |
Warfarin sodium (Coumadin) | Maintenance dose: 0.05-0.34 mg/kg per day | For long-term use in stable patient with a VAD; monitor INR or PT and signs/symptoms of hemorrhage |
Titrate based on INR | ||
Aprotinin (Trasylol) | 40 mL/m2 per hour | May cause renal impairment, heart attack, and stroke |
Inotropes† | ||
Dopamine (Inotropin) | 2-20 mcg/kg per minute (titrate to desired effect) | May produce extreme tachycardia or result in increased pulmonary artery pressure |
Dobutamine (Dobutrex) | 2-20 mcg/kg per minute (titrate to desired effect) | May produce extreme tachycardia and pulmonary vasoconstriction; patients with atrial fibrillation or flutter are at risk of developing a rapid ventricular response |
Epinephrine (Adrenalin) | 0.2-0.3 mcg/kg per minute | May produce tachycardia, hypertension, cardiac arrhythmias, increased myocardial oxygen consumption, decreased renal, and splenic blood flow |
Norepinephrine | 0.05-1 mcg/kg per minute | May produce arrhythmias, bradycardia, tachycardia, hypertension, or organ ischemia caused by vasoconstriction of renal and mesenteric arteries |
Vasopressin (Pitressin) | 0.2 to 2 milliunits/kg per minute (0.0002-0.002 units/kg per minute | Can produce venous thrombosis, vasoconstriction, bradycardia, heart block, and distal limb ischemia |
Phosphodiesterase-III inhibitor‡ | ||
Milrinone (Primacor) | Loading dose of 0.05 mg/kg (50 mcg/kg) slowly (over 10-60 min). Infusion dose: 0.25-0.75 mcg/kg per minute | Can produce ventricular and supraventricular arrhythmias and bronchospasm |
Vasodilators/Antihypertensives§ | ||
Nitropresside (Nipride) | IV: 0.3-8 mcg/kg per minute | Can produce excessive hypotensive response, tachycardia, and elevated serum creatinine |
Enalapril (Vasotec) | PO: 0.1-0.5 mg/kg per day in 1-2 doses | Can produce hypotension, angioedema, anaphylactic reactions, neutropenia, proteinuria, and hepatic failure |
IV: 5-10 mcg/kg per dose q8-24 h | ||
Captopril (Capoten) | PO: 0.1-0.5 mg/kg per dose q8-24 h | Can produce hypotension hepatic failure, anaphylactoid reactions, agranulocytosis, proteinuria |
Maximum: 6 mg/kg per day | ||
Esmolol (Brevibloc) | IV: 75-150 mcg/kg per minute | May cause bradycardia, hypotension, peripheral ischemia |
Titrate dose by 50 mcg/kg per min q10 min | ||
Maximum dose: 1000 mcg/kg per minute | ||
Propranolol (Inderal) | PO: 0.5 mg/kg per day | May cause hypotension and bradycardia. |
IV: 0.15-0.25 mg/kg | ||
Pulmonary Vasodilators|| | ||
Inhaled Nitric Oxide (INOmax) | In the ventilated patient: 5-20 ppm | Abrupt discontinuation may lead to worsening hypotension, oxygenation, and increasing pulmonary artery pressure; doses >20 ppm increase the risk of methemoglobinemia and elevated nitrogen dioxide levels |
Sildenafil (Viagra) | PO: 0.25-2 mg/kg per dose q4-8 h | May cause hypotension, tachycardia, ventricular arrhythmia, cerebrovascular hemorrhage, pulmonary hemorrhage, sudden hearing loss |
Diuretics¶ | ||
Furosemide (Lasix) | PO, IV: 1-2 mg/kg per dose q4-12 h | May cause hypokalemia and hyponatremia necessitating electrolyte monitoring |
IV continuous infusion: 0.1-0.4 mg/kg per hour | ||
Chlorothiazide (Diuril) | PO: 10-40 mg/kg per day divided in 2 doses | May cause hypokalemia, hyperglycemia, and hyperuricemia |
IV: 2-8 mg/kg per day divided into 1-2 doses | ||
Spironolactone (Aldactone) | PO: 1-3.5 mg/kg per day; may be given as single dose or total dose can be divided into 2 or 4 doses (given twice a day or every 6 h) | Potassium-sparing diuretic; may cause hyponatremia, hyperkalemia, and dehydration |
Bumetanide (Bumex) | PO: 0.04-0.8 mg/kg per day; dose is divided and given every 6-8 h | Can have profound diuresis with fluid and electrolyte loss; close supervision and dose evaluation are required. |
Electrolyte Replacement# | ||
Potassium chloride | Normal requirement: 2-3 mEq/kg per day | May cause cardiac arrhythmias, heart block, hypotension, and bradycardia; patients have a cardiac monitor during intermittent infusions |
Prevention of hypokalemia: 1-2 mEq/kg per day above maintenance | ||
IV intermittent infusion for severe depletion: 0.5-1 mEq/kg per dose, typically over 1-3 hours | ||
Calcium chloride | IV: 10-20 mg/kg per dose | May cause vasodilation, hypotension, bradycardia, and cardiac arrhythmias; avoid extravasation |
Repeat q4-6 h if needed | ||
Magnesium sulfate | PO: 100-200 mg/kg per dose q6 h | May cause hypotension, circulatory collapse, depression of cardiac function, and heart block |
IV: 25-50 mg/kg per dose q4-6 h |
ACT, Activated clotting time; aPTT, activated partial thromboplastin time; GI, gastrointestinal; HIT, heparin-induced thrombocytopenia; INR, international normalized ratio; IV, intravenous; PO, by mouth; PT, prothrombin time; PTT, partial prothrombin time; VAD, ventricular assist device.
* Primary concern related to blood-surface interaction with artificial materials, increasing the risk of clot in the mechanical circulatory support device.
† Indicated for poor systemic perfusion and myocardial function with appropriate oxygenation, ventilation, heart rate, and intravascular volume.
‡ Inotropic, chronotropic, and vasodilatory effects.
§ Dilate arteries and veins to reduce preload, reduce systemic vascular resistance, and improve ventricular compliance, thus improving cardiac output.
|| To aid in oxygenation and decrease right ventricular afterload.
¶ For treatment of oliguria and hypervolemia associated with poor systemic perfusion and CHF as the result of low cardiac output.
# Electrolyte imbalances alter myocardial transmembrane potential, depolarization, and repolarization, thus affecting the excitability of myocardial tissue and conduction of electrical impulses. To ensure optimal myocardial function and cardiac output, electrolytes must be closely monitored and replaced as necessary.
MCS for specific diseases
The Chapter 7 Supplement on the Evolve Website provides detailed information about common diseases requiring MCS support. Many of the specifics can be reviewed in more depth in Chapters 6 to 9. It is important to remember that the specific diseases discussed are at end-stage and require MCS for survival.
Diagnostic tests during MCS
The following diagnostic tests are the most commonly used to assess cardiac and respiratory function and to evaluate for possible postoperative MCS complications. Refer to Chapters 8 to 10 for more detailed descriptions of the tests.
1 Alexi-Meskishvili V., et al. The use of the Berlin heart in children. In: Duncan B.W., editor. Mechanical support for cardiac and respiratory failure in pediatric patients. New York: Marcel Dekker, 2001.
2 Ashton R.C.Jr, et al. Left ventricular assist device options in pediatric patients. ASAIO J. 1995;41:M277-M280.
3 Baffes T.G., et al. Extracorporeal circulation for support of palliative cardiac surgery in infants. Ann Thorac Surg. 1970;10:354-363.
4 Baldwin J.T., et al. The national heart, lung, and blood institute pediatric circulatory support program. Circulation. 2006;113:147-155.
5 Baroletti S.A., Goldhaber S.Z. Heparin-induced thrombocytopenia. Circulation. 2006;114:355-356.
6 Bartlett R.H., et al. Extracorporeal membrane oxygenation (ECMO) cardiopulmonary support in infancy. Trans Am Soc Artif Intern Organs. 1976;22:80-93.
7 Boneva R.S., et al. Mortality associated with congenital heart defects in the United States: trends and racial disparities, 1979-1997. Circulation. 2001;103:2376-2381.
8 Boucek M.M., et al. Registry for the International Society for Heart and Lung Transplantation: seventh official pediatric report: 2004. J Heart Lung Transplant. 2004;23:933-947.
9 Brown K.L., et al. Healthcare-associated infection in pediatric patients on extracorporeal life support: the role of multidisciplinary surveillance. Pediatr Crit Care Med. 2006;7:546-550.
10 Bruckner B.A., et al. Regression of fibrosis and hypertrophy in failing myocardium following mechanical circulatory support. J Heart Lung Transplant. 2001;20:457.
11 Carberry K.E., et al. Mechanical circulatory support for the pediatric patient. Crit Care Nurs Q. 2007;30(2):121-142.
12 Chen Y.S., et al. Experience and result of extracorporeal membrane oxygenation in treating fulminant myocarditis with shock: what mechanical support should be considered first? J Heart Lung Transplant. 2005;24:81-87.
13 Clowes G.H., Hopkins A.L., Neville W.E. An artificial lung dependent upon diffusion of oxygen and carbon dioxide through plastic membranes. J Thorac Cardiovasc Surg. 1956;32:630-637.
14 Collison P.S., Dager S.K. The role of the Intra-aortic balloon pump in supporting children with acute cardiac failure. Postgrad Med J. 2007;83:308-311.
15 Comunale M.E., Vancott E.M. Heparin induced thrombocytopenia. Int Anesthesiol Clin. 2004;42(3):27-43.
16 Cooper D.S., et al. Cardiac extracorporeal life support: state of the art in 2007. Cardiol Young. 2007;17(Suppl. 2):104-115.
17 Curley M.A., Meyer E.C. Parental experience of highly technical therapy: survivors and nonsurvivors of extracorporeal membrane oxygenation support. Pediatr Crit Care Med. 2003;4(2):214-219.
18 De Oliveira N.C., et al. Prevention of sudden circulatory collapse after the Norwood operation. Circulation. 2004;110:II133-II138.
19 Dickerson H.A., Chang A.C. Perioperative management of ventricular assist devices in children and adolescents. Semin Thorac Cardiovasc Surg Pediatr Card Surg Annu. 2006;9:128-139.
20 Duncan B.W., et al. Mechanical circulatory support for the treatment of children with acute fulminant myocarditis. J Thorac Cardiovasc Surg. 2001;122:440-448.
21 Duncan B.W. Mechanical circulatory support for infants and children with cardiac disease. Ann Thorac Surg. 2002;73:1670-1677.
22 Duncan B.W. Mechanical circulatory support in infants and children with cardiac disease. In Zwischenberger J.B., Bartlett R.H., editors: ECMO Extracorporeal Cardiopulmonary Support in Critical Care, ed 2, Ann Arbor, MI: Extracorporeal Life Support Organization, 2000.
23 Duncan B.W. Pediatric mechanical circulatory support in the United States: past, present, and future. ASAIO J. 2006;52:525-529.
24 : ECMO Registry of the Extracorporeal Life Support Organization (ELSO). January 2004 Ann Arbor, Michigan
25 Fenton K.N., et al. The significance of baseline cerebral oxygen saturation in children undergoing congenital heart surgery. Am J Surg. 2005;190(2):260-263.
26 Frazier O.H., et al. Research and development of an implantable axial-flow left ventricular assist device: the Jarvik 2000 Heart. Ann Thorac Surg. 2001;71:S125-S132.
27 Fuchs A., Netz H. Ventricular assist devices in pediatrics. Images Paediatr Cardiol. 2002;9:24-54.
28 Goldman A.P., et al. The waiting game: bridging to paediatric heart transplantation. Lancet. 2003;362:1967-1970.
29 Goodman D.M., Green T.P. Extracorporeal life support for children with acute respiratory distress syndrome. In: Zwischenberger J.B., Steinhorn R.H., Bartlett R.H., editors. ECMO: extracorporeal cardiopulmonary support in critical care. Ann Arbor, MI: Extracorporeal Life Support Organization; 2000:451-460.
30 Griffith B.P., et al. HeartMate II left ventricular assist system: from concept to first clinical use. Ann Thorac Surg. 2001;71:S116-S120.
31 Grinda J.M., et al. Fulminant myocarditis in adults and children: bi-ventricular assist device for recovery. Eur J Cardiothorac Surg. 2004;26:1169-1173.
32 Hansell D.R. Extracorporeal membrane oxygenation for perinatal and pediatric patients. Respir Care. 2003;48(4):352-362.
33 Hawkin J.A., Minich L.L. Intraaortic balloon counterpulsation for children with cardiac disease. In: Duncan B.W., editor. Mechanical support for cardiac and respiratory failure in pediatric patients. New York: Marcel Dekker, 2001.
34 Heerdt P.M., et al. Chronic unloading by left ventricular assist device reverses contractile dysfunction and alters gene expression in end-stage heart failure. Circulation. 2000;102:2713.
35 Helman D.N., et al. Implantable left ventricular assist devices can successfully bridge adolescent patients to transplant. J Heart Lung Transplant. 2000;19:121-126.
36 Hetzer R., Stiller B. Ventricular assist device for children. Nat Clin Pract Cardiovasc Med. 2006;3(7):377-386.
37 Hill J.D., et al. Prolonged extracorporeal oxygenation for acute post-traumatic respiratory failure (shock-lung syndrome). N Engl J Med. 1972;286:629-634.
38 Hintz S.R., et al. Decreased use of neonatal extracorporeal membrane oxygenation (ECMO): how new treatment modalities have affected ECMO utilization. Pediatrics. 2000;106(6):1339-1343.
39 Kalavrouziotis G., Karunaratne A., Raja S. Intraaortic balloon pumping in children undergoing cardiac surgery: an update on the Liverpool experience. J Thorac Cardiovasc Surg. 2006;131:1382-1389.
40 Karl T.R., Horton S.B. Centrifugal pump ventricular assist device in pediatric cardiac surgery. In: Duncan B.W., editor. Mechanical support for cardiac and respiratory failure in pediatric patients. New York: Marcel Dekker, 2001.
41 Kolobow T., Bowman R.L. Construction and evaluation of an alveolar membrane artificial heart-lung. Trans Am Soc Artif Intern Organs. 1963;9:238-243.
42 Korfer R., et al. Single-center experience with the Thoratec ventricular assist device. J Thorac Cardiovasc Surg. 2000;119:596-600.
43 Lequier L. Extracorporeal life support in pediatric and neonatal critical care: a review. J Intensive Care Med. 2004;19:243-258.
44 Lipshultz S.E., et al. The incidence of pediatric cardiomyopathy in two regions of the United States. N Engl J Med. 2003;348:1647-1655.
45 McBride L.R., et al. Clinical experience with 111 Thoratec ventricular assist devices. Ann Thorac Surg. 1999;67:1233-1239.
46 Morales D.L., et al. Lessons learned from the first application of the DeBakey VAD Child: an intracorporeal ventricular assist device for children. J Heart Lung Transplant. 2005;24:331-337.
47 Moynihan P.J., et al. Nursing management of children with cardiac disease on mechanical circulatory support. In: Duncan B.W., editor. Mechanical support for cardiac and respiratory failure in pediatric patients. New York: Marcel Dekker, 2001.
48 Ogletree-Hughes M.L., et al. Mechanical unloading restores beta-adrenergic responsiveness and reverses receptor down regulation in the failing human heart. Circulation. 2001;104:881.
49 Petrou S., et al. Cost-effectiveness of neonatal extracorporeal membrane oxygenation based on 7-year results from the United Kingdom Collaborative ECMO Trial. Pediatrics. 2006;117(5):1640-1649.
50 Pinkney K.A., et al. Current results with intraaortic balloon pumping in infants and children. Ann Thorac Surg. 2002;73:887-891.
51 Pizarro C., et al. Is there a role for extracorporeal life support after stage I Norwood? Eur J Cardiothorac Surg. 2001;19:294-301.
52 Pollock J., Charlton M.C., Williams W.G. Intraaortic balloon pumping in children. Ann Thorac Surg. 1980;29:522-528.
53 Ravishankar C., et al. Extracorporeal membrane oxygenation after staged I reconstruction for hypoplastic left heart syndrome. Pediatr Crit Care Med. 2006;7:319-323.
54 Schmid C., et al. Pediatric assist with Medos and Excor systems in small children. ASAIO J. 2006;52:505-508.
55 Slaughter MS, et al. Advanced heart failure treated with continuous-flow left ventricular assist device. N Engl J Med. 2009;361:2241-2251.
56 Throckmorton A.L., et al. Pediatric circulatory support systems. ASAIO J. 2002;48:216-221.
57 UK Collaborative ECMO Trial Group. UK collaborative randomised trial of neonatal extracorporeal membrane oxygenation. Lancet. 1996;348:75-82.
58 Ungerleider R.M., et al. Routine mechanical ventricular assist following the Norwood procedure—improved neurologic outcome and excellent hospital survival. Ann Thorac Surg. 2004;77:18-22.
59 Van Meurs K.P., et al, editors. ECMO: extracorporeal cardiopulmonary support in critical care, ed 3, Ann Arbor, MI: Extracorporeal Life Support Organization, 2005.
60 Wessel D.L., Almodovar M.C., Laussen P.C. Intensive care management of cardiac patients on extracorporeal membrane oxygenation. In: Duncan B.W., editor. Mechanical support for cardiac and respiratory failure in pediatric patients. New York: Marcel Dekker, 2001.
61 Wieselthaler G.M., et al. First clinical experience with the DeBakey VAD continuous-axial-flow pump for bridge to transplantation. Circulation. 2000;101:356-359.
62 Zahraa J.N., et al. Venovenous versus venoarterial extracorporeal life support for pediatric respiratory failure: are there differences in survival and acute complications? Crit Care Med. 2000;28(2):521-525.