CHAPTER 91 Management of Spasticity by Central Nervous System Infusion Techniques
Chronic intrathecal infusion of baclofen has been extremely successful in the treatment of spasticity.1,2 Because baclofen does not cure spasticity, most treated patients need to take baclofen intrathecally for the rest of their lives. This long-term commitment to treatment is similar to maintaining shunt function in hydrocephalics and may be equally rewarding or frustrating. To make proper use of this powerful tool, neurosurgeons need to understand the pharmacology and distribution of intrathecal baclofen and how pumps are used to infuse the drug. Proper selection of patients requires an understanding of the physiology of spasticity and its clinical manifestations. Other neurosurgical methods for reducing spasticity are considered elsewhere; in particular, dorsal rhizotomy for spastic cerebral palsy in children is discussed in Chapter 90.
Definition of Spasticity
Spasticity is a term that refers to a wide variety of motor problems and has numerous associated definitions.3 It has been used to connote difficulty with coordinated movements, spasms, rigidity, abnormal primitive reflexes, and hyperactive reflexes. Researchers studying spasticity tend to stress definitions that emphasize abnormal reflex responses, whereas clinicians tend to stress more global definitions, primarily related to impairment of movement. To further complicate the issue, many clinical syndromes associated with spasticity are caused by injuries at multiple sites in the neuraxis, and the pathologic mechanisms producing motor dysfunction are equally varied.
For the sake of clarity, a narrow physiologic definition is used in this chapter, with note made when more general meanings arise in clinical situations. According to the definition of Lance, spasticity is “a motor disorder characterized by a velocity-dependent increase in tonic stretch reflexes (i.e., muscle tone) with exaggerated tendon jerks, resulting from hyperexcitability of the stretch reflex, as one component of the upper motoneuron syndrome.”4 The important point to stress about this definition is that spasticity is a velocity-dependent phenomenon. This dependence on velocity differentiates spasticity from other, non–velocity-dependent forms of rigidity that can be caused by contractures, dystonias, or Parkinson’s disease. In addition to hyperactive reflexes, spasticity can be associated with various symptoms that can be viewed in terms of positive and negative categories.5 Positive signs are produced by overactivity (i.e., disinhibition) of certain pathways as a result of injury to a specific part of the motor system; negative symptoms are caused by lack of function of the injured area. In spasticity, an increase in deep tendon reflexes and resistance to passive stretch of the limb are positive signs. Negative symptoms are weakness and loss of dexterity. It is the negative symptoms that usually create the clinically significant problems that are disabling for the patient. Occasionally, increased muscle tone, clonus, and hyperactive reflexes can interfere with initiation and smooth completion of a movement.6 Spasms, although often associated with hyperactive reflexes, are not a necessary concomitant of hyperreflexia and should be considered separately.
The relationship between physiologic abnormalities and motor disability is a major area of investigation and of more than academic interest. It is uncertain how or to what extent the velocity-dependent increase in stretch reflex activity interferes with movement.7 Therapies that reduce reflex excitability may not necessarily improve the symptoms of spasticity.3,8,9 To evaluate and treat patients with spasticity, the physician must have a basic understanding of its pathophysiology and knowledge about scales of measurement and different types of treatment.
Physiologic Basis of Spasticity
The final common pathway to the muscle is the alpha motoneuron. Many mechanisms influence the output of the alpha motoneuron and may therefore exaggerate this neuron’s response to stretch. The muscles contain receptors called spindles, which are diagrammed in the center portion of Figure 91-1. A spindle is made up of intrafusal fibers attached to primary sensory endings. The primary sensory endings, which are extensions of the large, myelinated Ia afferents, attach at the noncontractile equatorial region of the intrafusal fibers. The spindle organs are attached at both ends of the muscle mass and consist of extrafusal fibers. Because the spindles are attached in this way, they undergo the same changes in length as the overall muscle and monitor changes in muscle length. The spindles themselves are under control of the fusimotor efferents (i.e., gamma system).
When a muscle is stretched, it contracts in an attempt to regain its original length. This response can be broken down into five events, four of which are diagrammed in Figure 91-1:
Mechanisms Underlying Reflex Function
To understand how changes in these mechanisms lead to spasticity, we must trace the events that occur when a muscle is stretched. In a closed loop system, it is impossible to identify the beginning and end of a sequence of events. For simplicity of presentation, the various mechanisms are discussed in the following order: Ia monosynaptic connection, Ia excitatory polysynaptic pathways, reciprocal Ia inhibition, group II pathways, decreased recurrent inhibition, alpha motoneuron hyperexcitability, gamma motoneuron hyperactivity, and group Ib inhibition. A detailed discussion of these mechanisms has been presented by Pierrot-Deseilligny,11,12 Pierrot-Deseilligny and Mazieres,13 and Sehgal and McGuire.14 Sehgal and McGuire also provided a detailed explanation of the different electrophysiologic testing procedures that are used to deduce which mechanisms are underlying the spasticity.14
Ia Monosynaptic Connection
When a muscle is stretched, the Ia afferent neuron transmits excitatory messages from its receptor, the muscle spindle, to the alpha motoneuron of the same muscle by means of a monosynaptic connection. It has been suggested that Ia discharge is normally reduced by presynaptic inhibition and that reduced levels of presynaptic inhibition could lead to an increased stretch reflex.13 For example, Calancie and colleagues argued that presynaptic inhibition is enhanced in patients in the acute stage of spinal cord injury but is reduced in the chronic stage.15 This reduction in presynaptic inhibition could give rise to an increased reflex response. There is also evidence of a decrease in presynaptic inhibition in paraplegic patients but not in hemiplegic patients.16
Ia Excitatory Polysynaptic Pathways
The alpha motoneuron can also be affected by the Ia excitatory polysynaptic pathway (Fig. 91-2).17 This can be shown by producing vibration-induced activity in group Ia fibers to generate tonic contraction of the vibrated muscle through Ia excitatory polysynaptic pathways, the tonic vibration reflex. This reflex can be increased or decreased, or both, in spastic patients.18 Facilitation of this pathway leads to increased stretch reflexes.
Reciprocal Ia Inhibition
When a muscle is stretched, the reflex evoked in that muscle is normally accompanied by inhibition of the opposing muscle. This finding of reciprocal inhibition has had a pervasive influence on our understanding of movement control.19 It has been assumed that as a movement is made, stretch-related activation in the antagonist muscle is suppressed by reciprocal inhibition. Lack of reciprocal inhibition can lead to unwanted activation of the antagonist muscle and impede movement. The Ia inhibitory interneuron receives excitatory synaptic input from numerous descending pathways, including the corticospinal tract.20 Input from the descending tracts is combined with output from the Ia afferent of the contracting agonist muscle.21 If the Ia interneuron does not receive input from the corticospinal tract, reciprocal inhibition may become ineffective, a phenomenon observed in some spastic patients.22
Decreased Recurrent Inhibition
Motor axons give off recurrent collaterals that activate Renshaw cells, which in turn inhibit alpha motoneurons,11 thereby creating a recurrent inhibitory circuit. Renshaw cells are influenced by supraspinal control, which can facilitate or inhibit them. At rest, a complicated picture emerges with respect to recurrent inhibition.23 In about 40% of spastic patients tested, there is no evidence of abnormal recurrent inhibition at rest. In patients with hemiplegia (most often from stroke) and in patients with spinal cord injury (most often from trauma), recurrent inhibition increases. In contrast, patients with progressive paraparesis caused by hereditary spastic paraparesis or amyotrophic lateral sclerosis exhibit reduced recurrent inhibition.
During active movements in spastic patients, an increased reflex response may be caused by lack of inhibition of Renshaw cells. In healthy subjects, Renshaw cells are inhibited, thereby inhibiting the Ia inhibitory interneuron directed to the antagonist motoneuron. This prevents decreased inhibition of the Ia inhibitory interneuron by Renshaw cells and allows reciprocal inhibition to function to suppress a stretch reflex in the antagonist muscles.24 Lack of descending control of Renshaw cells leads to impaired voluntary movements and prevents modulation of control of the antagonist muscle, as has been shown by Katz and Pierrot-Deseilligny.25
Alpha Motoneuron Hyperexcitability
The alpha motoneuron receives excitatory input from the segmental and descending pathways. Lesions at numerous levels of the central nervous system may upset the delicate balance between excitatory and inhibitory input influencing the alpha motoneuron. There may also be a change in the intrinsic properties of motoneurons in spasticity.12 However, the influence of alpha hyperexcitability is impossible to assess in humans because it requires knowledge of the firing level of motoneurons deprived of any sensory input.11 There is no evidence for or against the involvement of alpha hyperexcitability in spasticity, although Noth has argued that it does play a role.26
Gamma Motoneuron Hyperactivity
The gamma system modulates the length of the intrafusal fibers and is therefore responsible for establishing the firing thresholds for group Ia and group II neurons (Fig. 91-3). Incorrect threshold settings for the afferent neurons could lead to a hyperactive response. Evidence to support gamma hyperactivity is derived from analogy with the decerebrate cat, from selective blockade of the fusimotor system with local anesthetics, from the Jendrassik maneuver, and from comparison of the tendon jerk reflex and the H-reflex. Selective blockade of the fusimotor system has been used clinically in spastic patients. Injection of dilute procaine into the motor points of spastic and rigid muscles decreases muscle tone.27 This effect seems to support the view that spasticity is related to an imbalanced fusimotor system in that stretch reflexes are abolished as a result of block of the gamma system but voluntary muscle power is maintained by the output of unaffected larger alpha motoneurons. However, at a different site, intrathecal and epidural injections of local anesthetic did not show a link between increased fusimotor activity and spasticity.28 The strongest evidence against the gamma hyperactivity hypothesis comes from the finding that the discharge rate of primary spindle endings is the same in spastic and normal individuals.29 Increased gamma activity should lead to increased sensitivity of the discharge rate of primary spindle endings in spastic patients. Although the gamma hyperactivity hypothesis has been influential, the evidence to support it is indirect, inconclusive, and circumstantial.30
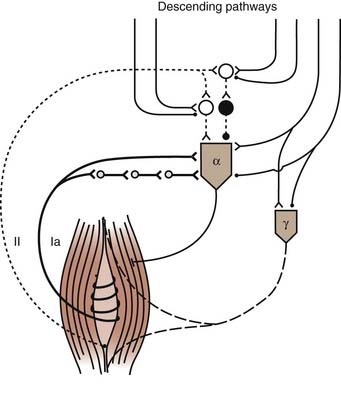
FIGURE 91-3 Muscle spindle afferents and fusimotor innervation. Ia pathways are described in Figure 91-2. Two pathways from group II afferents (dotted line) to homonymous motoneurons are represented: synapses are angle signs if excitatory and small filled circles if inhibitory. Interneurons are open circles if excitatory and filled circles if inhibitory. Notice that alpha (α) and gamma (γ) motoneurons are controlled by descending pathways.
(Redrawn from Pierrot-Deseilligny E. Pathophysiology of spasticity. Triangle. 1983;22:165-174.)
Decreased Ib Inhibition
Golgi tendon organs are sensitive to stretch because they lie in series with the muscle. On muscle stretch, impulses from Ib fibers are transmitted to homonymous motoneuron pools and heteronymous pools acting synergistically to inhibit stretch.31 Lack of inhibition can result in an increased response to stretch and thereby contribute to spasticity. This has been demonstrated by Delwaide and Oliver, who showed that Ib inhibition was markedly reduced in spastic patients.32
Summary of Mechanisms
Contributions of the different spinal mechanisms to hyperreflexia are summarized in Table 91-1. The information in this table should be treated with caution because of considerable variability in the tests used and in the types of spasticity in the different studies. Under the column “Mechanism” we have listed the putative mechanism by which hyperreflexia could occur. The evidence is strong that decreased presynaptic inhibition, decreased inhibition from supraspinal centers, and decreased Golgi tendon organ inhibition can each play a role in hyperreflexia. However, the importance of each mechanism may vary in the different types of injuries that cause hyperreflexia. Research conducted over the past 20 years makes it strikingly clear that different causes of spasticity can have opposing effects on the patterns of inhibition and excitation observed in various spinal pathways.
Why Do Spinal Circuits Malfunction?
Abnormal Descending Control
One way to consider the diminished motor control in patients with spasticity is from a developmental perspective.39,40 It is well known that babies demonstrate a mass of uncontrolled reflexes. Before a baby can stand, the flexor reflexes in the lower limbs must be inhibited and the extensor reflexes enhanced to brace the limbs against gravity, a function of the vestibulospinal and facilitatory reticulospinal tracts. After the child has learned how to stand, the next step is walking. For the child to walk, the extensor pattern of reflex standing in the lower limbs must be inhibited, and the flexor synergy of the lower limbs must be integrated into a walking pattern. This requires involvement of the motor cortex to inhibit extensor activity and facilitate flexor activity.
As maturity is reached, the pyramidal tract exerts control over the direct connections to the anterior horn cells, in conjunction with the basal ganglia, cerebellum, and brainstem. If the motor cortex or its projections are damaged, the brainstem exerts primary control. If the brainstem assumes complete control, a decorticate posture consisting of flexion of the upper limbs and extension of the lower limbs develops. In cerebral spasticity, there is enhancement of the stretch reflexes in the upper limb flexors and lower limb extensors. If the spinal cord is damaged, even brainstem control is disrupted such that flexor reflexes and stretch reflexes are released. This can lead to any or all of the following physical signs40:
Transection of the spinal cord results acutely in spinal shock, abolition of reflexes, and muscle flaccidity.41 Slowly, over a period of weeks, muscle tone and reflex activity reappear and then become excessive. These increases may be caused by loss of descending control, but this loss cannot explain the reflex exaggeration as time proceeds. This is best explained by reorganization of the spinal cord circuitry and alterations in levels of presynaptic inhibition.15 A detailed review of the neurophysiology of spinal spasticity has been provided by Ashby and McCrea.42
Local Changes at the Spinal Level
Most evidence for plastic changes at the spinal level is based on experience with spinal cord hemisection.43,44 Changes in the spinal cord may be structural or functional, or both. Collateral sprouting of peripheral afferents has been shown to occur. In a hemisected spinal cord, a greater number of dorsal root fibers are eventually found on the hemisected side than on the intact side. Ia fibers may eventually constitute 10% of the synapses on motoneurons instead of the normal 1%, which may account for exaggerated tendon reflexes.41
There is evidence that changes can occur in the spinal circuitry of humans who have suffered perinatal injuries to the immature nervous system.45–47 In adults who suffered birth-onset injuries, rapid stretch of the soleus muscle elicits a reflex in the soleus and tibialis anterior muscles. This phenomenon of reciprocal excitation is in marked contrast to the normal occurrence of reciprocal inhibition in the nonstretched muscle, thus suggesting that some disorders (e.g., cerebral palsy) may be characterized by abnormal spinal cord circuitry and brain damage.
Changes in Muscle Fiber and Connective Tissue
Neural mechanisms initiate spasticity. However, these altered neural mechanisms lead to numerous secondary changes in muscle that may be partially responsible for the symptoms of spasticity. The mechanical properties of muscles and joints in spastic limbs can change in several ways.48 The degree of abnormality of the muscles of children with cerebral palsy depends on which muscle groups are involved.49 Examples have been found of atrophy, hypertrophy, and myopathy. During long-term spastic hemiplegia in human patients, some motor units develop increased fatigability and prolonged twitch-contraction times, which causes changes in the dynamic properties of muscle.50 Several studies on locomotion and interlimb coordination have suggested that when hypertonia is present without concomitant changes on electromyography, the hypertonia must be caused by altered muscle properties.51–57 For example, histochemistry and morphometry of the spastic muscle of four individuals revealed increased atrophy of muscle fibers (especially type II) and a predominance of type I fibers.58
In some patients, another likely explanation for spastic hypertonia is that some muscle fibers are replaced by connective tissue. In the more severely atrophic muscles, an increase in the amount of perimysial-endomysial connective and interstitial tissue and an increase in the number of internal nuclei have been found.49 Summarizing many studies, Dietz and Sinkjaer have recently argued that the degree of hyperreflexia found in patients does not correlate with functional loss and that treatment of spasticity should be directed at improving movement and not simply decreasing overactive reflexes.9 They also point out that changes in muscles are important in producing rigidity and that abnormal spinal circuits interfere with voluntary movements and are a major cause of disability in spastic patients.
Measurement of Spasticity
Clinical Evaluation
Clinically, spasticity is graded qualitatively. Muscle response to stretch is judged by the deep tendon reflexes elicited by a reflex hammer and by the spread of the response to other muscle groups. Symmetry of response is more important than the strength of the response, and even a few beats of clonus in both ankles may be normal. Resistance to passive stretch can be a gauge of spasticity but often reflects other problems, such as contractures or ankylosis of the joint being tested. Spasticity may vary because of factors such as fatigue and emotional stress. Normal individuals may even experience sustained clonus under these conditions.59 Position (i.e., vestibular input) dramatically changes spasticity. For example, patients with partial cord injuries, when tested in the upright position or in a wheelchair, may demonstrate much less resistance than when supine. Voluntary movement is assessed by analysis of gait and the ability to generate rapid successive movements.
To provide a simple grading system that would be useful for repeated clinical examinations, Ashworth devised the scale shown in Table 91-2.60 A graded scale is appropriate because the response of a muscle to stretch can vary considerably in a normal individual, and a spastic patient’s increased response represents the higher end of a continuum. In general, the categories are distinct enough that changes in spasticity can be judged accurately during repeated examinations. However, one problem with the scale is that the scores of many patients cluster in the middle. Thus, it is worth considering the modified Ashworth scale, which tries to account for this problem by adding an extra scoring category.61 Another problem with the scale is that factors that affect the response of a muscle to stretch are not differentiated (i.e., reflex abnormalities versus contractures). Numerous other outcome measures can be used to facilitate effective management of spasticity and are summarized by Pierson.62 Objective tests should be used whenever possible because placebo effects have been noted in up to 50% of patients.63
SCORE* | CHARACTERISTIC |
---|---|
1 | No increase in tone |
2 | Slight increase in tone; a “catch” occurs when the affected part is moved in flexion and extension |
3 | More marked increase in tone but affected parts easily flexed |
4 | Considerable increase in tone; passive movement difficult |
5 | Affected parts rigid in flexion or extension |
* The original Ashworth scale was scored on a scale of 1 to 4.
(Modified Ashworth Scale)
Passive Quantifiable Tests
A normal limb can be extended passively or flexed without encountering resistance. This is not the case for a spastic limb. After movement of a spastic limb has been initiated, the following characteristics can be recognized: resistance that increases gradually through the movement, resistance that may suddenly subside as the limits of range of motion are reached (i.e., clasp-knife phenomenon), resistance that is proportional to the velocity of stretch, and a stretch reflex that is dependent on position.64
Quantitative measures of spasticity must record the velocity of the stretch, the resistance to stretch, and if possible, the electromyographic response of the stretched muscle. Several such tests have been developed.65–68 One of the simplest evaluations appropriate for clinical use is a drop test.69 To perform this test, the patient is placed supine. The patient’s leg is lifted to a horizontal position and then allowed to fall freely as the knee angle and quadriceps response are recorded. Figure 91-4 shows how different degrees of spasticity are manifested on the knee goniogram and quadriceps electromyogram.
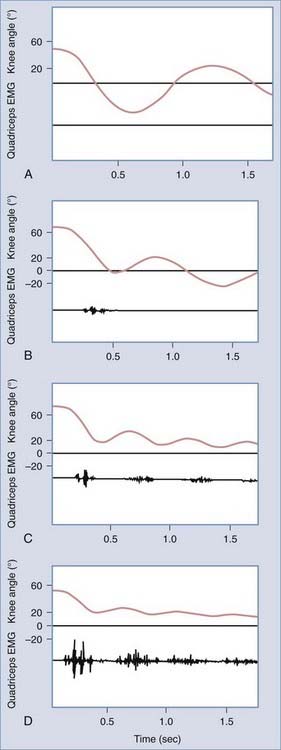
FIGURE 91-4 Degrees of spasticity: absent (A), slight (B), moderate (C), and severe (D). EMG, electromyogram.
(Adapted from Bajd T, Bowman B. Testing and modeling of spasticity. J Biomed Eng. 1982;4:90-97.)
A wide variety of electrophysiologic tests can be used to explore the neurophysiologic mechanisms that underlie spasticity. A comprehensive explanation of these tests is provided by Sehgal and McGuire.14
Reflexes are more easily measurable than activities of daily living but are less representative of changes in general motor performance.70 It is important to bridge this gap with the use of isokinetic measurements, gait studies, and studies of general movements.
Active Tests of Movement
Direct Interference in Movement Capability
Deficits in the ability to generate movements can be tested and analyzed in numerous ways. The most common methods have been studies of unidirectional or reciprocal movements, use of ergometers, and evaluation of gait.53,71–73 The definition of spasticity presented at the beginning of this chapter suggests that a reflex can be elicited in the muscle antagonistic to a movement. The evidence for this assertion, however, is contradictory. It has been suggested that there is no relationship between hyperactive reflexes and movement deficits,9,73 but it has also been shown that reflexes in patients with mild spasticity are suppressed during voluntary movement.74 It has been suggested that abolition of hyperactive stretch reflexes does not necessarily improve motor performance.3,8,28 However, direct evidence showing the effect of reflex involvement in movement impairment has also been observed.5,72,75,76 Boorman and colleagues found loss of supraspinal control over spinal inhibitory mechanisms during cycling movements in patients with spinal cord injury.77
Studies of gait in spastic patients have described abnormal features of locomotion with the use of force plate recordings, accelerometry, and photography, alone or in conjunction with electromyography. The findings emphasize large interindividual variations in the underlying mechanisms. For instance, a specific clinical entity (e.g., footdrop) can be caused by diverse mechanisms.78 Frequently, the observed paralysis of the foot is caused by pull of the hypertonic triceps surae muscle.
Measurement of Spasms
Lesions of the central nervous system often produce spasms and spasticity. The lesions are often in the spinal cord and cause a release of inhibition that results in exaggeration of the flexor reflex. Spasms are often associated with pain, can compromise standing and sitting, and may facilitate the development of contractures. One of the few objective methods for documenting spasms involves placing electrodes over the ankle flexors and using a rectifier circuit to create an envelope of electromyographic activity that is easy to quantify visually or electronically.79 Patients can then be tested during sleep (approximately 6 to 9 hours).
Treatment of Spasticity
Consideration of the pathophysiology also makes it clear that a wide variety of measures can be taken to reduce motoneuron hyperexcitability. Alterations in one or more of the pathways and connections of the reflex arc have been postulated to play an instrumental role in spasticity. However, studies that selectively reduce spasticity by allegedly influencing a single mechanism should be viewed with caution because that mechanism may not be responsible for the spasticity.12 Reduction of excitatory input from suprasegmental levels, spinal circuits, or dorsal roots reduces motoneuron drive, as does activation of inhibitory pathways. Even if a particular input is not the primary cause of motoneuron excitation, reducing or eliminating it may yield the desired therapeutic effect. The success of therapy directed at one mechanism influencing the motoneuron pool does not imply that the cause of the spasticity is understood. It is rare that a drug or operative treatment has a simple physiologic effect. Therapy is empirical, and results must be interpreted with care because responses among patients can be significantly different.
Intrathecal Baclofen
Physiologic Effects of Baclofen
Baclofen was designed to be a lipophilic γ-aminobutyric acid (GABA) agonist that could pass through the blood-brain barrier.80 However, baclofen only partially permeates from blood to brain and does not fully resemble GABA in its physiologic actions.81 Several types of receptors for GABA are found in the brain and spinal cord, and baclofen affects only the B type. This receptor has been identified and sequenced.82 It is a transmembrane protein that affects calcium and potassium channels. Immunohistologic staining of the spinal cord has shown a high concentration of GABAB receptors in layer II of the dorsal gray matter.83 Physiologic experiments on isolated perfused spinal cords have demonstrated that baclofen produces a profound reduction in monosynaptic and polysynaptic spinal reflexes.84 This effect is caused by activation of the GABAB receptors, which reduces the influx of calcium into the presynaptic terminals of afferent fibers, the result being a reduction in the release of excitatory transmitters. Baclofen can also affect the postsynaptic membrane by increasing potassium influx, thereby stabilizing or increasing the membrane potential and inhibiting neuronal firing.85,86
The sum of these presynaptic and postsynaptic effects is to decrease drive on the motoneurons. Diazepam, the other centrally active antispastic medication, works differently. It binds to the presynaptic membrane and facilitates GABA-mediated presynaptic inhibition. Unlike baclofen, which directly activates the receptor, diazepam works only when GABA is released, and it enhances response to the transmitter (Fig. 91-5).87
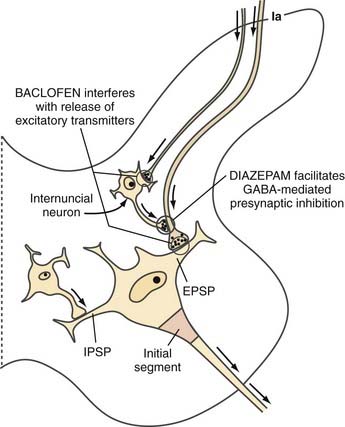
FIGURE 91-5 Enlargement of the left half of Figure 91-1. Synaptic mechanisms at the spinal level are responsible for excitatory postsynaptic potential (EPSP) and two types of inhibition: inhibitory postsynaptic potential (IPSP), and presynaptic inhibition. The sites of action of γ-aminobutyric acid (GABA), baclofen, and diazepam at the spinal level are schematically illustrated.
(Redrawn from Young RR, Delwaide PJ. Drug therapy: spasticity. Part 2. N Engl J Med. 1981;304:96-99.)
The physiologic effects of baclofen have been studied most frequently in the normal spinal cord. After spinal cord injury, spasticity develops slowly and is related to plastic changes in the spinal cord circuitry. The physiology of the damaged spinal cord is significantly changed, and so is its response to medications. Tests have shown that after a cervical injury the rat lumbar cord increases the number of its GABAB receptors by 30%.88 Other experiments in rats and cats have demonstrated that bladder reflexes change after cord injury and that the modified reflexes are inhibited by morphine and baclofen.89 These animal findings are supported by clinical observations. Intrathecal baclofen given to normal patients does not interfere with movement or decrease strength, but the same dose given to a spastic patient markedly decreases spasticity and muscle tone. Ninety-six percent or more of patients with spasticity from multiple sclerosis or spinal cord injury have responded to intrathecal baclofen in double-blind studies.90 The reason that baclofen is so effective in reducing spasticity is that the same changes in the spinal cord that produce spasticity increase sensitivity to baclofen.
Kinetics and Distribution of Intrathecal Baclofen
Baclofen, like morphine, is primarily water soluble, which means that only a small amount crosses the blood-brain barrier.81 Similarly, little of the drug that is introduced into cerebrospinal fluid is lost by movement across membranes into the systemic circulation. Therefore, cerebrospinal fluid flow is the only distribution source of intrathecal baclofen to the spinal cord. When a bolus of baclofen is delivered via lumbar puncture, it mixes rapidly in the lumbar subarachnoid space and is gradually carried upward along the spinal cord.91 In 3 to 6 hours it reaches the brainstem and then travels over the convexities, where it is eliminated into the systemic circulation at the arachnoid granulations. Bolus administration leads to transient but very high drug levels at the spinal cord and, later, at the brainstem. The half-life of a bolus of baclofen in cerebrospinal fluid is about 90 minutes.92 It is stable in cerebrospinal fluid and does not become metabolized by tissue.
If baclofen is given by slow infusion, a different distribution is achieved. The concentration gradually reaches a steady state in which the same amount of baclofen is being removed from cerebrospinal fluid as is being infused; this occurs at about 12 to 18 hours (i.e., seven times the half-life). As has been measured in patients, the final steady-state concentration is directly proportional to the drug infusion rate.93 Other measurements in patients have demonstrated that in the steady state, the concentration of baclofen is decreased to one quarter from the lumbar cistern to the high cervical region. The manner in which the concentration varies along the spinal cord is indicated by indium 111 flow studies of cerebrospinal dynamics in patients with implanted pumps.91 These tests indicate that the decrease in concentration is gradual and almost linear along the cord (Fig. 91-6).
The results of these kinetic and distribution studies have practical consequences:
Another point of considerable clinical significance is that to have its physiologic effect, baclofen must travel from cerebrospinal fluid to the spinal cord receptors, a distance of 2 to 5 mm through cord tissue. Diffusion is a very slow process. This accounts for the 45- to 60-minute delay from the time that a bolus dose is injected until spasticity is reduced. After the receptors have been reached, diffusion back to cerebrospinal fluid is equally slow. A single bolus dose may reduce spasticity for 4 to 12 hours; its maximal effect occurs when the level in cerebrospinal fluid has decreased to almost zero.94 This slow diffusion from tissue also means that a large, single overdose causes long-lasting respiratory depression and coma, even after cerebrospinal fluid levels have been reduced. When giving medication intrathecally, the physician must always be aware that the clinical effects are slow to appear and equally slow to clear because the drug requires time for diffusion into the spinal cord, and the cord tissue acts as a reservoir after it is loaded.
Efficacy of Intrathecal Baclofen for Spinal Spasticity
The most effective use of oral baclofen has been for the treatment of spasticity caused by spinal cord injury or multiple sclerosis. The initial studies of intrathecal delivery were conducted in these patient groups after oral medications showed limited success or had unacceptable side effects such as drowsiness. In this well-defined patient population, a bolus of 50 to 100 µg of intrathecal baclofen reduced abnormal muscle tone 2 or more points on the Ashworth scale for almost all patients. If patients had spasms, they were also significantly reduced. The short-term effect could be maintained with constant delivery. Individual and multicenter studies in the United States and Europe have demonstrated that control of spasticity and spasms can be achieved over a period of years by using implanted drug pumps to deliver baclofen (Table 91-3 and Fig. 91-7).
Drug Side Effects
The side effects of intrathecal baclofen are similar to those of oral baclofen and are dose related. Although some protection from central side effects is afforded by the fact that the baclofen concentration is higher in the lumbar region than at the brainstem, increasing dosage can lead to adverse effects. Common problems from high dosage include drowsiness, mental confusion, lightheadedness, and ataxia. Weakness can be induced in some patients, as can loss of function because of reduced muscle tone. A 10% to 20% reduction in dosage usually eliminates these symptoms. Bolus administration, used for testing before implantation of a pump, is more frequently associated with these side effects and occasionally produces hypotension, nausea, and respiratory depression. A large overdose, in the range of 1 to 20 mg, results in coma, flaccidity, hypotension, and respiratory depression.99 If an overdose occurs, the patient must be kept under surveillance with apnea monitoring and, if necessary, be provided with ventilatory support. No deaths from overdose have been reported, but the intrathecal route of drug administration can result in potent and serious side effects. Treatment of a moderate overdose with physostigmine (0.5 to 2 mg) often reverses the somnolence and respiratory effects.100 This treatment does not work for large overdoses and should not be given to patients with heart conduction defects. The central effects of an overdose should clear in 24 to 48 hours.
Tolerance to baclofen develops in most patients. Over the first 6 to 12 months, the dose of baclofen required to achieve a given clinical effect usually doubles and then eventually stabilizes.90 In a few patients, tolerance may be a significant problem that necessitates “drug holidays” for several weeks or a switch to intrathecal morphine, which shares some of the antispastic effects of baclofen. Most often, the need for increasing dosage after the first year is related to a problem with drug delivery through the catheter rather than true tolerance.
A rare life-threatening baclofen withdrawal syndrome of high fever, altered mental state, and profound muscular rigidity that can lead to fatal rhabdomyolysis has been described. The most effective treatment is rapid reinstatement of intrathecal baclofen. Oral and intravenous medications, including high-dose benzodiazepines, and supportive care can help,101 but in severe cases intrathecal baclofen should be given by lumbar puncture or external catheter. Patients with preexisting autonomic dysreflexia are particularly at risk for this withdrawal syndrome.101
Delivery Systems
The major cause of disruption of drug delivery is catheter malfunction. The implanted catheters are thin walled and small caliber so that they can be passed easily into the subarachnoid space without causing injury to the nerve roots.90 Despite anchoring devices, the flimsy catheters can pull out of position because of movement. The catheters can also tear, disconnect, or occlude. Frequently, the problem can be diagnosed by plain radiography. If the cause is not obvious, indium 111 can be placed in the pump and the patient scanned at 24 and 48 hours to check catheter patency.102 The indium 111 study has the advantage of showing the flow of cerebrospinal fluid, and it can demonstrate a subarachnoid block caused by arachnoiditis or fibrosis around the catheter tip. Sometimes, a pinhole leak in a catheter cannot be seen on any study and the cause is found only when the catheter is replaced. An alternative is to inject a radiopaque dye under fluoroscopic guidance into the pump’s side port and look for blockage or a leak outside the subarachnoid space.
Long-term management of patients requires regular pump refills, dose adjustments, and diagnosis and treatment of complications.103 Despite these requirements, the dropout rate of implanted patients is quite low. In a 7-year study, no patients were lost because of medication side effects, and although more than 30% had pump or catheter problems requiring operative repair, only 10% decided to not continue long-term treatment.90 The key to management is a well-trained nurse practitioner who can help with patient education, pump refills, and dose adjustments.
Patient Selection
The U.S. Food and Drug Administration first approved pump implantation and intrathecal baclofen treatment of spasticity caused by multiple sclerosis or spinal cord injury on the basis of several double-blind, multicenter studies. Approval was then extended to patients with spasticity of cerebral origin, including those with cerebral palsy. These and other conditions in which spasticity may be reduced with baclofen are listed in Table 91-4. In general, if a patient has the classic signs of spasticity, baclofen can decrease them. Athetosis and generalized dystonia do not usually improve, although exceptions are found.103,111 Focal, painful lower limb dystonias may be helped considerably.114 Stiff man syndrome, in which spinal GABA-producing neurons are thought to be lost and the patient suffers from episodes of severe axial and limb spasms and rigidity, is markedly improved.113
SPASTICITY-ASSOCIATED CONDITION | REFERENCE |
---|---|
Spinal cord injury | See Table 91-3 |
Cerebral palsy | Albright et al., 1993104 |
Albright et al., 1991105 | |
Hydromyelia | Case reports |
Spondylosis | |
Progressive lateral sclerosis | Case reports |
Traumatic brain injury | Meythaler et al., 1999106 |
Meythaler et al., 1997107 | |
Becker et al., 1997108 | |
Rifici et al., 1994109 | |
Saltuari et al., 1992110 | |
Dystonias | |
Axial | Ford et al., 1996111 |
Cerebral palsy | Albright et al., 1993104 |
Albright et al., 1991105 | |
Distal extremity—foot | Meythaler et al., 1999112 |
Miscellaneous | |
Stiff man syndrome | Penn and Mangieri, 1993113 |
Painful leg syndrome moving toes | Penn and Gianino, 1995114 |
Albright AL, Cervi A, Singletary J. Intrathecal baclofen for spasticity in cerebral palsy. JAMA. 1991;265:1418-1422.
Coffey RJ, Edgar TS, Francisco GE, et al. Abrupt withdrawal from intrathecal baclofen: recognition and management of a potentially life-threatening syndrome. Arch Phys Med Rehabil. 2002;83:735-741.
Corcos D, Gottlieb G, Penn R, et al. Movement deficits caused by hyperexcitable stretch reflexes in spastic humans. Brain. 1986;109:1043-1058.
Dietz V, Quintern J, Berger W. Electrophysiological studies of gait in spasticity, and rigidity: evidence that altered mechanical properties of muscle contribute to hypertonia. Brain. 1981;104:431-439.
Dietz V, Sinkjaer T. Spastic movement disorder: impaired reflex function and altered muscle mechanics. Lancet Neurol. 2007;6:725-733.
Hagbarth K, Wallin G, Lofstedt L. Muscle spindle responses to stretch in normal and spastic subjects. Scand J Rehab Med. 1973;5:156-159.
Katz R, Pierrot-Deseilligny E. Recurrent inhibition in humans. Prog Neurobiol. 1999;57:325-355.
Kroin J, Ali A, York M, et al. The distribution of medication along the spinal canal after chronic intrathecal administration. Neurosurgery. 1993;33:226-230.
Lance J. The control of muscle tone, reflexes, and movement: Robert Wartenberg Lecture. Neurology. 1980;30:1303-1313.
Landau W. Spasticity: the fable of the neurological demon and the emperor’s new therapy. Arch Neurol. 1974;31:217-219.
McCouch G, Austin G, Liu C, et al. Sprouting as a cause of spasticity. J Neurophysiol. 1958;21:205-216.
Ochs G, Struppler A, Meyerson B, et al. Intrathecal baclofen for long-term treatment of spasticity: a multi-centre study. J Neurol Neurosurg Psychiatry. 1989;52:933-939.
Penn R, Kroin J. Intrathecal baclofen alleviates spinal cord spasticity. Lancet. 1984;1:1078.
Penn R, Savoy S, Corcos D, et al. Intrathecal baclofen for severe spinal spasticity: a double-blind crossover study. N Engl J Med. 1989;320:1517-1521.
Pierrot-Deseilligny E. Electrophysiological assessment of the spinal mechanisms underlying spasticity. Electroencephalogr Clin Neurophysiol Suppl. 1990;41:264-273.
Price G, Wilkin G, Turnbull M, et al. Are baclofen-sensitive GABA-B receptors present on primary afferent terminals of the spinal cord? Nature. 1984;307:71-74.
Rosenson A, Ali A, Fordham E, et al. Indium-111 DPTA flow study to evaluate surgically implanted drug delivery system. Clin Nucl Med. 1990;15:154-156.
Sherrington C. The Integrative Action of the Nervous System. New Haven, CT: Yale University Press; 1906.
Young R, Delwaide P. Drug therapy: spasticity. Part 2. N Engl J Med. 1981;304:96-99.
Zieglglansberger W, Howe J, Sutor B. The neuropharmacology of baclofen. In: Muller H, Zierski J, Penn R, editors. Local Spinal Therapy of Spasticity. Berlin: Springer-Verlag; 1988:37-49.
1 Penn R, Savoy S, Corcos D, et al. Intrathecal baclofen for severe spinal spasticity: a double-blind crossover study. N Engl J Med. 1989;320:1517-1521.
2 Müller H. Treatment of severe spasticity: results of a multicenter trial conducted in Germany involving the intrathecal infusion of baclofen by an implantable drug delivery system. Rev Eur Technol Biomed. 1991;13:184-186.
3 Landau W. Spasticity: the fable of the neurological demon and the emperor’s new therapy. Arch Neurol. 1974;31:217-219.
4 Lance J. Symposium synopsis. In: Feldman R, Young R, Koella W, editors. Spasticity: Disordered Motor Control. Chicago: Year Book; 1980:485-494.
5 Jackson J. On the comparative study of diseases of the nervous system. In: Taylor J, editor. Selected Writings of John Hughlings Jackson. New York: Basic Books; 1958:393-410.
6 Corcos D, Gottlieb G, Penn R, et al. Movement deficits caused by hyperexcitable stretch reflexes in spastic humans. Brain. 1986;109:1043-1058.
7 Dietz V. Spasticity: exaggerated reflexes or movement disorder? In: Forssberg H, Hirschfeld H, editors. Movement Disorders in Children. Medicine and Sports Science Series, Vol 36. Basel: Karger; 1992:225-233.
8 Landau W. Spasticity: What is it? What is it not? In: Feldman R, Young R, Koella W, editors. Spasticity: Disordered Motor Control. Chicago: Year Book; 1980:17-24.
9 Dietz V, Sinkjaer T. Spastic movement disorder: impaired reflex function and altered muscle mechanics. Lancet Neurol. 2007;6:725-733.
10 Rushworth G. Some pathophysiological aspects of spasticity and the search for rational and successful therapy. Int Rehabil Med. 1977;2:460-465.
11 Pierrot-Deseilligny E. Pathophysiology of spasticity. Triangle. 1983;22:165-174.
12 Pierrot-Deseilligny E. Electrophysiological assessment of the spinal mechanisms underlying spasticity. Electroencephalogr Clin Neurophysiol Suppl. 1990;41:264-273.
13 Pierrot-Deseilligny E, Mazieres L. Spinal mechanisms underlying spasticity. In: Delwaide P, Young R, editors. Clinical Neuro-physiology in Spasticity. Amsterdam: Elsevier; 1985:63-76.
14 Sehgal N, McGuire J. Beyond Ashworth. Electrophysiological quantification of spasticity. Phys Med Rehabil Clin N Am. 1998;9:949-979.
15 Calancie B, Broton J, Klose K, et al. Evidence that alterations in presynaptic inhibition contribute to segmental hypo- and hyperexcitability after spinal cord injury in man. Electroencephalogr Clin Neurophysiol. 1993;89:177-186.
16 Faist M, Mazevet D, Dietz V, et al. A quantitative assessment of presynaptic inhibition of Ia afferents in spastics: differences in hemiplegics and paraplegics. Brain. 1994;117:1449-1455.
17 Hultborn H, Wigstrom H. Motor response with long latency and maintained duration evoked by activity in Ia afferents. In: Desmedt J, editor. Spinal and Supraspinal Mechanisms of Voluntary Control and Locomotion: Progress in Clinical Neurophysiology. Basel: Karger; 1980:99-116.
18 Hagbarth K. The effect of muscle vibration in normal man and in patients with motor disorders. In: Desmedt J, editor. New Developments in Electromyography and Clinical Neurophysiology. Basel: Karger; 1973:428-443.
19 Sherrington C. The Integrative Action of the Nervous System. New Haven, CT: Yale University Press; 1906.
20 Hultborn H. Transmission in the pathway of reciprocal Ia inhibition to motoneurons and its control during the tonic stretch reflex. In: Homma S, editor. Understanding the Stretch Reflex: Progress in Brain Research. Amsterdam: Elsevier; 1976:235-255.
21 Tanaka R. Reciprocal Ia inhibition during voluntary movements in man. Exp Brain Res. 1974;21:529-540.
22 Yanagisawa N, Tanaka R, Ito Z. Reciprocal Ia inhibition in spastic hemiplegia of man. Brain. 1976;99:555-574.
23 Katz R, Pierrot-Deseilligny E. Recurrent inhibition in humans. Prog Neurobiol. 1999;57:325-355.
24 Hultborn H, Pierrot-Deseilligny E. Changes in recurrent inhibition during voluntary soleus contractions in man studied by an H-reflex technique. J Physiol. 1979;297:229-251.
25 Katz R, Pierrot-Deseilligny E. Recurrent inhibition of motoneurons with upper motor lesions. Brain. 1982;105:103-124.
26 Noth J. Trends in the pathophysiology and pharmacotherapy of spasticity. J Neurol. 1991;238:131-139.
27 Rushworth G. Spasticity and rigidity: An experimental study and review. J Neurol Neurosurg Psychiatry. 1960;23:99-118.
28 Landau W, Weaver R, Hornbein T. Fusimotor nerve function in man: differential nerve block studies in normal subjects and in spasticity and rigidity. Arch Neurol. 1960;3:10-23.
29 Hagbarth K, Wallin G, Lofstedt L. Muscle spindle responses to stretch in normal and spastic subjects. Scand J Rehabil Med. 1973;5:156-159.
30 Burke D. Critical examination of the case for or against fusimotor involvement in disorders of muscle tone. Adv Neurol. 1983;39:133-150.
31 Eccles J, Eccles R, Lundberg A. Synaptic actions on motoneurons caused by impulses in Golgi tendon organ afferents. J Physiol (Lond). 1957;138:227-252.
32 Delwaide P, Oliver E. Short-latency, autogenic inhibition (IB inhibition) in human spasticity. J Neurol Neurosurg Psychiatry. 1988;51:1546-1550.
33 Crone C, Nielsen J, Petersen N, Ballegaard M, et al. Disynaptic reciprocal inhibition of ankle extensors in spastic patients. Brain. 1994;117:1161-1168.
34 Ashby P, Wiens M. Reciprocal inhibition following lesions of the spinal cord in man. J Physiol (Lond). 1989;414:145-157.
35 Shefner J, Berman S, Sarkarati M, et al. Recurrent inhibition is increased in patients with spinal cord injury. Neurology. 1992;42:2162-2168.
36 Mazzocchio R, Rossi A. Involvement of spinal recurrent inhibition in spasticity: further insight into the regulation of Renshaw activity. Brain. 1997;120:991-1003.
37 Raynor E, Shefner J. Recurrent inhibition is decreased in patients with amyotrophic lateral sclerosis. Neurology. 1994;44:2148-2153.
38 Katz R, Rymer W. Spastic hypertonia: mechanisms and measurement. Arch Phys Med Rehabil. 1989;70:144-155.
39 Lance J. The control of muscle tone, reflexes, and movement: Robert Wartenberg Lecture. Neurology. 1980;30:1303-1313.
40 Lance J. Pathophysiology of spasticity and clinical experience with baclofen. In: Feldman R, Young R, Koella W, editors. Spasticity: Disordered Motor Control. Chicago: Year Book; 1980:185-203.
41 Woolsey R. Symposium on spasticity and spasms. J Am Paraplegia Soc. 1982;5:3-9.
42 Ashby P, McCrea D. Neurophysiology of spinal spasticity. In: Davidoff R, editor. Handbook of the Spinal Cord. New York: Marcel Decker; 1987:119-143.
43 Malmsten J. Time course of segmental reflex changes after chronic spinal cord hemisection in the rat. Acta Physiol Scand. 1983;119:435-443.
44 McCouch G, Austin G, Liu C, et al. Sprouting as a cause of spasticity. J Neurophysiol. 1958;21:205-216.
45 Gottlieb G, Myklebust B, Penn R, et al. Reciprocal excitation of muscle antagonists by the primary afferent pathway. Exp Brain Res. 1982;46:454-465.
46 Myklebust B, Gottlieb G, Penn R, et al. Reciprocal excitation of antagonistic muscles as a differentiating feature in spasticity. Ann Neurol. 1982;12:367-374.
47 Corcos D, Pfann K, Penn R. The control of movement in Parkinson’s disease and spasticity: role of inhibition and excitation. In: Crockard A, Hoff R, Hoff J, editors. Neurosurgery: The Scientific Basis of Clinical Practice. Boston: Blackwell Scientific Publications, 2000.
48 Lowenthal M, Tobis J. Contractures in chronic neurologic disease. Arch Phys Med Rehabil. 1957;38:640-645.
49 Castle M, Reyman T, Schneider M. Pathology of spastic muscle in cerebral palsy. Clin Orthop Relat Res. 1979;142:223-233.
50 Young J, Mayer R. Physiological alterations of motor units in hemiplegia. J Neurol Sci. 1982;54:401-412.
51 Ahlqvist G, Landin S, Wrobblewski R. Ultrastructure of skeletal muscle in patients with Parkinson’s disease and upper motor lesions. Lab Invest. 1975;32:673-679.
52 Berger W, Horstmann G, Dietz V. Tension development and muscle activation in the leg during gait in spastic hemiparesis: independence of muscle hypertonia and exaggerated stretch reflexes. J Neurol Neurosurg Psychiatry. 1984;47:1029-1033.
53 Dietz V, Berger W. Normal and impaired regulation of muscle stiffness in gait: a hypothesis about muscle hypertonia. Exp Neurol. 1983;79:680-687.
54 Dietz V, Berger W. Interlimb coordination of posture in patients with spastic paresis. Brain. 1984;107:965-978.
55 Dietz V, Quintern J, Berger W. Electrophysiological studies of gait in spasticity, and rigidity: evidence that altered mechanical properties of muscle contribute to hypertonia. Brain. 1981;104:431-439.
56 Edstrom L. Selective changes in the sizes of red and white muscle fibers in upper motor neuron lesions and parkinsonism. J Neurol Sci. 1970;11:537-550.
57 Hufschmidt A, Mauritz K. Chronic transformation of muscle in spasticity: a peripheral contribution to increased tone. J Neurol Neurosurg Psychiatry. 1985;48:676-685.
58 Dietz V, Ketelsen U, Berger W, et al. Motor unit involvement in spastic paresis: relationship between leg muscle activation and histochemistry. J Neurol Sci. 1986;75:89-103.
59 Gottlieb G, Agarwal G. Physiological clonus in man. Exp Neurol. 1977;54:616-621.
60 Ashworth B. Preliminary trial of carisoprodol in multiple sclerosis. Practitioner. 1964;192:540-542.
61 Bohannon R, Smith M. Interrater reliability of a modified Ashworth scale of muscle spasticity. Phys Ther. 1987;67:206-207.
62 Pierson S. Outcome measures in spasticity management. Muscle Nerve Suppl. 1997;6:S36-S60.
63 Burry H. Objective measurement of spasticity. Dev Med Child Neurol. 1972;14:508-510.
64 Bishop B. Spasticity: its physiology and management. Phys Ther. 1977;57:371-401.
65 Burke D, Gillies J, Lance J. The quadriceps stretch reflex in human spasticity. J Neurol Neurosurg Psychiatry. 1970;33:216-223.
66 Burke D, Gillies J, Lance J. Hamstring stretch reflex in human spasticity. J Neurol Neurosurg Psychiatry. 1971;34:231-235.
67 Gottlieb G, Agarwal G, Penn R. Sinusoidal oscillation of the ankle as a means of evaluating the spastic patient. J Neurol Neurosurg Psychiatry. 1978;41:32-39.
68 Lehmann J, Price R, deLateur B, et al. Spasticity: quantitative measurements as a basis for assessing effectiveness of therapeutic intervention. Arch Phys Med Rehabil. 1989;70:6-15.
69 Bajd T, Bowman B. Testing and modeling of spasticity. J Biomed Eng. 1982;4:90-97.
70 Pederson E. Evaluation of antispastic therapy. In: Delwaide P, Young R, editors. Restorative Neurology: Clinical Neurophysiology in Spasticity, vol 1. Amsterdam: Elsevier; 1985:221-223.
71 Benecke R, Conrad B, Meinck H, et al. Electromyographic analysis of bicycling on an ergometer for evaluation of spasticity of lower limbs in man. Adv Neurol. 1983;39:1035-1046.
72 Mizrahi E, Angel R. Impairment of voluntary movement by spasticity. Ann Neurol. 1979;5:594-595.
73 Sahrmann S, Norton B. The relationship of voluntary movement to spasticity in the upper motor syndrome. Ann Neurol. 1977;2:460-465.
74 McLellan D. Co-contraction and stretch reflexes in spasticity during treatment with baclofen. J Neurol Neurosurg Psychiatry. 1977;1:30-38.
75 Dimitrijevic M, Nathan P. Studies of spasticity in man. II. Analysis of stretch reflexes in spasticity. Brain. 1967;90:333-358.
76 Knuttson E, Martensson A. Dynamic motor capacity in spastic paresis and its relation to prime mover dysfunction, spastic reflexes and antagonistic coactivation. Scand J Rehabil Med. 1980;12:93-106.
77 Boorman G, Becker W, Morrice B, et al. Modulation of the soleus H-reflex during pedalling in normal humans and in patients with spasticity. J Neurol Neurosurg Psychiatry. 1992;55:1150-1156.
78 Dimitrijevic M, Faganel J, Sherwood A, et al. Activation of paralyzed leg flexors and extensors during gait in patients after stroke. Scand J Rehabil Med. 1981;13:109-115.
79 Pederson E, Klemar B, Torring J. Counting of flexor spasms. Acta Neurol Scand. 1979;60:164-169.
80 Faigle J, Keberle H, Degen P. Chemistry and pharmacokinetics of baclofen. In: Feldman R, Young R, Koella W, editors. Spasticity: Disordered Motor Control. Miami: Symposia Specialists, 1980.
81 Knutsson E, Lindbloom U, Martensson A. Plasma and cerebrospinal fluid levels of baclofen (Lioresal) at optimal therapeutic responses in spastic paresis. J Neurol Sci. 1974;23:473-484.
82 Kaupmann K, Huggel K, Heid J, et al. Expression cloning of GABAB receptors uncovers similarity to metabotropic glutamate receptors. Nature. 1997;386:239-246.
83 Price G, Wilkin G, Turnbull M, et al. Are baclofen-sensitive GABA-B receptors present on primary afferent terminals of the spinal cord? Nature. 1984;307:71-74.
84 Ault B, Evans R. The depressant action of baclofen on the isolated spinal cord of the neonatal rat. Eur J Pharmacol. 1981;71:357-364.
85 Azouvi P, Roby-Brami A, Biraben A, et al. Effect of intrathecal baclofen on the monosynaptic reflex in humans: Evidence for a postsynaptic action. J Neurol Neurosurg Psychiatry. 1993;56:515-519.
86 Zieglglansberger W, Howe J, Sutor B. The neuropharmacology of baclofen. In: Muller H, Zierski J, Penn R, editors. Local Spinal Therapy of Spasticity. Berlin: Springer-Verlag; 1988:37-49.
87 Young R, Delwaide P. Drug therapy: Spasticity. Part 2. N Engl J Med. 1981;304:96-99.
88 Kroin J, Bianchi G, Penn R. Intrathecal baclofen downregulates GABA B receptors in the rat substantia gelatinosa. J Neurosurg. 1993;79:544-549.
89 DeGroat W. Nervous control of the urinary bladder of the cat. Brain Res. 1975;87:201-211.
90 Penn R. Intrathecal baclofen for spasticity of spinal origin: Seven years of experience. J Neurosurg. 1992;77:236-240.
91 Kroin J, Ali A, York M, et al. The distribution of medication along the spinal canal after chronic intrathecal administration. Neurosurgery. 1993;33:226-230.
92 Kroin J. Which drugs? What space? In: Penn R, editor. Neurological Applications of Implanted Drug Pumps. New York: New York Academy of Sciences; 1988:40-47.
93 Muller H, Zierski J, Penn R. Local-Spinal Therapy of Spasticity. Berlin: Springer-Verlag; 1988.
94 Penn R, Kroin J. Intrathecal baclofen alleviates spinal cord spasticity. Lancet. 1984;1:1078.
95 Ochs G, Struppler A, Meyerson B, et al. Intrathecal baclofen for long-term treatment of spasticity: A multi-centre study. J Neurol Neurosurg Psychiatry. 1989;52:933-939.
96 Lazorthes Y, Sallerin-Caute B, Verdie J, et al. Chronic intrathecal baclofen administration for control of severe spasticity. J Neurosurg. 1990;72:393-402.
97 Loubser P, Narayan P, Sandin K, et al. Continuous infusion of intrathecal baclofen: Long-term effects on spasticity in spinal cord injury. Paraplegia. 1991;29:48-64.
98 Coffey JR, Cahill D, Steers W, et al. Intrathecal baclofen for intractable spasticity of spinal origin: Results of a long-term multicenter study. J Neurosurg. 1993;78:226-232.
99 Delhaas E, Brouwers J. Intrathecal baclofen overdose: Report of 7 events in 5 patients and review of the literature. Int J Clin Pharmacol Ther Toxicol. 1991;29:274-280.
100 Muller-Schwepe G, Penn R. Physostigmine in the treatment of intrathecal baclofen overdose: Reports of three cases. J Neurosurg. 1989;71:273-275.
101 Coffey RJ, Edgar TS, Francisco GE, et al. Abrupt withdrawal from intrathecal baclofen: Recognition and management of a potentially life-threatening syndrome. Arch Phys Med Rehabil. 2002;83:735-741.
102 Rosenson A, Ali A, Fordham E, et al. Indium-111 DPTA flow study to evaluate surgically implanted drug delivery system. Clin Nucl Med. 1990;15:154-156.
103 Naravan R, Loubser P, Jankovic J, et al. Intrathecal baclofen for intractable axial dystonia. Neurology. 1991;41:1141-1142.
104 Albright AL, Barron W, Fasick M, et al. Continuous intrathecal baclofen infusion for spasticity of cerebral origin. JAMA. 1993;270:2475-2477.
105 Albright AL, Cervi A, Singletary J. Intrathecal baclofen for spasticity in cerebral palsy. JAMA. 1991;265:1418-1422.
106 Meythaler JM, Guin-Renfroe SG, Grabb P, et al. Long-term continuously infused intrathecal baclofen for spastic-dystonic hypertonia in traumatic brain injury: 1-year experience. Arch Phys Med Rehabil. 1999;80:13-19.
107 Meythaler JM, Hadley M, McCary A. Prospective study on the use of continuously infused intrathecal baclofen for spasticity due to acquired brain injury: A preliminary report. J Neurosurg. 1997;87:415-419.
108 Becker R, Alberti O, Bauer BL. Continuous intrathecal baclofen infusion in severe spasticity after traumatic or hypoxic brain injury. J Neurol. 1997;244:160-166.
109 Rifici C, Kofler M, Kronenberg M, et al. Intrathecal baclofen application in patients with supraspinal spasticity secondary to severe traumatic brain injury. Funct Neurol. 1994;9:29-34.
110 Saltuari L, Kronenberg M, Marosi MJ, et al. Long-term intrathecal baclofen in supraspinal spasticity. Acta Neurol. 1992;14:195-207.
111 Ford B, Greene P, Louis E, et al. Use of intrathecal baclofen in the treatment of patients with dystonia. Arch Neurol. 1996;53:1241-1246.
112 Meythaler JM, Guin-Renfroe SG, Hadley MN. Continuously infused intrathecal baclofen for spastic-dystonic hemiplegia: a preliminary report. Am J Phys Med Rehabil. 1999;78:247-254.
113 Penn R, Mangieri E. Stiff-man syndrome treated with intrathecal baclofen. Neurology. 1993;43:2412.
114 Penn R, Gianino J, York M. Intrathecal baclofen for motor disorders. Mov Disord. 1995;10:675-677.