Chapter 142 Management of Penetrating Brain Injury
Epidemiology
In the United States, the incidence of PBI is estimated to be 12 per 100,000, higher than in any other developed nation.1 Approximately 15% to 20% of trauma-related noncombatant deaths are gunshot wounds to the head—the most common PBI in the civilian sector.1,1a Of the 178,876 traumatic brain injury (TBI) cases among U.S. military personnel in Iraq between 2000 and 2010, approximately 2% (more than 3500 cases) were PBIs—the majority due to explosions from improvised explosive devices combining blast injury and shrapnel that penetrate the cranium.2
History
Since early human history, healers have treated PBIs. Military combat provided the highest concentration of PBIs; therefore, the majority of advances in PBI management were conceived on or near the battlefield. The earliest recorded case series dates to 1700 BC, when Egyptians recorded treatment of four depressed skull fractures by free drainage of the intracranial cavity and dressing of the wound with grease. In the 5th century BC, the Greeks utilized trephination (Fig. 142-1) for injuries to the skull. In the 2nd century AD, Galen published his observations correlating motor deficits in gladiators to contralateral brain injury. In the 17th century, Richard Wiseman managed subdural hematomas and blunt trauma by evacuation of hematomas and foreign bodies. The advent of germ theory in the 19th century and Joseph Lister’s aseptic technique were first put to use for PBI during the Second Boer War. During World War I, as senior consultant to the American Expeditionary Force,3 Harvey Cushing brought mortality down from 54.4% to 28.8% by establishing the meticulous technique of craniectomy, débridement, and dural closure. He brought Wilhelm Roentgen’s (Röntgen’s) novel x-ray to the operating theater for the localization and extraction of foreign bodies—which he believed served as nidi for future infection. Cushing’s techniques remained the standard of care, unchanged for more than 50 years. Survival was doubled in World War II after the introduction of antibiotics and Hugh Cairns’ mobile neurosurgical units. The Korean War also saw improvement with the advent of early evacuation of head injury patients. It was not until after the Vietnam War that aggressive pursuit of all bone and shrapnel fragments came into question. Results of the Vietnam Head Injury Study, as well as data from the Lebanon conflict,4 indicated that there is no added benefit in removing deep-seated metallic fragments or bone chips, introducing the doctrine in use today that only accessible fragments should be surgically removed. Current practice continues to develop in the battlefield, as addressed further later in this chapter.
Pathophysiology
Primary Injury
The injury sustained by the brain upon impact with a projectile depends upon four factors: (1) the nature of the projectile (blunt or sharp), (2) the approach of the projectile through the cranium, (3) the kinetic and thermal energy transferred by the projectile, and (4) the susceptibility factors of the victim. The skull has been shown to withstand impacts exceeding 5000 N/cm2 over the frontal bone and more than 2000 N/cm2 over the temporal bone.5 The cranium is often penetrated due to the sharpness and/or the kinetic energy of the foreign body. Bone fragments can act as additional projectiles, causing their own tracts of injury. Kinetic energy (KE) of a projectile is calculated by the following formula:
Here, m represents mass, and V represents velocity. Vinitial represents the velocity immediately before impact. At impact, the skull absorbs most of the energy of the projectile, but the brain parenchyma must absorb the remainder. A penetrating bullet usually causes a narrow, carrot-shaped tract. Higher-velocity projectiles transfer additional energy to the parenchyma in the form of expansile–contractile cavitation waves, leaving wide, turnip-shaped tracts.6 Biophysicists have used high-speed digital video and pressure transducers in brain parenchyma simulant to demonstrate that a 9-mm round (379 m/sec) transfers 6 times the energy and 1.5 times the cavitation volume of a 25-caliber round (238 m/sec). Clinically, the type of round is less important, because the speed of the missile after leaving the weapon rarely informs Vinitial due to unknown friction losses during trajectory.
Secondary Injury
The parenchymal damage mediated by direct laceration and indirect pressure waves, or primary injury, is instantaneous and irreversible. The events that follow—ischemia, neuroinflammation, excitotoxicity, and oxidative stress—combine to create a self-perpetuating cycle of blood–brain barrier (BBB) breakdown, cerebral edema, increased intracranial pressure (ICP), and ultimately decreased cerebral perfusion pressure (CPP) (Fig. 142-2). This is often referred to as secondary injury, and though most of it is thought to occur within the first 12 to 24 hours postinjury, it has been observed to persist for days, possibly weeks.
Animal models have demonstrated the effects of PBI on mean arterial blood pressure, ICP, and CPP. Initially, PBI is followed by a rapid rise in ICP, peaking within a few minutes and decreasing thereafter.7 This is due in part to the reexpansion of the missile cavity by extravasation of blood. In multiple models, CPP was shown to decrease by 50% within the first minute postinjury as measured by intracarotid xenon 133 injections.8 When the pressure waves created by a missile rise sufficiently high, they may pass through the fourth ventricle, affecting the medullary respiratory center and causing apnea. Experiments in the feline model suggest that there is a narrow window between the energy required to penetrate the skull and the level causing fatal apnea,9 indicating that airway protection and rapid management of elevated ICP are crucial.
Extracranial pathology secondary to PBI, especially myocardial dysfunction, coagulopathy,10 and acute lung injury,11 also contributes to hypoxemia and brain ischemia. Therefore, the clinical management of PBI requires a comprehensive approach, as discussed in the next section. The primary aim is to halt or slow the cycle of secondary brain injury in an attempt to preserve the neurovascular unit (neuronal circuit, microvasculature, and astrocyte), with the ultimate goal of preserving cerebral function.
Initial Management: Basic Advanced Trauma Life Support Guidelines
As in all trauma situations, initial management of the patient begins with well-trained prehospital personnel and a trauma team that is experienced in the practice of advanced trauma life support guidelines. In the military, this is of particular importance due to time, safety, and resource limitations in the field of combat. Initial stabilization of the PBI patient requires constant surveillance of the airway, breathing, and circulation, with simultaneous initial neurologic evaluation and assessment for other life- or limb-threatening injuries. Specifically, the Glasgow Coma Scale (GCS)12 is useful for neurologic assessment, making decisions about intubation in the field, and predicting outcomes. The trauma team, including a general surgeon, neurosurgeon, and anesthesiologist, should be notified of information related to the mechanism of injury, vital signs, and initial GCS, as well as a report on interventions performed at the scene and en route to hospital. Careful survey of the body, including nasopharynx and external auditory canals, uncovers hemorrhage, cerebrospinal fluid (CSF) leaks, and additional entrance and exit wounds. Shaving of the scalp may be necessary to expose wounds concealed behind hair clotted with blood. Immediately upon arrival, pulse and blood pressure monitoring, as well as pulse oximetry, should be initiated. If not already done, large-bore intravenous access for colloid or crystalloid volume resuscitation should be established immediately. Simultaneously, arterial blood gases are taken, and venous blood is drawn and sent for complete blood count, electrolytes, glucose, coagulation profile, type, and cross match.
PBI-Specific Medical Management
Intracranial Pressure
All patients with suspected brain injury should have the head of bed elevated to 30 degrees once the cervical spine has been cleared. This allows gravity to facilitate venous and CSF drainage from the cranium. Changes in ICP can occur rapidly, and it is important to perform regular pupillary examinations. If a fixed and dilated pupil is noted and herniation is suspected, osmotic therapy should be initiated immediately in any hemodynamically stable patient. Osmotic agents, first described in a neurosurgical context by Weed and McKibben in 1919,13 create an osmotic gradient that draws free water out of the brain and lowers ICP.
Mannitol is the agent most commonly used, and its proposed mechanisms of action include dehydration of extravascular spaces and augmentation of blood volume while decreasing viscosity, which can lead to increased CPP, cerebral blood flow, and oxygen delivery.14 The diuretic effect of mannitol is efficacious in patients with good renal function and systolic blood pressure of more than 90 mm Hg. Recent retrospective analysis demonstrates a dose-dependent efficacy for mannitol, where every 0.1 g/kg increased over the 50-g dose achieved a 1-mm Hg drop in ICP. The data are still not sufficient to establish a standard dose for mannitol.14
Hypertonic saline (HS) also creates an osmotic gradient to decrease ICP; similarly, it induces a biphasic rheologic and osmotic response in patients. Other proposed mechanisms of ICP reduction with HS include anti-inflammatory activity and downregulation of the molecular water channel, aquaporin-4,15 theoretically decreasing water transport across the BBB. Administration of HS is even less standardized than mannitol. Some studies report the administration of a 3% infusion,16 while others utilize a bolus dose of concentrations ranging from 7% to 23.4%.17 Adverse effects of HS include the possibility of inducing central pontine myelinolysis in patients who are hyponatremic, a tendency to induce a persistent hyperchloremic metabolic acidosis, and the risk of pulmonary edema in patients with underlying cardiac or pulmonary disease.
Hyperventilation has long been known to decrease ICP, likely due to the elevated pH created by eliminating carbonic acid, which causes cerebral arteriole vasoconstriction and decreased cerebral blood volume. This effect is short lived, and vessels have been shown to retrain to a newer, elevated pH. Upon returning the patient to a normal PaCO2, these retrained vessels dilate, thereby elevating ICP even further. Not surprisingly, routine use of hyperventilation was shown to lead to poorer outcomes in randomized trials.18 The Guidelines for the Management of Severe Traumatic Brain Injury recommend avoidance of hyperventilation during the first 24 hours after trauma to preserve cerebral blood flow to at-risk brain tissue. The use of hyperventilation for brief temporizing measures to reduce ICP is acceptable, but it is recommended that brain oxygenation be monitored to reduce the risk of inducing brain tissue hypoxia.
Seizure Prophylaxis
It is reported that 30% to 50% of PBI patients have seizures, and up to 10% of these occur within the first week after injury. PBI is clearly associated with a higher rate of seizures than nonpenetrating TBI.19,20 Therefore, all patients with PBI should receive a loading dose of antiepileptic medications upon arrival to hospital, and maintenance doses should be administered for at least 7 days postinjury. An intravenous bolus of diazepam or lorazepam is used to treat any witnessed seizure. Although the Brain Trauma Foundation guidelines do not recommend prophylaxis beyond 1 week, this recommendation is not based on a study designed to test the efficacy of anticonvulsant therapy specifically in a PBI population, so some authors recommend a longer course of prophylaxis. Phenytoin has long been the first-line drug for short-term seizure prophylaxis.21 Disadvantages of phenytoin include effects on drug metabolism via cytochrome P450 induction, hypersensitivity reactions, and the requirement for serum level monitoring. These have caused some groups to consider alternatives. Since it became available in intravenous form in 2006, levetiracetam has become a popular alternative because it requires no monitoring of drug concentration in the serum.
Infection Prophylaxis
Broad-spectrum antibiotic therapy is recommended in the setting of PBI due to high likelihood of foreign body contamination.22 Although infection with gram-positive organisms is most common, increasing rates of infection with gram-negative organisms, especially Acinetobacter species, is being reported.23 Prophylactic antibiotics were first introduced at the dawn of the penicillin era, during World War II, effectively dropping infection rates from 60% to 6% in PBI patients.24 Current infection rates have been reported to be as low as 1% in the civilian setting, with higher rates of 12% associated with PBI in the military setting.25,26 In addition, tetanus toxin is administered with any foreign body penetration. In recent years, the use of antibiotic-impregnated ventricular catheters has been shown to dramatically decrease the rates of ventriculostomy infections in general neurosurgery patients,27 though no study has been performed specifically in PBI patients.
Imaging and Endovascular Intervention
Upon stabilization of the patient, the initial imaging study is computed tomography (CT). The extent of brain injury, wound tract, presence of pneumocephalus, hematomas, and location of bone chips or foreign bodies can be identified rapidly.4 Today, volume acquisition is the preferred modality.28 Although advances in technology have made computed tomography angiography (CTA) increasingly popular, digital subtraction angiography (DSA) remains the gold standard for detection of traumatic aneurysms, and a recent comparative analysis suggests that CTA only detects half as many vascular injuries as DSA.29 More importantly, DSA allows the opportunity for rapid, less-invasive endovascular intervention with coil occlusion, liquid embolic agents, and stent-buttressed coil embolization to treat traumatic intracranial vessel injuries (Fig. 142-3).
The reported incidence of traumatic intracranial aneurysms (TICAs) varies widely.28 Whether this is due to true variation in incidence or to different rates of lesion detection in various series is unknown. The indications for performing angiography have varied among series. Patients with wound tracts through multiple dural compartments or through faciocranial, orbitocranial, and pterional regions with concomitant hematoma have been reported to have 4 to 10 times the risk of developing an aneurysm (Figs. 142-4 and 142-5).30 However, limiting angiographic studies to these patients may miss important vascular compromise, leading to increased ICP and scarring, making future surgery more difficult, and possibly worsening outcome.31 During Operation Iraqi Freedom and Operation Enduring Freedom, Bell and colleagues33 utilized DSA in all patients with PBI, as well as in those with increased velocities detected by transcranial Doppler ultrasonography. The incidence of TICAs was surprisingly higher than in previous reports: more than 64 of 187 injured combatants (34%) had PBI.
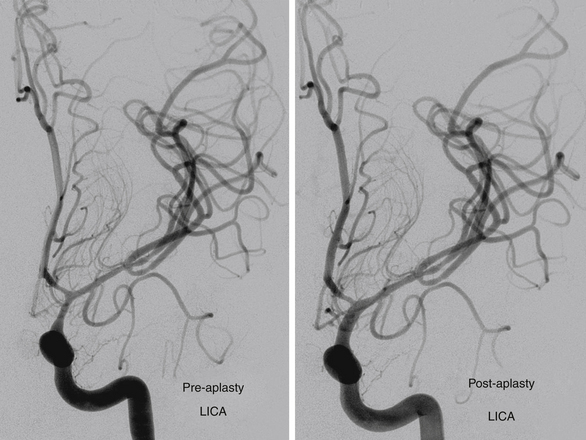
FIGURE 142-4 Vasospasm with associated traumatic psuedoaneurysm enlargement, treated with endovascular stenting.
Traumatic cerebral vasospasm (TCV) is another important complication of PBI that is a major cause of delayed ischemia.32 A recent case series demonstrates TCV occurring in more than half of combatants suffering PBI during Operations Iraqi and Enduring Freedom.33 Treatment of this condition remains controversial, and current modalities include medical management with hypertension, hemodilution, and hypervolemia, as well as systemic or intra-arterial nicardipine or intra-arterial papaverine.33 As the field of neuroendovascular therapy continues to expand, further studies will greatly enhance our understanding of these pathologies and our ability to intervene.
Surgical Management
All patients with PBI should receive aggressive initial resuscitation and imaging evaluation by CT scan. When stabilization and imaging are complete, decisions concerning further therapy often take into account the patient’s neurologic status as determined by the GCS score. Poor survival and outcome are reported in patients with GCS scores between 3 and 5 points.34,35 Most neurosurgeons agree that a patient with a postresuscitation GCS score of 3 points with two dilated nonreactive pupils but without a mass lesion on CT should not receive surgical intervention.36 It has long been known that bihemispheric and transventricular injuries have poor prognosis (Fig. 142-6).37 Kim et al. used vector analysis on bullet trajectories in a series of penetrating head injuries, identifying other anatomic regions that correlated with fatal outcome. This fatal region, or zona fatalis,