Chapter 223 Management of Cervical Disc Herniation
Dorsal Laminoforaminotomy plus Discectomy
Cervical spondylosis is one of the most common pathologies seen by spine surgeons.1,2 Patients with cervical spondylosis can present with pure mechanical neck pain, arm pain that can be traced back to a specific nerve root distribution, signs of spinal cord dysfunction, or often a combination of these factors. On the basis of the location of the degenerative process, the clinical diagnosis accompanying cervical disc disease can be broadly categorized as arthritic neck pain, radiculopathy, myelopathy, or myeloradiculopathy. It is imperative to classify the patient into one of these four diagnoses before selecting the appropriate nonoperative and operative treatment.3 The sole focus for this chapter is the diagnosis and treatment of cervical radiculopathy.
The diagnosis of isolated cervical radiculopathy is established when a patient complains of arm pain that surpasses neck pain. This may or may not be accompanied by motor loss, sensory dysfunction, or an alteration of the reflex arc associated with a particular nerve root. This symptom complex can be caused by a dorsal and lateral disc herniation or a degenerative cascade that results in uncovertebral spurring, ligamentum hypertrophy, and facet arthrosis, leading to cervical neural foraminal stenosis. The natural history of cervical radiculopathy is distinctly separate and different from cervical spondylotic myelopathy.4 Lees and Turner performed a long-term follow-up on patients with cervical spondylosis and reported that 30% of these individuals experienced intermittent radiculitis, whereas about a quarter had persistent radiculopathy.5 As a general principle, conservative care can quell symptoms of cervical radiculopathy in the short term, but in the long run, symptoms frequently recur. Gore et al. conducted a long-term retrospective review on patients with cervical radiculopathy and reported that with conservative care, 79% had a significant decrease in pain, and 43% were free of pain. However, they noted that 50% continued to have persistent symptoms, and approximately 32% had moderate or severe residual pain at 15-year follow-up.6
Unyielding radiculopathy that is recalcitrant to nonoperative management such as nonsteroidal anti-inflammatory drugs, physical therapy, and epidural steroids can be treated surgically. Patients with isolated radiculopathy can be treated with either a ventral or a dorsal decompression as dictated by their pathology. The approach of choice has been a subject of debate for the last 70 years. Semmes and Murphy first described this pathology in 1943. They delineated the compression of the seventh cervical nerve root at the foramen by a unilateral rupture of the C6-7 disc.7 Around the same time, Spurling and Scoville8,9 and separately Frykholm10 pioneered the technique of dorsal foraminal decompression. Within 10 years, Smith and Robinson described the ventral cervical discectomy and interbody fusion using autograft.11 This procedure was revised by Cloward in 1958, with disc excision, removal of compressive structures, and bone dowel grafts for fusion.12 Bailey and Badgley,13 along with Cloward12 and Smith and Robinson,11 helped to establish the ventral cervical discectomy and fusion as the gold standard for degenerative cervical disc disease. Although the ventral approach is associated with high patient satisfaction rates and short recovery times, it does have a greater potential for serious complications.14 Conversely, the dorsal approach completely sidesteps major complications such as recurrent laryngeal nerve injuries and esophageal, tracheal, or vascular injuries. Furthermore, it avoids graft- and fusion-related issues.15
The problem of adjacent-segment degeneration and disease following spine procedures remains a controversial topic. In a large retrospective series, Henderson et al. reported an overall prevalence of 9% with annual incidence of 3% for the development of adjacent-level degeneration in their patients undergoing dorsal cervical foraminotomy.16 Hilibrand et al., in their analysis of 383 patients with ventral surgery, showed that 25.6% of the patients who had a ventral arthrodesis would have new disease at an adjacent level within 10 years after the operation with a relatively constant incidence of 2.9%.17 However, Clarke et al. analyzed 303 patients, using a survivorship analysis similar to that used by Hilibrand, and showed that 6.7% of patients who had a dorsal cervical foraminotomy would have new disease at an adjacent level within 10 years after the operation with a constant incidence of 0.7%.18 These clinical observations suggest that dorsal cervical foraminotomy has a reduced rate of adjacent-segment degeneration compared with anterior cervical discectomy and fusion. Dorsal cervical foraminotomy is a motion-preserving operation. It may avoid increasing loads on the adjacent levels, unlike cervical fusion surgery.
Indications
The current spine literature supports the dorsal laminoforaminotomy approach to the cervical spine for several disorders. The most common indication is persistent and recalcitrant shoulder and arm pain or numbness with or without focal weakness. The ideal candidate should exhibit a specific dermatomal pattern that is correlated and confirmed with radiographic analysis. The typical pathology includes dorsolateral disc herniations and single-level or multilevel spondylotic foraminal stenosis without central canal compromise. Unremitting cervical radiculopathy after ventral cervical discectomy and fusion is another indication.19 The efficacy of dorsal laminoforaminotomy with or without discectomy for lateral recess or foraminal stenosis has been well documented over the last several decades.10,16,20,21 Most studies show that outcomes for axial neck pain are inferior to those for radiculopathy. The success for radicular symptoms is 90% to 95% improvement versus the 70% to 85% for axial neck pain.
The high rate of clinical success can be truly appreciated by analyzing the anatomic constraints of the intervertebral foramina. The neuroforamen is a funnel-shaped structure that is bordered by the uncovertebral joint ventrally and dorsally by the facet joint, specifically the superior articular process. The nerve root can be compressed ventrally by the disc or the osteophytes at the uncovertebral joints of Luschka. In contrast, dorsally the superior articular process or hypertrophic ligamentum flavum causes nerve root compression. Raynor et al. defined this anatomic relationship and delineated that a dorsal decompression allows 3 to 5 mm of the cervical nerve root to be visualized, whereas only 1 to 2 mm of the root can be freed up ventrally.22,23
However, dorsal cervical foraminotomy must be used discreetly, the primary indications being a unilateral dorsolateral disc herniation, facet arthritis with compression, uncovertebral osteophytes, or ligamentum flavum thickening. This procedure would be contraindicated for cases of myelopathy due to central or paracentral stenosis and for cases of deformity or instability.24 Spondylosis with a kyphotic deformity or instability, bilateral symptoms or a broad-based central disc bulge should be managed directly with a ventral decompression and fusion or a dorsal decompression (laminectomy or laminoplasty) with or without a fusion. In certain instances, a combined ventral and dorsal procedure may be the most appropriate.21,25
Surgical Technique
Frykholm was the first to describe the dorsal foraminotomy in 1951.26 The surgical techniques for this procedure have evolved tremendously over the last several decades. Initially, this surgery was performed as part of a multilevel laminectomy in the seated position.25 With surgical innovation and microscopic techniques, a less invasive keyhole foraminotomy was developed, and most recently, a microendoscopic laminoforaminotomy through a minimally invasive approach has been described.27
Open Laminoforaminotomy
After endotracheal intubation, the patient’s head is placed in a three-point Mayfield pinion, and the patient is turned prone onto gel-filled rolls for chest bolsters on a standard operating room table. The patient’s arms are tucked at the patient’s side, and any pressure points around the elbow, wrist, and hand are padded. Gentle inferior traction applied on the shoulders will allow visualization of the lower vertebrae in the cervical spine with lateral fluoroscopy or radiographs. Using a shoulder harness, taping the shoulders downward, using long Kerlex gauze wrapped around each wrist for traction, or alternatively pushing each shoulder downward with an empty suction canister is a useful and viable method for inferior traction on the shoulders for improved intraoperative radiographic localization. Excessive or prolonged force on the shoulders can cause injury to the brachial plexus and should be avoided. The knees are flexed, a foot rest is slid to position under the knees, and pillows are used to pad this pressure point. A buttock strap is placed behind the patient, and then the bed can be brought into a reverse Trendelenburg position. The buttock strap and foot rest under the knees prevent the patient from sliding down as the table is tilted. Tilting the table will obtain a parallel relationship of the neck to the floor, improve venous return, and minimize bleeding (Fig. 223-1).
After the bed has been tilted, it is helpful to recheck the positioning of the neck and place the cervical spine in a small amount of flexion with two to three finger breadths between the chin and the chest in most patients. The neck is then clipped where needed, prepped, and draped in the usual sterile manner. Using fluoroscopy or a lateral radiograph will confirm the correct operative level and minimize the length of the skin incision. Typically, a 3- to 4-cm skin incision is marked in the midline centered on the disc space. After cutting through the skin, the dissection is carried down the midline or slightly ipsilateral to the side of the planned exposure to the level of the spinous process. Staying within the nuchal ligament will avoid any unnecessary muscle trauma and minimize bleeding. The deep dissection after identification of the spinous process is then performed unilaterally and subperiosteally, the paraspinal muscles being stripped only on the symptomatic side. The paraspinal muscles insert on the inferior edge of the hemilamina, and division of the muscle at this location will allow the subperiosteal dissection to show the area of interest. The inferior aspect of the superior lamina and superior aspect of the inferior lamina are exposed by this process. After the spinous process and the lamina are exposed, another localizing radiograph is preformed to ensure the appropriate level. The exposure is carried laterally to expose the medial aspect of the facet joint, and a self-retaining retractor is placed. Removal of the medial 20% to 30% of the facet capsule will expose the lateral limit of the foraminotomy. Take heed that removal of more than 50% of the facet capsule can destabilize the spine.
Under microscopic or loop magnification, a high-speed burr is used to perform the foraminotomy. A 3- to 4-mm diamond burr will minimize bleeding during this step, although a small round- or acorn-tipped cutting burr on a long bit such as the Midas Rex AM-8 bit is also appropriate. A long bit and a small cutting or diamond burr will optimize visualization during the drilling process. The keyhole foraminotomy begins at the lamina-facet junction, with careful consideration of the amount of facet resection. Typically, only the medial one third is drilled. Then a 1- or 2-mm Kerrison punch can be carefully placed over the nerve root and then used to undercut the facet, ensuring that the spine is not destabilized by the foraminotomy. The amount of facet resection must not exceed 50% in order to preserve spine stability.28
Microendoscopic Laminoforaminotomy
The same standard setup is utilized to position the patient as was described in the section on open foraminotomy. Lateral fluoroscopy is brought into the field for this procedure, and it is often helpful to utilize gentle traction on the shoulders to visualize the lower cervical vertebra radiographically. The image should be matched to the anatomic position of the patient. A Steinman pin maybe used lateral to the neck to give a true dorsal and ventral reference and to help determine the angle of the lamina and disc space (see Fig. 223-1). The neck is then clipped where needed, prepped, and draped in the usual sterile manner.
After fluoroscopic confirmation of the correct operative level, a 2-cm incision is made 1 to 2 cm lateral to the spinous process. Avoid using a K-wire or a Steinman pin, as it may pass between the lamina and injure the dura, spinal cord, or nerve root. The fascia may be opened by a pair of Metzenbaum scissors or Bovie electrocautery so that dilators can be easily passed to the desired location. The smallest dilator can be used to palpate the inferior and superior lamina to identify this necessary landmark. This step also allows the dissection of the paraspinal muscles at the surgical field without resecting them (Fig. 223-2). Avoid excessive downward force in the interlaminar window with the dilator, as it could cause injury to the spinal cord or nerve root. After the smallest dilator is positioned over the appropriate disc space, serial dilation is performed to widen the opening and allow the tubular retraction system to be docked into place on the facet complex. A 14- to 18-mm-diameter tube is adequate for a single-level foraminotomy. Using the smallest beveled tube will allow strategic retractor placement on the lamina to avoid excessive muscle creep into the operative field. The tube is held in place by a retractor arm attached to the contralateral side of the bed, allowing the assistant to tighten the retractor arm while the surgeon holds the tube in position. The final retractor location should be verified via fluoroscopy to ensure the correct level.
The operative microscope or an endoscope can be utilized to provide adequate visualization of the operative field through the tubular retractor. If muscle encroachment continues to be an issue, a redilation of the muscles through the final retractor system may be performed, and a smaller tubular retractor may be utilized. Once again, this process can avoid the need for muscle resection for optimal visualization (Fig. 223-3). However, a small circular cuff of muscle may sometimes be removed to identify the lamina-facet junction. The foraminotomy is performed following the same principles and steps as detailed previously with the help of specialized bayoneted long instruments that allow the surgeon to work within the confines of a long, narrow working portal.19,24,27,29 By using these techniques, a soft lateral disc herniation can also be removed with a small incision and minimal exposure, reducing the length of hospital stay, narcotic requirements, and blood loss from the procedure (Fig. 223-4).
Seated Position
The seated position is labor intensive for the operating room staff, and most hospitals are not equipped for or familiar with the setup. More important, additional monitoring is required during the entire length of the procedure for rapid detection and treatment of venous air embolism. The reported incidence of air emboli varies throughout the anesthesia literature depending on the monitoring device utilized. A rate of 7% to 76% has been described in the literature. The incidence is about 7% to 25% for cervical laminectomies and 76% for posterior fossa surgery.30–32 The typical monitoring methods include precordial Doppler and the measurement of end-expired carbon dioxide and nitrogen with a mass spectrometer. Transesophageal echocardiography is a more sensitive monitor and may be utilized for higher-risk cases. A central venous catheter is also placed with its tip in the upper right atrium so that any air entering the venous system can be aspirated promptly.
If air embolism is detected, the surgeon should immediately attempt to locate and eliminate the site of air embolism. Flooding the field with saline and/or packing the wound with wet sponges may eliminate air embolism. Bone wax should be placed over any cut bleeding bone. Jugular venous pressure will minimize air entry and may aid the surgeon in localizing open veins at the site of increased bleeding. In addition to aspiration of air via a central venous catheter, the anesthesiologist should stop nitrous oxide, and the patient should be placed on 100% oxygen. Nitrous oxide may increase the size of the air embolus.33 In the event of cardiovascular collapse, the operative site should immediately be lowered below the level of the heart. Furthermore, the left lateral decubitus (Durant) position may be utilized to release an air lock in the right side of the heart.34
Complications
Dorsal cervical foraminotomy, with or without discectomy, is a safe procedure associated with a very low complication rate (0–10%).35 However, potential complications can range from a simple stitch abscess to a life-threatening air embolism or severe spinal cord injury. Excessive bleeding is noted more commonly in the prone position, while air emboli, pneumocephaly, and cord and brain ischemia are cited in the sitting position.
Intraoperative air embolism can be a lethal event.36 It is essential for a surgeon using the seated position to be well versed in the prevention as well as rapid detection and management of air emboli. Lower-extremity compression stockings and adequate preoperative hydration will increase right atrial pressure and reduce the negative pressure gradient of the surgical field. The key step in preventing air embolism is prompt surgical hemostasis, minimizing the exposure of the open venous system to the atmospheric pressure.
Another possible complication is a dural tear. Incidental durotomies are noted with this procedure at a rate of 1% to 3% with the open procedure, which is no different than the ventral approach. However, the rate of durotomy with the minimally invasive endoscopic approach has been reported to be as high as 8% in the early surgical experience and then levels out to a rate less than 3%.27 Wound infections are much lower compared to other dorsal cervical surgeries, likely due to the limited incision and minimal muscle retraction and ischemia.
The most common neurologic complication is a transient nerve root palsy, the majority of which resolve within a week.20 The most common nerve root affected is at the C5 level. The mechanism of action is thought to be a revascularization-related transitory ischemia and a traction injury after decompression of an inflamed nerve root. Transient nerve root palsy may be more common when two or more levels are preformed. Nerve root and spinal cord injury are reported but very rare. Recurrence of symptoms are reported in a small number of cases and are more common with long-term follow-up of patients with significant spondylosis.37–39
Finally, instability of the cervical spine can result from excessive resection of the facet joint. Several studies suggest that instability results only if more than 50% of the facet or facet capsule is resected,40–42 and that is usually unnecessary for an adequate decompression.
Outcomes
The effectiveness of dorsal cervical foraminotomy with or without discectomy is consistently documented favorably with the appropriately selected patients. In general, the outcomes are reported to be successful in more than 90% of the cases with a 70% to 100% range of success.43,44 Henderson et al. reported their experience of 846 cervical foraminotomies for hard and soft disc disease in 736 patients. They documented a 96% incidence of relief in regard to arm pain and parasthesias and a 98% improvement of the preoperative motor weakness.16 They delineated similar results for soft and hard disc protrusions, including purely spondylotic radiculopathy. Krupp et al. reported a good to excellent result in 98% of the 161 patients analyzed after dorsal cervical foraminotomies. They determined a slight difference between hard and soft disc pathology, with favorable outcomes of 98%, 84%, and 91% for soft, mixed, and hard discs, respectively. However, this study had a large loss to follow-up with an initial population of 230 patients.45 Herkowitz et al. prospectively compared the ventral and the dorsal approaches to address the soft disc radiculopathy and demonstrated a 75% good or excellent outcome in 16 patients randomized for the dorsal cervical foraminotomy.39 Silveri et al., in a retrospective study of 84 patients at a mean follow-up of 6 years, reported a 98% good to excellent result.46 Jodicke et al. report a 94% good to excellent result at 6 weeks post dorsal foraminotomy and an 85% good or excellent outcome at a mean of 33 months. According to their study, soft disc pathology had a higher ratio of good outcomes as compared to hard disc disease at early follow-up; however, at long term, there was no statistical difference between the two. A 15% recurrence rate was reported.47 More recently, Jagannathan et al. performed a retrospective review on 162 cases of a single-level unilateral dorsal foraminotomy by a single surgeon with a 5-year minimum follow-up. They reported a significant neck disability index improvement in 93% of the patients and a resolution of radiculopathy in 95% of the patients. They also reported no statistically significant change in focal or segmental kyphosis in their cohort. However, they did delineate certain risk factors associated with worsening sagittal alignment: age greater than 60 years, preoperative cervical lordosis less than10 degrees, and a previous dorsal surgery.48
Postoperative cervical muscular pain and spasms resulting from ischemia during subperiosteal dissection of the paraspinal muscles are the major disadvantages of the standard open approach. This has resulted in the advent of minimally invasive microendoscopic approaches to dorsal nerve root decompression. Roh et al., through a cadaveric study, showed that the average vertical and transverse diameters of the laminoforaminotomy defects were similar between the open and minimally invasive techniques. Furthermore, the length of the nerve root that was decompressed and the average portion of the facet that was removed were not statistically different in the two groups.19 Fessler and Khoo reported their clinical data on minimally invasive cervical microendoscopic foraminotomy (MEF). They performed a traditional open dorsal foraminotomy on 26 patients and compared it to the MEF technique in 25 patients. They reported that the MEF group had a lower operative time (115 minutes vs. 171 minutes), decreased blood loss (138 mL vs. 246 mL), shorter hospitalization (20 hours vs. 68 hours), and less narcotic use when compared with patients who underwent the open procedure. Both the open and MEF groups had greater than 90% improvement of symptoms and were statistically similar in outcome.24
Adamson reported the results of 100 consecutive cases of microendoscopic laminoforaminotomy for the treatment of unilateral cervical radiculopathy secondary to disc herniation or foraminal stenosis. In this study, 97% of the patients had good or excellent results, and 100% returned to their preoperative occupation at baseline activity between 1 day and 4 weeks postoperatively. Ninety percent were done as same-day procedures, and 10% of patients were discharged on postoperative day 1.27
Summary
Multiple procedures allow access to the spinal canal and/or neural foramen. Each procedure has distinct advantages and disadvantages. The dorsal laminoforaminotomy with or without discectomy is historically a very successful surgery. It avoids potentially serious complications such as esophageal and vascular injuries, as well as fusion problems associated with ventral surgery. The dorsal approach provides the simplest and the most direct exposure to single-level or multilevel nerve root pathology without requiring instrumentation and fusion, and it does not accelerate spondylotic degeneration of adjacent levels.18 The decompression does not require significant facet resection to affect the spine stability, and there is no postoperative immobilization. Careful patient selection with good surgical technique results in 95% improvement of preoperative symptoms. The optimal surgical candidate has a lateral soft disc or focal osteophyte pathology causing a unilateral medically refractory radiculopathy in the absence of kyphosis or spine instability.
Adamson T.E. Microendoscopic posterior cervical laminoforaminotomy for unilateral radiculopathy: results of a new technique in 100 cases. J Neurosurg. 2001;95:51-57.
Ducker T.B., Zeidman S.M. The posterior operative approach for cervical radiculopathy. Neurosurg Clin N Am. 1993;4:61-74.
Fessler R.G., Khoo L.T. Minimally invasive cervical microendoscopic foraminotomy: an initial clinical experience. Neurosurgery. 2002;51:S37-S45.
Frykholm R. Deformities of dural pouches and strictures of dural sheaths in the cervical region producing nerve root compression. J Neurosurg. 1947:403-413.
Gore D.R., Sepic S.B., Gardner G.M., et al. Neck pain: a long-term follow-up of 205 patients. Spine (Phila Pa 1976). 1987;12:1-5.
Henderson C.M., Hennessy R.G., Shuey H.M.Jr., et al. Posterior-lateral foraminotomy as an exclusive operative technique for cervical radiculopathy: a review of 846 consecutively operated cases. Neurosurgery. 1983;13:504-512.
Jagannathan J., Sherman J.H., Szabo T., et al. The posterior cervical foraminotomy in the treatment of cervical disc/osteophyte disease: a single-surgeon experience with a minimum of 5 years’ clinical and radiographic follow-up. J Neurosurg Spine. 2009;10:347-356.
1. Adams C.B., Logue V. Studies in cervical spondylotic myelopathy. I. movement of the cervical roots, dura and cord, and their relation to the course of the extrathecal roots. Brain. 1971;94:557-568.
2. Bohlman H.H., Emery S.E. The pathophysiology of cervical spondylosis and myelopathy. Spine (Phila Pa 1976). 1988;13:843-846.
3. Ducker T.B., Zeidman S.M. The posterior operative approach for cervical radiculopathy. Neurosurg Clin N Am. 1993;4:61-74.
4. Dillin W., Booth R., Cuckler J., et al. Cervical radiculopathy. A review. Spine (Phila Pa 1976). 1986;11:988-991.
5. Lees F., Turner J.W. Natural history and prognosis of cervical spondylosis. Br Med J. 1963;2:1607-1610.
6. Gore D.R., Sepic S.B., Gardner G.M., et al. Neck pain: a long-term follow-up of 205 patients. Spine (Phila Pa 1976). 1987;12:1-5.
7. Semmes R.E., Murphy F. The syndrome of unilateral rupture of the sixth cervical intervertebral disc. JAMA. 1943:1209-1214.
8. Scoville W.B. Rupture of the lateral cervical disk and its operative technique. Boston: Proceedings of the Harvey Cushing Meeting; 1946.
9. Spurling R., Scoville W.B. Lateral rupture of the cervical intervertebral discs: a common cause of shoulder and arm pain. Surg Gynae Obst. 1944:350-358.
10. Frykholm R. Deformities of dural pouches and strictures of dural sheaths in the cervical region producing nerve root compression. J Neurosurg. 1947:403-413.
11. Smith G.W., Robinson R.A. The treatment of certain cervical-spine disorders by anterior removal of the intervertebral disc and interbody fusion. J Bone Joint Surg [Am]. 1958;40:607-624.
12. Cloward R.B. The anterior approach for removal of ruptured cervical disks. J Neurosurg. 1958;15:602-617.
13. Bailey R.W., Badgley C.E. Stabilization of the cervical spine by anterior fusion. J Bone Joint Surg [Am]. 1960;42:565-594.
14. Graham J.J. Complications of cervical spine surgery. A five-year report on a survey of the membership of the cervical spine research society by the morbidity and mortality committee. Spine (Phila Pa 1976). 1989;14:1046-1050.
15. Cloward R.B. Complications of anterior cervical disc operation and their treatment. Surgery. 1971;69:175-182.
16. Henderson C.M., Hennessy R.G., Shuey H.M.Jr., et al. Posterior-lateral foraminotomy as an exclusive operative technique for cervical radiculopathy: a review of 846 consecutively operated cases. Neurosurgery. 1983;13:504-512.
17. Hilibrand A.S., Carlson G.D., Palumbo M.A., et al. Radiculopathy and myelopathy at segments adjacent to the site of a previous anterior cervical arthrodesis. J Bone Joint Surg [Am]. 1999;81:519-528.
18. Clarke M.J., Ecker R.D., Krauss W.E., et al. Same-segment and adjacent-segment disease following posterior cervical foraminotomy. J Neurosurg Spine. 2007;6:5-9.
19. Roh S.W., Kim D.H., Cardoso A.C., et al. Endoscopic foraminotomy using MED system in cadaveric specimens. Spine (Phila Pa 1976). 2000;25:260-264.
20. Zeidman S.M., Ducker T.B. Posterior cervical laminoforaminotomy for radiculopathy: review of 172 cases. Neurosurgery. 1993;33:356-362.
21. Epstein N.E. A review of laminoforaminotomy for the management of lateral and foraminal cervical disc herniations or spurs. Surg Neurol. 2002;57:226-233. discussion 233-4
22. Russell S.M., Benjamin V. Posterior surgical approach to the cervical neural foramen for intervertebral disc disease. Neurosurgery. 2004;54:662-665. discussion 665–666
23. Raynor R.B., Pugh J., Shapiro I. Cervical facetectomy and its effect on spine strength. J Neurosurg. 1985;63:278-282.
24. Fessler R.G., Khoo L.T. Minimally invasive cervical microendoscopic foraminotomy: an initial clinical experience. Neurosurgery. 2002;51:S37-S45.
25. Scoville W.B., Dohrmann G.J., Corkill G. Late results of cervical disc surgery. J Neurosurg. 1976;45:203-210.
26. Frykholm R. Cervical nerve root compression resulting from disk degeneration and root sleeve fibrosis. Acta Chir Scand. 1951;160:1-149.
27. Adamson T.E. Microendoscopic posterior cervical laminoforaminotomy for unilateral radiculopathy: results of a new technique in 100 cases. J Neurosurg. 2001;95:51-57.
28. Grundy P.L., Germon T.J., Gill S.S. Transpedicular approaches to cervical uncovertebral osteophytes causing radiculopathy. J Neurosurg. 2000;93:21-27.
29. Santiago P., Fessler R.G. Minimally invasive surgery for the management of cervical spondylosis. Neurosurgery. 2007;60:S160-S165.
30. Papadopoulos G., Kuhly P., Brock M., et al. Venous and paradoxical air embolism in the sitting position. A prospective study with transoesophageal echocardiography. Acta Neurochir (Wien). 1994;126:140-143.
31. Losasso T.J., Black S., Muzzi D.A., et al. Detection and hemodynamic consequences of venous air embolism. Does nitrous oxide make a difference? Anesthesiology. 1992;77:148-152.
32. Porter J.M., Pidgeon C., Cunningham A.J. The sitting position in neurosurgery: a critical appraisal. Br J Anaesth. 1999;82:117-128.
33. Munson E.S., Merrick H.C. Effect of nitrous oxide on venous air embolism. Anesthesiology. 1966;27:783-787.
34. Durant T.M., Long J., Oppenheimer M.J. Gas embolism. Proc Am Fed Clin Res. 1947;3:43.
35. Williams R.W. Microcervical foraminotomy. A surgical alternative for intractable radicular pain. Spine (Phila Pa 1976). 1983;8:708-716.
36. Flanagan J.P., Gradisar I.A., Gross R.J., et al. Air embolus—a lethal complication of subclavian venipuncture. N Engl J Med. 1969;281:488-489.
37. Woertgen C., Holzschuh M., Rothoerl R.D., et al. Prognostic factors of posterior cervical disc surgery: a prospective, consecutive study of 54 patients. Neurosurgery. 1997;40:724-728. discussion 728–729
38. Woertgen C., Rothoerl R.D., Henkel J., et al. Long term outcome after cervical foraminotomy. J Clin Neurosci. 2000;7:312-315.
39. Herkowitz H.N., Kurz L.T., Overholt D.P. Surgical management of cervical soft disc herniation. A comparison between the anterior and posterior approach. Spine (Phila Pa 1976). 1990;15:1026-1030.
40. Harrop J.S., Silva M.T., Sharan A.D., et al. Cervicothoracic radiculopathy treated using posterior cervical foraminotomy/discectomy. J Neurosurg. 2003;98:131-136.
41. Grieve J.P., Kitchen N.D., Moore A.J., et al. Results of posterior cervical foraminotomy for treatment of cervical spondylitic radiculopathy. Br J Neurosurg. 2000;14:40-43.
42. Zdeblick T.A., Zou D., Warden K.E., et al. Cervical stability after foraminotomy. A biomechanical in vitro analysis. J Bone Joint Surg [Am]. 1992;74:22-27.
43. Murphey F., Simmons J.C. Ruptured cervical disc. Experience with 250 cases. Am Surg. 1966;32:83-88.
44. Murphey F., Simmons J.C., Brunson B. Surgical treatment of laterally ruptured cervical disc. Review of 648 cases, 1939 to 1972. J Neurosurg. 1973;38:679-683.
45. Krupp W., Schattke H., Muke R. Clinical results of the foraminotomy as described by Frykholm for the treatment of lateral cervical disc herniation. Acta Neurochir (Wien). 1990;107:22-29.
46. Silveri C.P., Simpson J.M., Simeone F.A., et al. Cervical disk disease and the keyhole foraminotomy: proven efficacy at extended long-term follow up. Orthopedics. 1997;20:687-692.
47. Jodicke A., Daentzer D., Kastner S., et al. Risk factors for outcome and complications of dorsal foraminotomy in cervical disc herniation. Surg Neurol. 2003;60:124-129. discussion 129–130
48. Jagannathan J., Sherman J.H., Szabo T., et al. The posterior cervical foraminotomy in the treatment of cervical disc/osteophyte disease: a single-surgeon experience with a minimum of 5 years’ clinical and radiographic follow-up. J Neurosurg Spine. 2009;10:347-356.
Ventral Cervical Discectomy and Fusion with Allograft or Bone Morphogenetic Protein and Plating
Since the introduction of the ventral cervical discectomy and fusion for the treatment of cervical disc disease by Cloward, Smith, and Robinson1–3 the technique has undergone continual refinement, incorporating the advances in plating and allografts and the recent developments in osteobiologics. Anterior cervical approaches remain one of the most popular and successful operative approaches to treat cervical spine disorders. Because of the simplicity and elegance of the procedure and its overall versatility and success in treating ventral cervical pathologies, every spine surgeon should be comfortable with the surgical anatomy and operative techniques of this operation. With the vast array of plating systems, the numerous allograft alternatives, and the introduction of bone morphogenetic proteins, a sophisticated understanding of these options by the surgeons facilitates their selection and safe implementation. This chapter provides a description of operative techniques for ventral cervical discectomy and then reviews the alternatives to autograft, including the various options for allograft and the use of bone morphogenetic protein. Finally, the principles of cervical plating are reviewed.
Preoperative Decision Making
The surgeon should determine the graft type before surgery. This decision depends on patient preference, nature of the pathology, patient compliance, and risk factors for pseudarthrosis. Radiographs should be examined to confirm the level of pathology, the extent of spinal cord compression, the presence or absence of kyphosis, bone quality, presence of spondylolisthesis, or instability. The surgeon should preoperatively select the most appropriate bone graft and plating system for the patient. Most implant systems are user friendly, and there do not appear to be significant differences in single-level fusion rates between the older plating systems and the newer dynamic systems.4 For single-level fusions, rigid or hybrid screw-plate systems suffice for most purposes. While a variety of plating systems are currently available, the basic principles of graft loading and screw-plate interface are the same in all of them. These principles are reviewed in the following sections.
Operative Technique
Patients are brought into the operating room and placed supine on the operating table. After induction of general anesthesia, a bag of intravenous fluid wrapped in a surgical towel is placed under the neck to restore lordosis (Fig. 223-5). The head may be placed on a doughnut. Esophageal stethoscopes and nasogastric tubes are avoided, since they can aggravate esophageal injury by retracting against rigid objects. Various anatomic landmarks may be palpated to estimate the level of the disc space. The hyoid bone is generally at C3, the thyroid cartilage is at C5, and the cricoid cartilage is at C6. The carotid tubercle, the most reliable landmark, can be palpated at C6. In the absence of reliable anatomic landmarks, a preoperative lateral cervical radiograph may be used to determine location of the incision. Once the incision has been planned, the ventral neck region is prepped and draped in the usual sterile fashion.
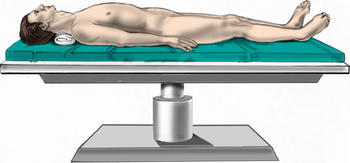
FIGURE 223-5 Patient positioning. The patient is positioned supine. A roll is placed under the neck to restore cervical lordosis.
The incision for a single- or two-level discectomy generally follows one of the neck creases. The incision should extend from midline to the medial border of the sternocleidomastoid muscle (SCM) (Fig. 223-6). Oblique incisions along the medial border of the SCM may be used if access to three or more levels is desired. The right side is generally preferred for a right-handed surgeon. There is not an increased incidence of recurrent laryngeal nerve palsies with a right-sided approach compared with a left-sided approach.5
After the incision is made with a no. 10 blade, Bovie electrocautery or Metzenbaum scissors can be used to traverse the subcutaneous tissues to access the platysma muscle. Undermining the platysma muscle is critical to aid in retraction. After the platysma muscle has been divided and undermined, the medial border of the SCM is identified. A plane is gently developed along the medial border of the SCM using superficial and sharp dissection. The carotid sheath is identified, and its contents are retracted laterally as the spine is bluntly palpated. The omohyoid muscle at C5-6 may be divided if necessary to improve access and exposure. The prevertebral fascia is divided in the middle of the vertebral body and swept off the spine by using a Kittner (Sheavor). Once the level has been reached, a localizing radiograph is obtained to confirm the operative disc space level. The use of a spine needle in the disc space may be helpful for this. The surgeon also may place an initial vertebral distraction pin into a body to aid with screw length determination. Once the level has been determined, optimal exposure is obtained by cauterizing the edges of the longus colli muscle to gain access to the uncovertebral joints (Fig. 223-7). This also aids in the identification of midline.
The longus colli muscle can be released with the Bovie or bipolar electrocautery. Bovie electrocautery should be used judiciously below C6 to avoid inadvertent thermal injury to the recurrent laryngeal nerve. No attempt during the exposure is made to visualize the recurrent laryngeal nerve. Self-retaining retractors are placed, with small teeth under the medial longus colli muscle (Fig. 223-8). Larger-toothed retractors can be used, but care should be taken to ensure that the teeth insert well under the longus colli. Once the self-retaining retractors have been placed beneath the longus colli, the surgeon may request the cuff of the endotracheal tube to be deflated completely and reinflated only to prevent an air leak. If further exposure is desired, longitudinal blunt self-retaining retractors can be placed in a rostrocaudal fashion, as shown in Fig. 223-9.
A Cushing rongeur and a quarter-inch key elevator can be used to remove ventral osteophytes and to “garden” the vertebral bodies of interest (Fig. 223-10; see also Fig. 223-9). Vertebral body gardening further exposes the ventral disc and prepares the vertebrae for plating. Next, distraction posts (Caspar pins) are placed into the vertebral body, which will allow the graft to be placed under distraction. These posts may be angled to reduce a kyphosis (Fig. 223-11). If radiographs are taken, the length of the distraction pins can be used as a guide to select the appropriate screw length. A no. 15 or no. 11 blade is used to incise the annulus and anterior disc (Fig. 223-12).
The disc is then removed to the level of the posterior longitudinal ligament (PLL) using curettes. The spine segment is expanded by applying distraction across the disc space as the disc is removed (Fig. 223-13). The PLL and disc space are usually obscured by ventral and dorsal osteophytes (Figs. 223-14 and 223-15). As the inferior aspect of the vertebral bodies are naturally scalloped, the ventral lip of the superior vertebral body may be drilled to improve visualization of the disc space and prepare for graft insertion (Fig. 223-16). Dorsal osteophytes and end plates may be drilled to access the PLL (Fig. 223-17). The drilling of the end plates widens the disc space and increases access to the PLL (Fig. 223-18). Further drilling of the dorsal edge of the caudal vertebra and undercutting with 3-mm thin-lipped cervical Kerrison rongeurs may be performed to remove osteophytes and further widen the interspace (Figs. 223-19 and 223-20).
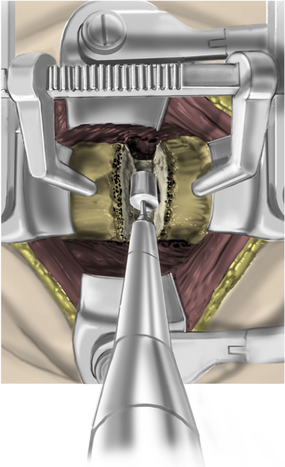
FIGURE 223-17 Drilling dorsal osteophytes. Drilling dorsal osteophytes facilitates access to the posterior longitudinal ligament.
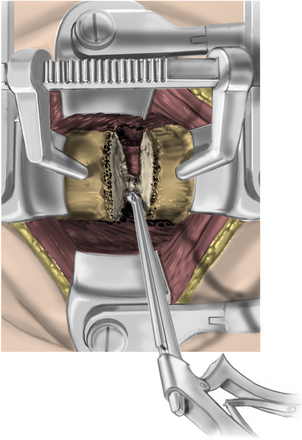
FIGURE 223-19 Drilling the dorsal caudal vertebral body. This widens the interspace and removes osteophytes.
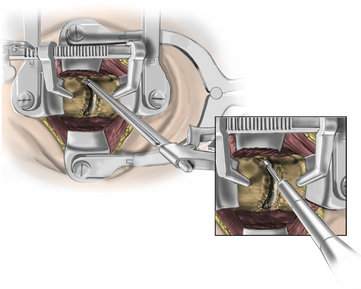
FIGURE 223-20 Undercutting rostral and caudal vertebral bodies with a 3-mm thin-lipped cervical Kerrison rongeur.
Forward- and back-angled curettes may be used to cut the PLL from bone. The PLL is thinner laterally and may be easier to open laterally. The PLL is carefully removed to avoid cerebrospinal fluid leak. A small burr may be used to drill the lateral inferior uncovertebral joint (Fig. 223-21). This permits identification of the pedicle and the nerve root, which is rostral to the pedicle. Foraminotomies are performed as needed, using a 2-mm Kerrison rongeur. It is important to stay on bone to avoid epidural veins. Decompression should extend laterally until direct visualization of the medial aspect of the nerve root is achieved. Following decompression of the disc space and neural elements, the end plates are prepared for graft insertion. It is important to remove the cartilaginous end plate of both vertebral bodies and visualize punctate bleeding from the bone to ensure an optimal environment for arthrodesis. The goal of graft site preparation in using the allograft is to create a combination of cancellous and cortical bone by drilling the ventral caudal edge of the rostral vertebra and dorsal rostral edge of the caudal vertebral body (Fig. 223-22). Cortical bone prevents graft subsidence, while the configuration of cancellous bone enhances fusion. If bone morphogenetic protein is to be used, on the other hand, it is vital to preserve the cortical end plate. Cancellous bone in contact with bone morphogenetic protein (BMP) may result in osteolysis.
Selection of an Interbody Graft
The use of autologous iliac crest bone graft is conceptually ideal for an interbody graft with the cortical bone for structural support and cancellous bone for its osteoinductive and osteoconductive properties.6 However, graft site complications and persistent donor site problems have made this option less appealing.7,8 Therefore, despite its being considered the gold standard, there is a trend away from using autograft. Fortunately, several options are available to the surgeon in lieu of iliac autograft (Fig, 223-23):
• Cadaver tricortical graft has the advantages of cortical bone for structural support with the cancellous matrix for osteoconduction. As it is processed cadaver bone, it has no osteoinductive properties. Since it is not machined, it remains low cost, but it requires the surgeon to shape the graft to size during surgery.
• Machined cadaver cortical allograft is precut to various standard sizes that correspond with the trials in the surgical sets. It has the advantages of cortical bone for structural support but lacks the cancellous structure for osteoconduction, as it is hollow in the middle. This graft may be filled with collected bone shavings or demineralized bone matrix. The grafts are shaped with either parallel or lordotic end plates depending on the type of pathology at hand and the goals of the surgery.
• Machined cadaver cortical cancellous allograft is also precut to various standard sizes. Instead of one cortical ring, this graft is made up of two cortical planks surrounding a cancellous center. The cortical planks provide strength for structural support, while the cancellous bone provides a lattice for an osteoconductive environment but without osteoinductive properties. Again, these are shaped with either parallel or lordotic end plates.
• Bone morphogenetic protein in a polyetheretherketone (PEEK) spacer. (See later discussion.)
Using allograft avoids all graft site complications associated with taking autograft, although fusion rates may be lower with allograft.9–13 Several studies have demonstrated that fusion rates may be significantly augmented with the addition of a cervical plate following graft placement.4,14–18
Bone Morphogenetic Protein
The homodimeric bone morphogenetic protein-2 (BMP-2) is a member of the transforming growth factor beta (TGF-β) superfamily that is known to induce bone formation and regeneration.19,20 When BMP binds one of its two cellular membrane receptors, it sets into motion a complex molecular cascade that will ultimately result in cell migration, proliferation, and differentiation needed for bone formation.20,21 Within days of implantation, the graft site is infiltrated by osteoblasts and undifferentiated mesenchymal cells. After the differentiation of the mesenchymal cells into chondrocytes, cartilage is formed. The differentiated osteoblasts will then begin to lay down bone matrix within the cartilage framework. Finally, mineralization of the new bone occurs as the cartilaginous template resorbs.22
The framework for the formation of new bone is an absorbable type I collagen sponge. After the reconstitution of BMP-2, the sponge is allowed to soak for a minimum of 15 minutes. On the basis of in vitro and in vivo studies 95% of BMP-2 is bound to the sponge after 15 minutes, which increases to only 97% after 120 minutes.23,24 When contained within a nonporous, noncompressible spacer, the collagen sponge releases the bound BMP in the vicinity of the grafting site and has an undetectable concentration 4 weeks after implantation.25 Migrating cells are then drawn into the sponge, where they proliferate and differentiate. This same collagen sponge in turns acts as an osteoconductive scaffold for bone formation before being resorbed and replaced entirely by mature bone.26 Reconstitution of the BMP and preparation of the sponge are critical factors in this process and are reviewed in the following sections.
Role of the Interbody Spacer
While several spacers have been used in combination with BMP-2 in the cervical spine, including allograft, porous, and resorbable spacers, the authors prefer a spacer made from a semicrystalline thermoplastic polymer known as PEEK (Fig. 223-24).27–30 This polymer has a modulus of elasticity between that of cortical and cancellous bone, thus mimicking the load characteristics of the native spine. Because it is radiolucent, radiopaque markers are placed on the anterior and posterior aspects of the spacer. This allows for readily evaluating a bony fusion, which is more difficult with an allograft spacer. Regardless of the spacer utilized with BMP-2, it is our preference that porous or resorbable spacers not be used, given the capacity of unwanted elution of BMP onto surrounding structures.
Bone Morphogenetic Protein and Dose
Perhaps the most critical component in using BMP in the cervical spine is the volume of sponge used per level. This is contingent on the appropriate reconstitution of the BMP-2, the correct positioning of the sponge, and the type of spacer used. As was mentioned previously, approximately 15 minutes should be allowed for the type I collagen sponge to soak in the reconstituted BMP. The dose per level should not exceed 0.7 mg of rhBMP-2, and a dose as low as 0.2 mg may be adequate to achieve a radiographic fusion (unpublished data).27 Currently, the smallest prepackaged available dose is 1.05 mg per sponge, so it is imperative to trim the sponge to an appropriate dose. The divided sponge is lightly placed into the PEEK spacer and secured into position, as discussed later. No sponge is placed along the spacer or in front of the plate.
Review of the complications in the literature related to breathing or swallowing difficulties secondarily appear to be related to dose and placement of the sponge.29,30 In these reports, 2.1 mg of BMP-2 were used per level, and at times the enhanced sponge was placed atop the cervical plate.29 Collectively, these experiences demonstrate that the inflammatory response brought on by BMP-2 may be well tolerated at the local graft site within the confines of the disc space but poorly tolerated when in direct contact with the esophagus and trachea. An appropriate dose limited within a nonporous and nonresorbable spacer has been demonstrated to minimize clinically significant dysphagia.27,28
After placement of the PEEK spacer with the BMP-2–enhanced sponge into the prepared interspace, it is our preference to use no further irrigation. A preoperative dose of dexamethasone may reduce soft tissue edema, and a drain may be utilized postoperatively at the discretion of the surgeon (Fig. 223-25).
Placement of Interbody Graft
Following removal of the PLL and complete decompression, the dimension of the disc space is determined, and an appropriately sized graft is selected for placement (Fig. 223-26). Successful trial selection confirms parallel end-plate preparation. Regardless of the interbody graft selected, the trial should fit flush and produce a tight fit in the disc space (Fig. 223-27). If this is not possible, a larger trial should be attempted, and/or the end plates should be more adequately prepared. Thus, the trials not only help the surgeon to choose the appropriately sized graft, but also help to determine the adequacy of end-plate preparation.
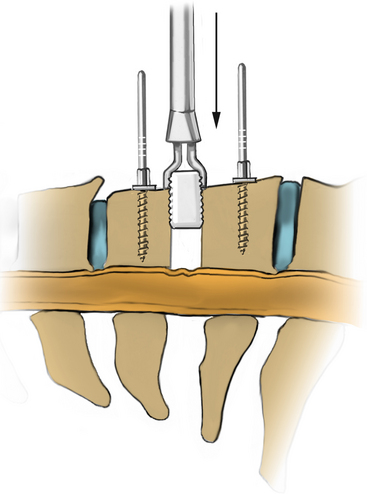
FIGURE 223-27 The graft that corresponds to the final trial is chosen and gently placed into the disc space by using the graft holder.
Choosing a trial that is too large may produce distraction and cause the distraction pins to break out. The strut that corresponds to the final trial is chosen and gently placed into the disc space by using the graft holder (Fig. 223-28). The graft is tapped into the disc space by using a tamp and mallet. The center of hollow implants may be filled with cancellous bone dust from the end plate drilling or with the surgeon’s choice of osteoinductive material. Depending on the bone quality, the graft may be “preloaded” by applying force on the intervertebral body pins. This permits graft compression when the distraction pins are released. Struts are typically countersunk 2 to 3 mm below vertebral body surface. Tension is released on the distractors to ensure a tight fit, and the distraction pins are removed. To check the stability of the newly placed graft, an angled curette can be used to attempt to pull the graft out of the interspace. The angled curette also may be used to palpate the posterior aspect of the graft to ensure that the graft does not compress the spinal cord.
Anterior Cervical Plating
The current design of all cervical plating systems revolves around the screw-plate interface to confer either a constrained or a semiconstrained construct. Currently, all plating systems have a locking mechanism of some sort for the screws to prevent backout. In constrained constructs, fixed-angle screws prevent any motion of the construct, while semiconstrained devices with a combination of fixed-angle and variable-angle screws allow for an element of subsidence of the construct. Semiconstrained plates may also have a rotational or translational component, with translation or rotation occurring again at the plate-screw interface. Thus, depending on the screw used, that is, fixed or variable angle, the construct may be fixed, hybrid, or variable.31–33
Regardless of the plating system to be used, the vertebral bodies need to be prepared for the instrumentation. Soft tissue and ventral osteophytes should have been adequately removed to allow the plate to sit evenly on the spine. The Atlantis hybrid plate (Medtronic Sofamor Danek, Memphis, TN) is described here, although any of the plating systems on the market can be used. Using the Atlantis plate, we typically place fixed screws caudally and use variable screws rostrally. An appropriately sized plate is selected (Fig. 223-29). The edge of the plate should not involve the adjacent unfused disc spaces.
Figure 223-30 illustrates different angles achievable with hardware that allows variable screw placement. Once the drill guide is seated, the screw hole is drilled by using either a 13-mm drill bit or an adjustable drill bit with an adjustable drill stop. Unless clinically indicated, we routinely place unicortical screws. The drilled hole is tapped at the same angle. In most cases, only the outer cortex requires tapping. If the screw length is not known at this point, a depth gauge may be used to verify or select appropriate screw length. A second screw is placed diagonally from the first screw on the opposite side of the plate.
After placement of the first two screws, the plate-holding pin is removed, and the remaining bone screws are placed after drilling and tapping. Final tightening is performed sequentially until the plate evenly and firmly abuts the surface of the spine. The medial angulation also enhances screw-plate stability. Problems with plating can arise if the graft is not fully seated, gardening is suboptimal, or the plate is too long (Fig. 223-31).
Conclusion
The last 50 years have brought continuous refinement to the ventral discectomy and fusion. Collectively, the introduction of the cervical plate and advancements in understanding the biology of fusion have made the arthrodesis almost the rule. These advancements have allowed for the development of an array of interbody graft options that forgo the need for autograft harvest without compromising fusion. This chapter has presented a basic description of the ventral cervical discectomy with allograft fusion techniques. Work reported by Kaiser et al. has demonstrated that fusion and excellent clinical results can be achieved by using the techniques describe herein.4
Baskin D.S., Ryan P., Sonntag V., et al. A prospective, randomized, controlled cervical fusion study using recombinant human bone morphogenetic protein-2 with the CORNERSTONE-SR allograft ring and the ATLANTIS anterior cervical plate. Spine (Phila Pa 1976). 2003;28(12):1219-1224. discussion 1225
Connolly P.J., Esses S.I., Kostuik J.P. Anterior cervical fusion: outcome analysis of patients fused with and without anterior cervical plates. J Spinal Disord. 1996;9(3):202-206.
Haid R.W., Foley K.T., Rodts G.E., Barnes B. The Cervical Spine Study Group anterior cervical plate nomenclature. Neurosurg Focus. 2002;12(1):E15.
Kaiser M.G., Haid R.W.Jr., Subach B.R., et al. Anterior cervical plating enhances arthrodesis after discectomy and fusion with cortical allograft. Neurosurgery. 2002;50(2):229-236. discussion 236–238
Tumialán L.M., Pan J., Rodts G.E., Mummaneni P.V. The safety and efficacy of anterior cervical discectomy and fusion with polyetheretherketone spacer and recombinant human bone morphogenetic protein-2: a review of 200 patients. J Neurosurg Spine. 2008;8(6):529-535.
Wang J.C., McDonough P.W., Endow K., et al. The effect of cervical plating on single-level anterior cervical discectomy and fusion. J Spinal Disord. 1999;12(6):467-471.
1. Cloward R. The anterior approach for the removal of ruptured cervical discs. J Neurosurg. 1958;15:602-615.
2. Smith G.R., Robinson R. The treatment of cervical spine disorders by anterior removal of the intervertebral disc and interbody fusion. J Bone Joint Surg [Am]. 1958;40:607-624.
3. Robinson R.S., Smith G. Anterior lateral disc removal and interbody fusion for cervical disc syndrome. Bull Johns Hopkins Hosp. 1955;69:223-224.
4. Kaiser M.G., Haid R.W.Jr., Subach B.R., et al. Anterior cervical plating enhances arthrodesis after discectomy and fusion with cortical allograft. Neurosurgery. 2002;50(2):229-236. discussion 36–38
5. Beutler W.J., Sweeney C.A., Connolly P.J. Recurrent laryngeal nerve injury with anterior cervical spine surgery risk with laterality of surgical approach. Spine (Phila Pa 1976). 2001;26(12):1337-1342.
6. Boden S.D., Schimandle J.H. Biologic enhancement of spinal fusion. Spine (Phila Pa 1976). 1995;20(Suppl 24):113S-123S.
7. Laurie S.W., Kaban L.B., Mulliken J.B., Murray J.E. Donor-site morbidity after harvesting rib and iliac bone. Plast Reconstr Surg. 1984;73(6):933-938.
8. Pollock R., Alcelik I., Bhatia C., et al. Donor site morbidity following iliac crest bone harvesting for cervical fusion: a comparison between minimally invasive and open techniques. Eur Spine J. 2008;17(6):845-852.
9. Brown M.D., Malinin T.I., Davis P.B. A roentgenographic evaluation of frozen allografts versus autografts in anterior cervical spine fusions. Clin Orthop Relat Res. 1976;119:231-236.
10. Cloward R.B. Gas-sterilized cadaver bone grafts for spinal fusion operations. A simplified bone bank. Spine (Phila Pa 1976). 1980;5(1):4-10.
11. Grossman W., Peppelman W.C., Baum J.A., Kraus D.R. The use of freeze-dried fibular allograft in anterior cervical fusion. Spine (Phila Pa 1976). 1992;17(5):565-569.
12. Young W.F., Rosenwasser R.H. An early comparative analysis of the use of fibular allograft versus autologous iliac crest graft for interbody fusion after anterior cervical discectomy. Spine (Phila Pa 1976). 1993;18(9):1123-1124.
13. Zdeblick T.A., Ducker T.B. The use of freeze-dried allograft bone for anterior cervical fusions. Spine (Phila Pa 1976). 1991;16(7):726-729.
14. Connolly P.J., Esses S.I., Kostuik J.P. Anterior cervical fusion: outcome analysis of patients fused with and without anterior cervical plates. J Spinal Disord. 1996;9(3):202-206.
15. Schneeberger A.G., Boos N., Schwarzenbach O., Aebi M. Anterior cervical interbody fusion with plate fixation for chronic spondylotic radiculopathy: a 2- to 8-year follow-up. J Spinal Disord. 1999;12(3):215-220. discussion 221
16. Wang J.C., McDonough P.W., Endow K., et al. The effect of cervical plating on single-level anterior cervical discectomy and fusion. J Spinal Disord. 1999;12(6):467-471.
17. Wang J.C., McDonough P.W., Endow K.K., Delamarter R.B. Increased fusion rates with cervical plating for two-level anterior cervical discectomy and fusion. Spine (Phila Pa 1976). 2000;25(1):41-45.
18. Wang J.C., McDonough P.W., Kanim L.E., et al. Increased fusion rates with cervical plating for three-level anterior cervical discectomy and fusion. Spine (Phila Pa 1976). 2001;26(6):643-646. discussion 646–647
19. Hanada K., Solchaga L.A., Caplan A.I., et al. BMP-2 induction and TGF-beta 1 modulation of rat periosteal cell chondrogenesis. J Cell Biochem. 2001;81(2):284-294.
20. Riley E.H., Lane J.M., Urist M.R., et al. Bone morphogenetic protein-2: biology and applications. Clin Orthop Relat Res. 1996;324:39-46.
21. Hassan M.Q., Tare R.S., Lee S.H., et al. BMP2 commitment to the osteogenic lineage involves activation of Runx2 by DLX3 and a homeodomain transcriptional network. J Biol Chem. 2006;281(52):40515-40526.
22. Kawamura M., Urist M.R. Induction of callus formation by implants of bone morphogenetic protein and associated bone matrix noncollagenous proteins. Clin Orthop Relat Res. 1988;236:240-248.
23. BMP 2—Genetics Institute/Medtronic-Sofamor Danek/Integra. Bone morphogenetic protein 2—Genetics Institute/Medtronic-Sofamor Danek/Integra, INFUSE Bone Graft, recombinant human bone morphogenetic protein 2—Genetics Institute/Medtronic-Sofamor Danek/Integra, RhBMP 2—Genetics Institute/Medtronic-Sofamor Danek/Integra. BioDrugs. 2002;16(5):376-377.
24. Hsu H.P., Zanella J.M., Peckham S.M., Spector M. Comparing ectopic bone growth induced by rhBMP-2 on an absorbable collagen sponge in rat and rabbit models. J Orthop Res. 2006;24(8):1660-1669.
25. Mok J.M., Durrani S.K., Piper S.L., et al. Extravasation of rhBMP-2 with use of postoperative drains after posterolateral spinal fusion. Spine. 2008;33(15):1668-1674.
26. Geiger M., Li R.H., Friess W. Collagen sponges for bone regeneration with rhBMP-2. Adv Drug Deliv Rev. 2003;55(12):1613-1629.
27. Tumialan L.M., Pan J., Rodts G.E., Mummaneni P.V. The safety and efficacy of anterior cervical discectomy and fusion with polyetheretherketone spacer and recombinant human bone morphogenetic protein-2: a review of 200 patients. J Neurosurg Spine. 2008;8(6):529-535.
28. Baskin D.S., Ryan P., Sonntag V., et al. A prospective, randomized, controlled cervical fusion study using recombinant human bone morphogenetic protein-2 with the CORNERSTONE-SR allograft ring and the ATLANTIS anterior cervical plate. Spine (Phila Pa 1976). 2003;28(12):1219-1224. discussion 1225
29. Shields L.B., Raque G.H., Glassman S.D., et al. Adverse effects associated with high-dose recombinant human bone morphogenetic protein-2 use in anterior cervical spine fusion. Spine (Phila Pa 1976). 2006;31(5):542-547.
30. Smucker J.D., Rhee J.M., Singh K., et al. Increased swelling complications associated with off-label usage of rhBMP-2 in the anterior cervical spine. Spine (Phila Pa 1976). 2006;31(24):2813-2819.
31. Haid R.W., Foley K.T., Rodts G.E., Barnes B. The Cervical Spine Study Group anterior cervical plate nomenclature. Neurosurg Focus. 2002;12(1):E15.
32. Dipaola C.P., Jacobson J.A., Awad H., et al. Screw orientation and plate type (variable- vs. fixed-angle) affect strength of fixation for in vitro biomechanical testing of the Synthes CSLP. Spine J. 2008;8(5):717-722.
33. Fogel G.R., Liu W., Reitman C.A., Esses S.I. Cervical plates: comparison of physical characteristics and in vitro pushout strength. Spine J. 2003;3(2):118-124.
Anterior Cervical Discectomy and Fusion with Autograft and without Plating
Cervical Myelopathy
Cervical myelopathy is a pathologic condition of the cervical spinal cord that is commonly due to spinal cord compression from a large central herniation, cervical spondylosis, tumor, or ossification of the posterior longitudinal ligament. Pathologically, this condition is characterized by both gray and white matter destruction and by demyelinization above and below the level of compression.1 Clinically, it is characterized by a constellation of symptoms and signs, the manifestation of which depends on the level and nature of the anatomic structures that are compressed. The clinical picture may be so unusual that diagnostic confusion may ensue, and the patient may be mislabeled with any one of a host of other diagnoses, including motor neuron disease, multiple sclerosis, Guillain-Barré syndrome, peripheral neuropathy, or intracranial causes. The patient may present with very nondescript symptoms such as a subtle gait disturbance or mild hand numbness. At the other extreme, the patient may be floridly myelopathic and present as wheelchair-bound, with loss of fine motor control of the hands or with incontinence.
The diagnosis of cervical myelopathy is confirmed radiographically by the presence of spinal cord compression. MRI has replaced CT myelography as the preferred imaging modality, although the latter remains a useful imaging modality because it distinguishes bone from other compressive pathology better than MRI does, and it often shows subtle degrees of foraminal pathology better than MRI does.2 Whenever doubt exists, the clinician should have a low threshold for obtaining a myelogram and postmyelogram CT scan. Radiographic findings of cervical spinal cord compression include absence of the subarachnoid space at the site of compression or compression or frank distortion with deformation of the spinal cord itself. Such findings are often associated with a preexisting narrowed spinal canal, on either a congenital or an acquired basis. Such narrowing diminishes the “functional reserve” of the spinal canal, thereby permitting spinal cord compression by a comparatively smaller extradural lesion than would otherwise be required to cause symptomatic spinal cord compression. Occasionally, spinal cord signal changes may exist at sites of extreme compression, indicative of myelomalacia.
Axial Neck Pain
Surgery for axial pain, in contrast to radicular pain, does not carry the imperative of neural decompression, since the goal of surgery is stabilization of a presumed painful segment. Some fusion techniques for neck pain, such as the Keystone graft technique, therefore involve only partial discectomy without nerve root or spinal cord decompression.3
Surgical Technique
Intraoperative Considerations
Incision
The decision regarding the side of surgical approach is based on anatomic considerations related to the recurrent laryngeal nerve (RLN) and the thoracic duct. The RLN is located in the tracheoesophageal groove and is more constant in location on the left side of the neck than on the right. This has prompted some surgeons to recommend a left-sided surgical approach to minimize the risk of vocal cord paralysis from damage to the RLN, although a left-sided surgical approach is, arguably, more difficult for a right-handed surgeon than is a right-sided approach. The theoretically greater risk of injury to the RLN from a right-sided surgical approach has not been proven clinically. A retrospective review of 328 ventral cervical spine fusions revealed 9 cases (2.7%) of RLN symptoms.4 Although there was a significant difference in the incidence of RLN injury between primary and redo ventral procedures (2.3% vs. 9.5%, respectively), there was no association between side of approach and incidence of RLN symptoms.
Knowledge of the characteristics and location of anatomic landmarks is essential in order to place the skin incision accurately.5 The angle of the jaw is just above the C1-2 level. The hyoid bone is generally at the level of C3, the thyroid cartilage at C4-5, and the cricoid cartilage at C6. The C7-T1 level is located approximately one finger breadth above the sternal notch. A common mistake is to place the skin incision too caudally. This is more likely to occur if the incision is planned with the patient’s neck in extension. For exposures at C4-5 and above, the incision should be placed approximately three finger breadths above the clavicle. For exposures below C5, the incision should be about two finger breadths above the clavicle.
Approach
The key to the surgical approach to the ventral cervical spine is understanding the fascial layers in the neck. The cervical fascia consists of one superficial layer and four deep layers.6 The superficial fascia surrounds the subcutaneous tissue of the neck, including the platysma. The deep fascia consists of four components:
1. The superficial layer, which surrounds the neck and splits to envelop the SCM and trapezius muscles.
2. The middle layer, which is subdivided into three parts, the third portion of which is the visceral fascia that surrounds the trachea, esophagus, and thyroid gland, and fuses in the midline with the underlying alar fascia.
3. The alar fascia, which lies dorsal to the esophagus and spreads laterally to surround the carotid sheath. It ultimately fuses with the prevertebral fascia laterally over the transverse processes.
4. The prevertebral fascia, which surrounds the vertebral bodies and adjacent paravertebral musculature.
It has been demonstrated that during retraction of the longus colli muscles, the larynx may be displaced against the shaft of the endotracheal tube (ET), thereby allowing impingement on the intralaryngeal portion of the RLN.7 This can result in injury to the RLN with resulting hoarseness, vocal cord fatigue, development of a weak cough, and/or risk of aspiration. In a clinical study involving 900 consecutive ventral cervical surgeries with plating, it was found that the risk of RLN injury could be significantly reduced by monitoring the ET cuff pressure, deflating the cuff after retractor placement, and subsequently reinflating the cuff to 15 mm Hg.7 These data and a concomitant laboratory experiment by the same authors supported the hypothesis that injury to the RLN occurred from ET-mediated compression during retraction. Furthermore, releasing the cuff pressure could reduce the risk of vocal cord paralysis.
Discectomy
Ventral cervical fusion for cervical spondylosis was first described by Robinson and Smith in 1955.8,9 The original technique employed a piece of tricortical iliac graft for the fusion, a method that has traditionally been the gold standard. This method of arthrodesis restores disc space height and thereby enlarges both the central canal and the neural foramen, thus indirectly decompressing the nerve root. The nerve root is therefore decompressed both directly, by removal of disc material or osteophyte, and indirectly, by disc space distraction with bone graft. A successful fusion also provides stability to the level that is fused and therefore minimizes the risk of future segmental instability.
The cartilaginous surfaces of the rostral and caudal end plates are removed with curettes until the underlying subchondral bone is exposed. Many authors feel that this supporting structure should be kept intact, particularly if a noninstrumented fusion is planned, to prevent graft subsidence into the vertebral body. The rate of pseudarthrosis can be reduced, however, by burring the end plates to expose subchondral bone.10 Although this technique results in mild settling of the graft and a slight increase in kyphosis, clinical outcome was the same as with the standard technique of preserving the end plates.10 It is important to render the surfaces of the bone graft and the vertebral end plates as parallel and smooth as possible to maximize bony contact between the two contiguous bone surfaces and therefore increase the likelihood of fusion. The technique of noninstrumented fusion requires more meticulous attention to detail than with instrumentation, since stability with the former is totally dependent upon precise carpentry. The use of ventral hardware, on the other hand, can provide stability and, therefore, ultimately fusion even in the absence of congruent surfaces (Fig. 223-32).
There is debate about whether or not a large dorsal osteophyte causing spinal cord compression should be removed, even in the presence of clinical myelopathy. Some authors feel that it may be left intact, since there seems to be no difference in clinical outcome regardless of the osteophyte, and the osteophyte frequently remodels and resorbs following successful arthrodesis.11–13 Other authors have reported that spontaneous remodeling of the osteophyte may not occur, even in the presence of a solid arthrodesis.14 These discussions may no longer be an issue, since stability concerns about PLL removal, a prerequisite to osteophyte removal, have largely vanished with the near universal use of ventral instrumentation. Both of these structures are commonly removed, particularly in the presence of myelopathy. In cases of myeloradiculopathy, the nerve root should be checked within its neural foramen, and if it is compressed, a foraminotomy should be performed.
If multiple discectomies are planned, the identical procedure is repeated for each of the other levels. Distraction pins for the longitudinal spreader (Caspar) are placed at each vertebral body level, and the terminal pins can be left in place to serve as effective longitudinal soft tissue retractors. For a two-level discectomy, for example, a total of three pins will be required; for a three-level discectomy, four pins are needed. The distraction device is inserted over adjacent pins at each disc space to facilitate the discectomy at each level.
Bone Graft Considerations
The skin incision should be made parallel to, and either just below or above, the iliac crest to avoid placing the skin incision over a superficial and bony prominence. It should begin approximately 3 cm dorsal to the ASIS to avoid injury to the lateral femoral cutaneous nerve and to minimize the risk of fracture of the ASIS (Fig. 223-33). The iliac crest is exposed, and the periosteum is incised. A periosteal elevator is used to obtain subperiosteal exposure of both the external and internal surfaces of the iliac crest, and a sponge is packed between the periosteum and the bone to facilitate exposure and hemostasis. Retraction of the periosteum may be achieved by utilizing two Cobb periosteal elevators, one ventral and one dorsal to the iliac crest.
The graft should be harvested with an oscillating saw, rather than an osteotome, to minimize the risk of producing microfractures of the weight-bearing surfaces of the bone, which could lead to graft failure and eventual collapse.15 The graft can easily be harvested with a double oscillating saw blade that is manufactured in varying widths. The optimal thickness of a tricortical graft depends on the preoperative baseline disc height. For a preoperative disc height of 3.5 to 6.0 mm, an interbody graft of 2 mm above baseline thickness was found to be most appropriate.15 In general, the graft should be at least 5 mm thick to minimize the risk of collapse. Detaching the deep surface of the graft from the ilium may be accomplished with a curved osteotome.
Complications
Hoarseness
Hoarseness is a relatively common complaint in the initial postoperative period.16,17 Usually, it is due to edema from retraction and will subside within a few days. Although persistent hoarseness may indicate injury to either the superior laryngeal nerve or the RLN, a more likely explanation is an endolaryngeal mechanism related to the ET. Indeed, postoperative vocal cord paralysis has been described following general anesthesia for noncervical spine surgeries. A series of 564 patients reported that RLN injury was caused by the ET in 11.2% of cases.18
Injury to the RLN was the most frequent neurologic complication reported by Flynn in his review of more than 36,000 ventral cervical surgery cases.19 This nerve is more constant in location on the left side of the neck, where it arises at the level of the aortic arch and passes beneath the arch to ascend between the trachea and esophagus within the visceral fascia. Injury to the RLN may result in temporary or permanent hoarseness. A left-sided surgical approach, therefore, is theoretically less risky to the nerve than is a right-sided approach. However, a retrospective review of 187 patients undergoing ventral cervical surgery found no difference in RLN symptoms based on side of surgical approach.4 This study was retrospective and nonrandom and relied only on voice changes (hoarseness) or subjective swallowing symptoms as evidence of potential RLN injury. Mild, asymptomatic abnormalities were not included. It is therefore possible that the incidence of nerve injury was underestimated.
Neurologic Complications
Although uncommon, neurologic injury is the most feared complication of ventral cervical surgery. One widely quoted article surveyed 1358 neurosurgeons in 1974 to determine the frequency and severity of neurologic complications following ACDF.19 Responses to the mailed questionnaire were received from 704 surgeons (52% response rate), involving 82,114 cases. No neurologic complications were reported in 71% of the replies (45,457 cases). There were a total of 311 neurologic complications in the remaining 36,657 cases. As was noted previously, the single most common neurologic complication was injury to the RLN (52 cases; 16.7% of all complications). Postoperative myelopathy occurred in 78 cases. Of the 70 cases that had sufficient information for analysis, there was no apparent cause in 24 (34% of cases), and 38 (54%) were thought to be due to intraoperative trauma. The author concluded that the risk of major neurologic complication was 1 in 355 cases.
Neurologic complications following surgery for cervical myelopathy were reviewed in a series of 384 patients.20 Two hundred four of the patients had ventral surgery, including 134 with ventral interbody fusions. Overall, 21 patients (5.5% of total) sustained neurologic deterioration related to their surgery, including 3% involving the C5 root. Deterioration involved either spinal cord function or nerve root function. Causes of spinal cord dysfunction included intraoperative spinal cord injury, malalignment of the spine associated with graft complication, and epidural hematoma. Paralysis of the deltoid and biceps brachii muscles was related to C5 nerve root deterioration. Causes of this paralysis included direct injury to the nerve, spine malalignment related to graft complications, and tethering or distraction of the nerve root from shifting of the spinal cord after decompression. The C5 root is short and is commonly the most ventral of the roots, since it typically lies at the point of maximal cervical lordosis. These factors make it susceptible to a traction type of injury following decompression, particularly dorsal decompression. In addition, the deltoid muscle commonly has a single root innervation from C5, making an injury to this root more clinically apparent.
Less common neurologic involvement included phrenic nerve injury. Bilateral phrenic nerve palsy has been described following ventral decompression and fusion for cervical ossification of the posterior longitudinal ligament.21 The diagnosis of bilateral phrenic nerve palsy was suspected when the patient unexpectedly failed to wean from the ventilator after surgery, and it was diagnosed radiographically by a postoperative chest radiograph that showed bilateral elevation of the diaphragm. The etiology of this complication was thought to be possible bilateral C4 nerve root stretching, although a central cord etiology could not be excluded.
Horner Syndrome
Horner syndrome is an unusual complication of ventral cervical surgery resulting from injury to the cervical sympathetic chain, which lies on the ventral surface of the longus colli muscle. Excessive dissection, retraction, or electrocoagulation of the longus colli may result in injury to the sympathetics. Horner syndrome is characterized by ptosis, meiosis, and anhydrosis and may initially be unrecognized. Its estimated incidence is 2%, with less than 1% being permanent.19,22 Risk to the cervical sympathetics may be minimized by identifying the midline of the cervical vertebrae and by beginning the subperiosteal dissection of the longus colli at that point and avoiding excessive lateral exposure of the muscle. Caution should be exercised in using electrocautery laterally within the muscle.
Vascular Complications
The major vascular concern in ventral cervical surgery is injury to the vertebral artery. This complication is serious both because of its potential neurologic sequelae and because of the difficulty in controlling hemorrhage. Vertebral artery injury is more likely to occur during vertebral corpectomy than with simple discectomy. In a retrospective review of 10 patients who sustained iatrogenic vertebral artery injury during cervical decompression, all injuries were associated with corpectomy, and all occurred as a result of an air drill.23 Neurologic deficits were noted in half of the patients, although most resolved. The estimated incidence of this injury was 0.5%.
Vertebral artery injury can occur as a result of excessive lateral bone or disc removal, from distorted anatomy from tumor or infection, or if the dissection is inadvertently off the midline as a result of surgeon disorientation. The risk of arterial injury can be minimized by limiting the lateral exposure to the medial border of the longus colli muscles.23 Abnormal course of the vertebral artery can predispose to arterial injury. An angiographic, CT, and MRI review of 23 patients who underwent ventral cervical fusion revealed mild vertebral artery tortuosity in 10 patients and loop formation as a result of spondylotic changes in 3 patients.24 Narrowing of the disc space results in a relative elongation and redundancy of the vertebral artery, which can develop a tortuous course between its fixed position in the foramen transversarium proximally and distally. During ventral decompression, the looped vertebral artery is in jeopardy from an excessively wide resection of the bone or disc. In extreme cases of vertebral artery tortuosity, even routine cervical decompression can injure a looped vertebral artery.
Control of hemorrhage from vertebral artery injury can be extremely difficult. Control can be achieved by tamponade, by direct exposure with subsequent arterial repair, or by hemostasis with suture, electrocoagulation, or hemostatic clip.23 Ligation of one vertebral artery is usually not associated with any neurologic consequence unless there is arterial dominance. In this situation, one artery (usually the left vertebral artery) is dominant and supplies a greater portion of the blood supply to the hindbrain. If this situation is present and the dominant artery is injured and ligated, cerebellar or brainstem infarction can occur. Nerve root injury can result from blind placement of a suture to control bleeding.
Esophageal Injury
Manifestations of esophageal injury following ventral cervical surgery can range from mild dysphagia to esophageal perforation.16 Fortunately, dysphagia, although common, is usually mild and transient. Surgical decompression and fusion are performed ventrally, but the surgical approach is lateral, thereby necessitating some element of retraction of the esophagus and other tissues. Edema is thought to be a major cause of swallowing difficulties, although other causes exist. These include mechanical etiologies such as postoperative hematoma, abscess, or mechanical compression from prominent hardware or bone graft. Denervation may also play a contributing role.
Esophageal perforation is one of the most feared complications of ventral cervical surgery. The incidence of this complication is difficult to determine, but estimates range from 0.2% to 0.94%.25–27 A survey of members of the Cervical Spine Research Society identified 22 cases of esophageal perforations following ventral cervical spine surgery.26 Six were identified at the time of surgery, 6 were identified within the first postoperative week, and 10 were identified late (weeks to years later). There was one fatality due to sepsis. All of the immediately recognized cases were due to either a sharp or motorized instrument. Hardware prominence that caused esophageal erosion was a contributing factor in 40% of the late cases, and prominence of either bone or polymethylmethacrylate contributed in another 40%. Diagnosis was confirmed by esophagraphy or by reexploration. Recommended treatment of an acute perforation consisted of immediate repair, drainage, and parenteral antibiotics, although two cases were successfully treated by parenteral feeding tubes and antibiotics alone. The combination of cervical fracture with the use of hardware was thought to be a risk factor for this complication. A high index of clinical suspicion is of paramount importance in promptly and accurately diagnosing this complication. Drainage and parenteral antibiotics are the recommended and preferred treatment.
Airway Obstruction
Upper airway obstruction following ventral cervical spine surgery is a potentially life-threatening complication that demands emergent reintubation. Etiologies include laryngospasm, hematoma, vocal cord paralysis, allergic reaction, and edema.28 It is imperative not only that airway obstruction be promptly diagnosed, but also that it be prevented. It is more common following lengthy cervical procedures and is therefore more common following corpectomy than single-level discectomy and fusion. A retrospective review of seven patients with upper airway obstruction requiring immediate reintubation following ventral cervical corpectomy and fusion for myelopathy reported two deaths.28 The etiology of the obstruction was hypopharyngeal edema. This review identified several common risk factors for this complication, including moderate or severe preoperative myelopathy, multilevel corpectomy, lengthy procedure (average length 5 hours), preexisting pulmonary disease, and a heavy smoking history. The risk of this complication can be reduced by maintaining the ET for 24 to 72 hours postoperatively and then monitoring the patient in an intensive-care unit.
Chylothorax
The thoracic duct ascends from the chest, where it lies on the prevertebral fascia. It crosses the subclavian artery and enters the subclavian vein at the level of T1. Injury to the thoracic duct may occur during a left-sided C7-T1 approach. For ventral surgery at the C7-T1 or T1-2 level, therefore, a right-sided approach is preferred. When identified, the thoracic duct should be double ligated.16 If injury to the thoracic duct occurs and is unrecognized, a chylothorax may occur.
Bone Graft Complications
Although most surgeons still consider autogenous iliac crest to be the gold standard graft material for ventral cervical fusion, its use is associated with significant donor morbidity. Donor site complication rates as high as 10% have been reported.29–31 A retrospective review of 187 patients undergoing single-level ACDF obtained questionnaire follow-up of 134 of the patients (71.6% response).32 Functional assessment and visual analogue pain scale were evaluated. Acute symptoms (<3 months postoperatively) of difficulty ambulating were reported by 50.7% of patients. Chronic (>3 months postoperatively) symptoms of pain at the donor site were reported by 26.1% of patients, with a mean visual analogue pain score of 3.8. Work and professional activity restrictions were present in 9.7% of patients. Therefore, patients need to be informed of the adverse effects, as well as the benefits, of autogenous iliac crest bone graft.
The harvest of autogenous ventral iliac crest graft may be associated with injury to neural structures. Potential injury to four nerves may occur with ventral iliac bone grafting: the lateral femoral cutaneous nerve, the ilioinguinal nerve, the iliohypogastric nerve, and the femoral nerve. Injury to the lateral femoral cutaneous nerve can result in meralgia paresthetica, which produces paresthesias or pain along the anterolateral aspect of the thigh. The lateral femoral cutaneous nerve usually passes beneath the sartorius muscle and the inguinal ligament, both of which attach to the ASIS. The nerve therefore usually passes medial to the ASIS and is protected by staying lateral to it. In 10% of cases, however, it may be located up to 2 cm lateral to the ASIS, where it is at risk for injury during ventral bone harvesting. The ilioinguinal and iliohypogastric nerves enter the inguinal canal and may be injured by traction on the nerves during harvesting of bone from the inner wall of the ilium. The ilioinguinal nerve supplies sensation to an area of skin beneath the medial half of the inguinal ligament, along the proximal and medial thigh, to the penis and portions of the scrotum. The iliohypogastric nerve provides sensation to the ventral two thirds of the iliac crest. Injury to these two nerves may result in either pain or paresthesias in the involved areas. Injury to the femoral nerve is uncommon, but the nerve is theoretically vulnerable to injury during bone harvesting from the inner table of the ilium.
Pseudarthrosis
To some extent, clinical outcome is directly related to fusion rate.33 Patients with pseudarthrosis are more likely to be symptomatic than are patients with solid arthrodesis. In one study of 48 patients with radiographic pseudarthrosis following ACDF, one third had symptoms at an average of 5 years follow-up.33
The incidence of pseudarthrosis is directly proportional to the number of levels fused.34 For single-level noninstrumented ACDF, the incidence of pseudarthrosis is approximately 5% to 10%. For ACDF at three or more levels, the nonunion rate may exceed 30%.34,35 In most cases, the caudalmost level is the level that is most likely to develop a pseudarthrosis.33 The pseudarthrosis rate can be reduced by the use of ventral cervical plating. When a symptomatic pseudarthrosis develops, the preferred method of treatment is dorsal fusion. This eliminates the risk associated with having to reoperate through an area of scarring.
Some authors advocate corpectomy for multilevel ventral decompression rather than multilevel discectomy with fusion. A higher fusion rate can be achieved by corpectomy with strut grafting than with multilevel discectomy and interbody grafting without plate fixation.36 Whether or not the pseudarthrosis rate is less with corpectomy than with multilevel discectomy and fusion with plate fixation is unknown.
Apfelbaum R.I., Kriskovich M.D., Haller J.R. On the incidence, cause, and prevention of recurrent laryngeal nerve palsies during anterior cervical spine surgery. Spine (Phila Pa 1976). 2000;25:2906-2912.
Bohlman H.H. Cervical spondylosis with moderate to severe myelopathy. A report of 17 cases treated by Robinson anterior cervical discectomy and fusion. Spine (Phila Pa 1976). 1977;2:151-162.
Buetler W.J., Sweeney C., Connolly P.J. Recurrent laryngeal nerve injury with anterior cervical spine surgery, risk with laterality of surgical approach. Spine (Phila Pa 1976). 2001;26:1337-1342.
Emery S.E., Smith M.D., Bohlman H.H. Upper-airway obstruction after multilevel cervical corpectomy for myelopathy. J Bone Joint Surg [Am]. 1991;73:544-551.
Flynn T.B. Neurologic complications of anterior cervical interbody fusion. Spine (Phila Pa 1976). 1982;7:536-538.
Oga M., Yuge I., Terada K., et al. Tortuosity of the vertebral artery in patients with cervical spondylotic myelopathy: risk factor for the vertebral artery injury during anterior cervical decompression. Spine (Phila Pa 1976). 1996;21:1085-1089.
Robinson R.A., Smith G.W. Anterolateral cervical disc removal and interbody fusion for cervical disc syndrome. Bull Johns Hopkins Hosp. 1955;96:223-224.
Smith G.W., Robinson R.A. The treatment of cervical spine disorders by the anterior removal of the intervertebral disc and interbody fusion. J Bone Joint Surg [Am]. 1958;40:607-624.
1. Ono K., Ota H., Tada K., et al. Ossified posterior longitudinal ligament: a clinicopathologic study. Spine (Phila Pa 1976). 1977;2:126-138.
2. Bell G.R., Ross J.S. Diagnosis of nerve root compression, myelography, computed tomography, and MRI. Orthop Clin North Am. 1992;23:405-419.
3. Simmons E.H., Bhalla S.K. Anterior cervical discectomy and fusion (keystone technique). J Bone Joint Surg [Br]. 1969;51:225-237.
4. Buetler W.J., Sweeney C., Connolly P.J. Recurrent laryngeal nerve injury with anterior cervical spine surgery, risk with laterality of surgical approach. Spine (Phila Pa 1976). 2001;26:1337-1342.
5. Watkins R.G. Cervical, thoracic, and lumbar complications-anterior approach. In: Garfin S.R., editor. Complications of Spine Surgery. Baltimore: Williams & Wilkins; 1989:211-247.
6. Grodinsky M., Holyoke E.A. Fasciae and fascial spaces of head, neck and adjacent regions. Am J Anat. 1938;63:367-408.
7. Apfelbaum R.I., Kriskovich M.D., Haller J.R. On the incidence, cause, and prevention of recurrent laryngeal nerve palsies during anterior cervical spine surgery. Spine (Phila Pa 1976). 2000;25:2906-2912.
8. Robinson R.A., Smith G.W. Anterolateral cervical disc removal and interbody fusion for cervical disc syndrome. Bull Johns Hopkins Hosp. 1955;96:223-224.
9. Smith G.W., Robinson R.A. The treatment of cervical spine disorders by the anterior removal of the intervertebral disc and interbody fusion. J Bone Joint Surg [Am]. 1958;40:607-624.
10. Emery S.E., Bolesta M.J., Banks M.A. Robinson anterior cervical fusion. Comparison of the standard and modified techniques. Spine (Phila Pa 1976). 1994;19:660-663.
11. Bohlman H.H. Cervical spondylosis with moderate to severe myelopathy. A report of 17 cases treated by Robinson anterior cervical discectomy and fusion. Spine. 1977;2:151-162.
12. Kadoya S., Nakamura T., Kwak R. A microsurgical anterior osteophytectomy for cervical spondylotic myelopathy. Spine (Phila Pa 1976). 1984;9:437-441.
13. Yang K.C., Lu X.S., Cai Q.L., et al. Cervical spondylotic myelopathy treated by anterior multilevel decompression and fusion. follow-up report of 214 cases. Clinic Orthop Rel Res. 1987;221:161-164.
14. Stevens J.M., Clifton A.G., Whitear P. Appearances of posterior osteophytes after sound anterior interbody fusion in the cervical spine: a high-definition computed myelographic study. Neuroradiology. 1993;35:227-228.
15. An H.S., Evanich C.J., Nowicki B.H., et al. Ideal thickness of Smith-Robinson graft for anterior cervical fusion. A cadaveric study with computed tomographic correlation. Spine (Phila Pa 1976). 1993;18:2043-2047.
16. Andreshak T.G., An H.S. Complications of cervical spine surgery. In: An H.S., Simpson J.M., editors. Surgery of the Cervical Spine. Baltimore: Williams & Wilkins; 1994:401-426.
17. Graham J.J. Complications of cervical spine surgery. In: Sherk H.H., Dunn E.J., Eismont F.J., et al, editors. The Cervical Spine. ed 2. Philadelphia: Lippincott; 1989:831-837.
18. Yamada M., Hirano M. Ohkubo K: Recurrent laryngeal nerve paralysis: a ten year review of 564 patients. Auris Nasus Larynx. 1983;10(suppl):1-15.
19. Flynn T.B. Neurologic complications of anterior cervical interbody fusion. Spine (Phila Pa 1976). 1982;7:536-538.
20. Yonenobu K., Hosono N., Iwasaki M., et al. Neurologic complications of surgery for cervical compression myelopathy. Spine (Phila Pa 1976). 1991;16:1277-1282.
21. Fujibayashi S., Shikata J., Yoshitomi H., et al. Bilateral phrenic nerve palsy as a complication of anterior decompression and fusion for cervical ossification of the posterior longitudinal ligament. Spine (Phila Pa 1976). 2001;26:E281.
22. Saunders R.L. Complications of corpectomy. In: Tarlov E.C., editor. Complications of Spinal Surgery. Neurological Topics. Rolling Meadows, IL: American Association of Neurological Surgeons; 1991:105-113.
23. Smith M.D., Emery S.E., Dudley A., et al. Vertebral artery injury during anterior decompression of the cervical spine: a retrospective review of ten patients. J Bone Joint Surg [Br]. 1992;75:410-415.
24. Oga M., Yuge I., Terada K., et al. Tortuosity of the vertebral artery in patients with cervical spondylotic myelopathy: risk factor for the vertebral artery injury during anterior cervical decompression. Spine (Phila Pa 1976). 1996;21:1085-1089.
25. Capen D.A., Garland D.E., Waters R.L. Surgical stabilization of the cervical spine: a comparative analysis of anterior and posterior spine fusions. Clin Orthop. 1985;196:229-239.
26. Newhouse K.E., Lindsey R.W., Clark C.R., et al. Esophageal perforation following anterior cervical spine surgery. Spine (Phila Pa 1976). 1989;14:1051-1053.
27. Tew J.M., Mayfield F.H. Complications of surgery of the anterior cervical spine. Clin Neurosurg. 1976;23:424-434.
28. Emery S.E., Smith M.D., Bohlman H.H. Upper-airway obstruction after multilevel cervical corpectomy for myelopathy. J Bone Joint Surg [Am]. 1991;73:544-551.
29. Arrington E.D., Smith W.J., Chambers H.G., et al. Complications of iliac bone graft harvesting. Clin Orthop. 1996;329:300-309.
30. Ebraheim N.A., Hossein E., Rongming X. Bone-graft harvesting from iliac and fibular donor sites: techniques and complications. J Am Acad Orthop Surg. 2001;9:210-218.
31. Keene J.S., McKinley N.E. Iliac crest versus spinous process grafts in posttraumatic spinal fusion. Spine (Phila Pa 1976). 1992;17:790-794.
32. Silber J.S., Anderson G., Daffner S.D., et al. Donor site morbidity after anterior iliac crest bone harvest for single-level anterior cervical discectomy and fusion. Spine (Phila Pa 1976). 2003;28:134-139.
33. Phillips F.M., Carlson G., Emery S.E., et al. Anterior cervical pseudarthrosis. Natural history and treatment. Spine (Phila Pa 1976). 1997;22:1585-1589.
34. Herkowitz H.N. A comparison of anterior cervical fusion, cervical laminectomy, and cervical laminoplasty for the surgical management of multiple level spondylotic radiculopathy. Spine (Phila Pa 1976). 1988;13:774-780.
35. Emery S.E., Fisher J.R., Bohlman H.H. Three-level anterior cervical discectomy and fusion: radiographic and clinical results. Spine (Phila Pa 1976). 1997;22:2622-2624.
36. Hilibrand A.S., Fye M.A., Emery S.E., et al. Increased rate of arthrodesis with strut grafting after multilevel anterior cervical decompression. Spine (Phila Pa 1976). 2002;27:146-151.
Anterior Cervical Discectomy with Artificial Disc
The option of disc arthroplasty is emerging. Cervical disc arthroplasty or total intervertebral disc replacement (TDR) seems to be a promising nonfusion alternative for the treatment of degenerative disc disease for cases of predominantly soft cervical disc herniation.1 TDR is designed to preserve motion, avoid limitations of fusion, and allow patients to quickly return to routine activities. The primary goal of the procedure in the cervical spine is to maintain segmental motion after removing the local pathology and, by doing this, to prevent later adjacent-level degeneration,2 as is occasionally observed after ACDF owing to increased stress at those adjacent levels. TDR also avoids the morbidity of bone graft harvest, pseudarthrosis, issues caused by ventral cervical plating, and cervical immobilization side effects.2 The first cervical disc arthoplasty clinical trial in the United States was the Bryan (Medtronic Sofamor Danek, Memphis, TN) disc study initiated in May 2002 after a European prospective human clinical trial began in 2000.3 The results of the European clinical trial, though neither randomized nor controlled, validated the stability, biocompatibility, and functionality predicted by clinical testing. Lafuente et al.4 also showed that the Bryan cervical disc prosthesis seems reliable and safe in the treatment of patients with cervical spondylosis (Fig. 223-34). Although the mechanical rationale seems obvious and TDR thus can be considered an attractive tool in treating cervical soft disc herniation, Peng-Fei and Yu-Hua5 recently stated that the follow-up time of the studies that have already been published is not (yet) long enough to support the advantage of cervical disc prosthesis technology. On the contrary, Walraevens et al. recently showed that up to 8 years after surgery with the Bryan disc, maintenance of mobility at the treated level is seen in 95% of cases.6 Moreover, they documented that the prosthesis seems to protect against acceleration of adjacent-level degeneration as seen after ACDF. Finally, 90% of all patients were shown to have a clinical good to excellent outcome at each postoperative time interval up to 8 years.
Theoretical Aspects
The success and long-term stability of a prosthesis depend on the prosthetic design and material and on the technique and technology used by the surgeon to implant the prosthesis. The prosthesis should provide an immediate postoperative stable interface with the vertebral bodies and subsequent biologic ingrowth of bone to ensure long-term stability (osseointegration). The device should have significant strength and durability. It should be biomechanically compatible, and it should not be associated with excessive subsidence or migration into adjacent bone.
The choice of material for a prosthesis should consider the needs of the articulating surfaces and the interface between prosthesis and vertebral body, in an attempt to maintain the normal range and type of intervertebral motion while transmitting axial loading forces from the vertebral body above to the one below.7
The Bryan cervical disc prosthesis, for example, is a compressible unconstrained prosthesis that attains initial fixation through a press fit relationship with the end plates. The Prestige (Medtronic Sofamor Danek) cervical disc prosthesis is a ball-and-socket joint that is relatively constrained and requires no screw fixation. The Prodisc-C (Synthes Spine, West Chester, PA) device consists of a metal alloy and is a ball-and-socket joint too but is more constrained than the Prestige in that it does not allow for sagittal translation. There are many more prostheses on the market nowadays, at least outside the United States, such as the PCM (Cervitech, Rockaway, NJ), Cervicore (Stryker Spine, Kalamazoo, MI), Cervidisc (Scient’x-Alphatec Spine, Carlsbad, CA), Mobidisc Cervical Prosthesis (LDR, Austin, TX), and even more. Noteworthy is that from a postoperative imaging stand point, the Bryan prosthesis gives less artifact on MRI than do many other prosthetic devices (Figs. 223-35 to 223-38).
Because our experience is limited to the Bryan prosthesis, the information that is provided in the next parts of this chapter deals primarily with this particular device.
Clinical Aspects
Is Motion Preserved by Total Disc Replacement?
Lots of recent data about the Bryan disc suggest that motion on the prosthetic level is preserved over time in the vast majority of cases. Ranges of motion are measured by using dynamic plain radiograph studies and compared to the ranges of motion of adjacent segments. These studies focus almost exclusively on flexion-extension motion; almost no data about lateroflexion and rotation are available in the literature at this time. Wenger et al. conducted a study in 25 consecutive patients (in whom there were 29 prostheses) that showed a prosthetic motion that was physiologic and very similar to that of the healthy adjacent segments with a follow-up of 2 years.8 The larger series of Walraevens et al. recently showed maintenance of motion at the treated level in 95% of 89 cases at 8 years follow-up.6
Does Preserved Motion Prevent Adjacent-Level Disease?
In vitro studies have shown that fusion at the operated cervical level increases intersegmental motion and load and intradiscal pressure at adjacent levels, factors that are believed to accelerate progression of degeneration at these levels, in comparison to the normal progression related to age.9,10 By preserving motion and maintaining disc height, it may be possible to preserve the normal kinetics of the spine and maybe decrease this acceleration of adjacent-segment degeneration. Recent studies seem to confirm this theoretical advantage, although confirmation by long-term studies comparing ACDFs with prostheses will be necessary. We mention at this time the work of Kim et al.11 that showed significantly different percentages of radiographic adjacent-level changes in ACDF cases (40.74%) compared to TDR patients (17.6%). This was found for single-level disease, as well as for two treated levels. However, the radiographic data of protection against acceleration of adjacent-level disease was not translated symptomatically in the study of Kim et al., as there was no difference between the two study arms in both the visual analogue scale and the neck disability index scores.
How Do Patients Do in the Long Run?
This issue was studied by Heller et al.,12 among many others in the past. They conducted a randomized controlled clinical trial of 463 patients, equivalently treated with ACDF or TDR with the Bryan disc, with a follow-up of two years. Both study groups performed well considering all postoperative outcome measures. After 2 years, the group treated with the artificial disc had a statistically greater improvement in the primary outcome variables: neck disability index and overall success. With regard to implant-associated or implant/surgical-procedure–associated serious adverse events, the TDR group did not do worse; in fact, the contrary was seen. Patients who received the cervical artificial disc returned to work nearly 2 weeks earlier than the fusion patients, which turned out to be statistically significant.
Longer-term follow-up was presented in the aforementioned work of Walraevens et al.6 They conducted a long-term radiography study in 89 patients prospectively examined with 2-year time intervals up to 8 years after surgery to assess the change in motion at the index level and adjacent levels in addition to the evolution of disc degeneration at the adjacent levels up to 8 years after disc replacement surgery with the Bryan cervical disc. Second, the presence and change of heterotopic ossification at the index level and the stability of the device were investigated. Finally, the radiographic results were correlated with general clinical outcome by using Odom’s classification. The authors found that mobility of the treated level was maintained up to 8 years after surgery in 95% of cases. Moreover, they documented that the insertion of the prosthesis did not lead to an increase in mobility of the adjacent levels and seemed to protect against adjacent-level degeneration, as seen after ACDF. Heterotopic ossification was present in approximately 40% of all patients at all follow-up points; 8% of the patients lost motion at the operated level owing to the ossification. Heterotopic ossification did not seem to have progressed at 8 years compared to 4 years follow-up. No cases of anteroposterior migration or of subsidence were found. The prosthesis appeared to be securely anchored in the adjacent bone mass and proved to remain stable up to 8 years after postoperatively. Most relevantly, more than 90% of all patients had a good to excellent clinical outcome on the long run. A mobile prosthesis and (only) a little progression in degeneration at the adjacent levels appeared to promote better clinical outcome.
Indications and Complications
Considering that TDR is designed to overcome certain unfortunate evolutions (such as adjacent-level disease) occurring in ACDF, one must of course take into account what specific complications are related to TDR.13,14
In this matter again, many of the encountered problems, specifically related to TDR, can be avoided by limiting the procedure to well-delineated indications. Nowadays, a soft or hard disc herniation at one mobile cervical level is generally accepted as a good indication to deal with radiculopathy. The effect on myelopathy is disputed. In our opinion, patients presenting with pure axial neck pain are not considered good candidates.
Interestingly, a combination of techniques is also feasible, as Barbagallo et al. recently published.15 Their hybrid surgical technique (fusion at one level, simultaneous TDR at an immediately adjacent level) was evaluated in 24 patients with a mean-follow up of 23.8 months. In all but one patient, clinical improvement was seen, as well as satisfying radiological results (functioning disc prostheses and appropriate fusions through cages). This novel technique can probably be a valuable alternative in selected cases in which one of the levels has preoperatively already lost all or almost all mobility. Further larger studies with longer follow-up are undoubtedly needed to clarify this finding.
Intraoperative and Immediate Postoperative Complications
The intraoperative risk profile is quite similar to the one known from ACDF. Among others, Heller et al.12 and Murrey et al.16 did not encounter any major complications in large groups of patients treated with the Bryan disc or the ProDisc-C prosthesis, respectively.
The demonstration of postoperative kyphosis and its predisposal to adjacent-level degeneration are still being debated.17,18
Early Postoperative Complications
Finally, we mention the sometimes problematic postoperative CT and MR imaging in variable types of TDR.19
Intermediate Follow-Up Complications
Development of heterotopic ossification, in grades as distinguished by Mehren et al.,20 has been described since 2002. There have been recent suggestions that this might be related to severe preexisting disc arthrosis and/or OPLL. If this tends to be confirmed, there might be a rationale to perform CT scans preoperatively to assess OPLL and ossification of the anterior longitudinal ligament (OALL) which may constitute a contraindication for TDR.21 Heller and Goffin have reported that postoperative administration of nonsteroidal anti-inflammatory drugs for 2 or 3 weeks after surgery seems to mitigate the tendency to develop heterotopic ossification.22
Long-Term Follow-Up Complications
Wear debris formation is thought to become a potential enemy of the TDR technology. Wear is the physical process caused by motion across a bearing surface. In prostheses, it is associated with formation of particular debris, loss of joint height, and ultimately failure. Moreover, there is concern that an inflammatory effect can be induced, leading to pain, osteolysis, and prosthetic loosening.23 Both reassuring,24 and warning,25 studies have been published about this issue.
Anderson P., Sasso R., Rouleau J., et al. The Bryan Cervical Disc: wear properties and early clinical results. Spine J. 2004;4:3035-3095.
Goffin J. Complications of cervical disc arthroplasty. Spine Surg. 2006;18:87-98.
Heller J.G., Sasso R.C., Papadopoulus S.M., et al. Comparison of Bryan cervical disc arthroplasty with anterior cervical decompression and fusion. Clinical and radiographic results of a randomized, controlled, clinical trial. Spine (Phila Pa 1976). 2009;34:101-107.
Kim S.W., Limson M.A., Kim S.B., et al. Comparison of radiographic changes after ACDF versus Bryan disc arthroplasty in single and bi-level cases. Eur Spine J. 2009;18:218-231.
McAfee P., Cunningham B., Dmitriev A., et al. Cervical disc replacement porous coated motion prosthesis: a comparative biomechanical analysis showing the key role of the posterior longitudinal ligament. Spine (Phila Pa 1976). 2003;28:1765-1855.
Sasso R.C., Smucker J.D., Hacker R.J., Heller J.G. Artificial disc versus fusion. A prospective, randomized study with 2-year follow up on 99 patients. Spine (Phila Pa 1976). 2007;32:2933-2940.
Walraevens J., Demaerel P., Suetens P., et al. Longitudinal prospective long-term radiographic follow-up after treatment of single-level cervical disc disease with the Bryan Cervical Disc. Neurosurgery. 2010;67(3):679-687.
1. Yue W.M., Bronder W., Highland T.R. Long term results after anterior cervical discectomy and fusion with allograft and plating: 5-11 year radiologic and clinical follow up study. Spine (Phila Pa 1976). 2005;30:2138-2144.
2. Sasso R.C., Smucker J.D., Hacker R.J., Heller J.G. Artificial disc versus fusion. A prospective, randomized study with 2-year follow up on 99 patients. Spine (Phila Pa 1976). 2007;32:2933-2940.
3. Goffin J., Casey A., Kehr P., et al. Preliminary clinical experience with the Bryan cervical disc prosthesis. Neurosurgery. 2002;51:840-847.
4. Lafuente J., Casey A. Petzold, Brew S: The Bryan disc prosthesis as an alternative treatment to arthrodesis in the treatment of cervical spondylosis. J Bone Joint Surg [Br]. 2005;87:508-512.
5. Peng-Fei S., Yu-Hua J. Cervical disc prosthesis replacement and interbody fusion—a comparative study. Int Orthopedics. 2008;32:103-106.
6. Walraevens J., Demaerel P., Suetens P., et al. Longitudinal prospective long-term radiographic follow-up after treatment of single-level cervical disc disease with the Bryan Cervical Disc. Neurosurgery. 2010;67(3):679-687.
7. Sekhon L.H.S., Ball J.R. Artificial cervical disc replacement: principles, types and techniques. Neurology India. 2005;53:445-450.
8. Wenger M., van Hoonacker P., Zachee B., et al. Bryan cervical disc prostheses: preservation of function over time. J Clin Neurosci. 2009;16:220-225.
9. Alund M., Larsson S.E. Three dimensional analysis of neck motion. A clinical method. Spine (Phila Pa 1976). 1990;15:87-91.
10. Eck J.C., Humphreys S.C., Lim T.H., et al. Biomechanical study on the effect of cervical spine fusion on adjacent-level intradiscal pressure and segmental motion. Spine (Phila Pa 1976). 2002;27:2431-2434.
11. Kim S.W., Limson M.A., Kim S.B., et al. Comparison of radiographic changes after ACDF versus Bryan disc arthroplasty in single and bi-level cases. Eur Spine J. 2009;18:218-231.
12. Heller J.G., Sasso R.C., Papadopoulus S.M., et al. Comparison of Bryan cervical disc arthroplasty with anterior cervical decompression and fusion. Clinical and radiographic results of a randomized, controlled, clinical trial. Spine (Phila Pa 1976). 2009;34:101-107.
13. Kibuule L.K., Fischgrund J.S. Complications of cervical disc arthroplasty. Semin Spine Surg. 2009;21:185-193.
14. Goffin J. Complications of cervical disc arthroplasty. Semin Spine Surg. 2006;18:87-98.
15. Barbagallo G.M.V., Assietti R., Corbino L., et al. Early results and review of the literature of a novel hybrid surgical technique combining cervical arthrodesis and disc arthroplasty for treating multilevel degenerative disc disease: opposite or complementary techniques? Eur Spine J. 2009;18:29-39.
16. Murrey D., Janssen M., Delamarter R., et al. Results of the prospective, randomized, controlled multicenter Food and Drug Administration investigational device exemption study of the ProDisc-C total cervical disc replacement versus anterior discectomy and fusion for the treatment of 1-level symptomatic cervical disc disease. Spine J. 2009;9:275-286.
17. Katsura A., Hukuda S., Imanaka T. Anterior cervical plate used in degenerative disease can maintain cervical lordosis. J Spinal Disord. 1996;9:470-476.
18. Goffin J., Geusens E., Vantomme N. Long-term follow up after interbody fusion of the cervical spine. J Spinal Disord Techn. 2004;17:79-85.
19. Sekhon L., Duggal N., Lynch J., et al. Magnetic resonance imaging clarity of the Bryan, ProDisc-C, Prestige LP and PCM cervical arthroplasty devices. Spine (Phila Pa 1976). 2007;32:673-680.
20. Mehren C., Suchomel P., Grochulla F., et al. Heterotopic ossification in total cervical artificial disc replacement. Spine (Phila Pa 1976). 2006;31:2802-2806.
21. Yi S., Lee D.Y., Ahn P.G., et al. Radiologically documented adjacent-segment degeneration after cervical arthroplasty: characteristics and review of cases. Surg Neurol. 2009;72:325-329.
22. Heller J, Goffin J: Classification of paravertebral ossification after insertion of the Bryan Cervical Disc Prosthesis. Presented at the 31st Annual Meeting of the Cervical Spine Research Society, Phoenix, AR, 2003.
23. Anderson P., Sasso R., Rouleau J., et al. The Bryan Cervical Disc: wear properties and early clinical results. Spine J. 2004;4:3035-3095.
24. McAfee P., Cunningham B., Dmitriev A., et al. Cervical disc replacement porous coated motion prosthesis: a comparative biomechanical analysis showing the key role of the posterior longitudinal ligament. Spine (Phila Pa 1976). 2003;28:1765-1855.
25. Cavanaugh D., Nunley P., Kerr E., et al. Delayed hyper-reactivity to metal ions after cervical disc arthroplasty. A case report and literature review. Spine (Phila Pa 1976). 2009;34:262-265.