Chapter 224 Management of a 45-Year-Old with Mechanical Low Back Pain with or without L4-5 Spondylolisthesis and No Neurologic Findings
Nonoperative Management
Spondylolisthesis is commonly classified as isthmic or degenerative, based on the pathogenesis. Isthmic spondylolisthesis is associated with defects in the pars interarticularis, usually bilateral. Degenerative spondylolisthesis is a consequence of facet joint arthritis, ligamentous laxity, and ineffective muscular stabilization, resulting in ventral displacement of one vertebra over the subadjacent vertebra without disruption of the vertebral ring.1,2 Degenerative spondylolisthesis is most common at L4-5 and has a 3:1 female predominance; isthmic spondylolisthesis is most common at L5-S1 with a 3:1 male predominance.3 In a subset of persons participating in the Framingham Heart Study (mean age, 52.66 years), spondylolysis was identified in 11.5% using CT imaging, with almost 80% of these persons having associated spondylolisthesis.3 In this study the incidence of degenerative spondylolisthesis increased from the fifth through eighth decades of life, and about 20% of patients experienced severe back pain. However, no significant association was identified between spondylolisthesis, isthmic or degenerative, and the occurrence of low back pain.
Is the Spondylolisthesis Responsible for Symptoms?
In evaluating a patient with mechanical, nonradicular back pain without neurologic findings noted to have a spondylolisthesis, an initial question might be whether the slip is responsible for symptoms or is simply an incidental radiographic finding. Most patients with spondylolisthesis are asymptomatic and progression of clinical symptoms does not correlate with progression of the slip.4 The clinical significance of “instability,” characterized by change in listhesis demonstrable on flexion and extension lumbar lateral radiographs, is unknown and not clearly correlated with symptoms. Although patients with spondylolisthesis do experience more daily back pain, they do not report greater disability than individuals without a slip.5 The most common clinical entity associated with spondylolisthesis is symptomatic spinal stenosis (i.e., pseudoclaudication) in older patients with a degenerative slip.
What Is the Natural History of Axial Back Pain without Neurologic Findings in Patients with Spondylolisthesis?
Irrespective of pathogenesis, the natural history of axial low back pain in patients with spondylolisthesis is favorable. Most patients do well with conservative care.2 A prospective cohort study demonstrated that 76% of patients with degenerative spondylolisthesis and no neurologic deficits followed for more than 10 years remained without neurologic findings.4 Patients with neurologic symptoms, however, including pseudoclaudication or cauda equina symptoms, deteriorated without surgery. A meta-analysis of nonoperative treatment results in children and young adults with spondylolysis and isthmic grade 1 spondylolisthesis reported 83.9% of patients had a successful clinical outcome at 1 year.6 Interestingly, a successful treatment outcome was not dependent on healing of the spondylolytic lesion.
What Is the Optimal Medical/Interventional Treatment for Axial Back Pain without Neurologic Findings in a Patient with Spondylolisthesis?
A recent evidence-based review of medical and interventional treatment for degenerative spondylolisthesis found no high-quality studies to adequately address the question of whether nonoperative treatment improves the generally favorable natural history of this condition when neurologic symptoms, particularly pseudoclaudication, are absent.2 Lacking data, treatment of chronic axial back pain in patients with spondylolisthesis should be based on well-established, consensus treatment recommendations for chronic back pain without a slip. Exercise, both general aerobic conditioning and spine specific, is the cornerstone of treatment. Recent systematic reviews of exercise in nonspecific chronic low back pain have confirmed the efficacy of different approaches, including lumbar extensor strengthening, lumbar stabilization, and the McKenzie method.7–9 An older, small retrospective study comparing flexion and extension exercise programs in patients with spondylolisthesis and back pain found significantly better functional outcome and pain relief at 3 years in the flexion exercise group.10 A structured dynamic lumbar stabilization exercise program was more effective than usual care in reducing pain intensity and functional disability in a small randomized trial in younger patients with predominantly isthmic spondylolisthesis.11 Multiple studies have demonstrated that general aerobic conditioning is helpful in patients with nonspecific low back pain, but this approach has not been studied specifically in patients with spondylolisthesis.12–14
In most patients with chronic nonradicular low back pain and spondylolisthesis, the anatomic source of the pain remains ambiguous. A recent evidence-based systematic review strongly recommended against provocative discography as a diagnostic procedure in such patients, primarily because of a high rate of false positive responses.15 This study found no evidence that the use of provocative discography to select patients for fusion improved clinical outcomes. Similarly, the same analysis concluded there was “insufficient evidence” to evaluate the utility or validity of medial branch blocks for identification of facet-mediated pain in patients with axial low back pain. However, a recent randomized, double-blind, small controlled trial found that lumbar facet joint neurotomy using radiofrequency current in patients with chronic low back pain was at least statistically significantly superior to a “sham” procedure in improving pain and function.16 Interestingly, in this trial, only 15% of screened low back pain patients met the stringent diagnostic criteria for facet-mediated pain, suggesting that the facet joint is not commonly the source of chronic low back pain.
There is modest evidence that massage, manipulation, and acupuncture may be helpful adjunctive treatments in management of chronic nonspecific low back pain, but they have not been studied specifically in patients with spondylolisthesis.17–19 Similarly, a systematic review of the literature found no evidence that ancillary treatments, including bracing, traction, and electrical stimulation, are effective in degenerative spondylolisthesis.2
Kalichman L., Hunter D.J. Diagnosis and conservative management of degenerative lumbar spondylolisthesis. Eur Spine J. 2008;17:327-335.
Kalichman L., Kim D.H., Ling L., et al. Spondylolysis and spondylolisthesis. Prevalence and association with low back pain in the adult community-based population. Spine (Phila Pa 1976). 2009;34:199-205.
Matsunaga S., Ijiri K., Hayashi K. Nonsurgically managed patients with degenerative spondylolisthesis: a 10 to 18-year follow-up study. J Neurosurg. 2000;93:194-198.
Watters W.C., Bono C.M., Gilbert T.J., et al. An evidence-based clinical guideline for the diagnosis and treatment of degenerative lumbar spondylolisthesis. Spine J. 2009;9:609-614.
1. Kalichman L., Hunter D.J. Diagnosis and conservative management of degenerative lumbar spondylolisthesis. Eur Spine J. 2008;17:327-335.
2. Watters W.C., Bono C.M., Gilbert T.J., et al. An evidence-based clinical guideline for the diagnosis and treatment of degenerative lumbar spondylolisthesis. Spine J. 2009;9:609-614.
3. Kalichman L., Kim D.H., Ling L., et al. Spondylolysis and spondylolisthesis. Prevalence and association with low back pain in the adult community-based population. Spine (Phila Pa 1976). 2009;34:199-205.
4. Matsunaga S., Ijiri K., Hayashi K. Nonsurgically managed patients with degenerative spondylolisthesis: a 10- to 18-year follow-up study. J Neurosurg. 2000;93:194-198.
5. Kauppila L.I., Eustace S., Kiel D.P., et al. Degenerative displacement of lumbar vertebrae: a 25-year follow-up study in Framingham. Spine (Phila Pa 1976). 1998;23:1868-1873.
6. Klein G., Mehlman C.T., McCarty M. Nonoperative treatment of spondylolysis and grade 1 spondylolisthesis in children and young adults. J Pediatr Orthop. 2009;29:146-–156.
7. Mayer J., Mooney V., Dagenais S. Evidence-informed management of chronic low back pain with lumbar extensor strengthening exercises. Spine J. 2008;8:96-113.
8. Standaert C.J., Weinstein S.M., Rumpeltes J. Evidence-informed management of chronic low back pain with lumbar stabilization exercises. Spine J. 2008;8:114-120.
9. May S., Donelson R. Evidence-informed management of chronic low back pain with the McKenzie method. Spine J. 2008;8:134-141.
10. Sinaki M., Lutness M.P., Hstrup D.M., et al. Lumbar spondylolisthesis: retrospective comparison and 3-year follow up of two conservative treatment programs. Arch Phys Med Rehabil. 1989;70:594-598.
11. O’Sullivan P.B., Phyty G.D., Twomey L.T., Allison G.T. Evaluation of specific stabilizing exercise in the treatment of chronic low back pain with radiologic diagnosis of spondylolysis or spondylolisthesis. Spine (Phila Pa 1976). 1997;22:2959-2967.
12. Frost H., Lamb S.E., Moffett J.A.K., et al. A fitness program for patients with chronic low back pain: 2-year follow up of a randomized controlled trial. Pain. 1998:273-279.
13. Moffett J.K., Torgerson D., Bell-Byer S. Randomized controlled trial of exercise for low back pain: clinical outcomes, costs, and preferences. BMJ. 1999;319:279-283.
14. Van der Velde G., Mierau D. The effect of exercise on percentile rank aerobic capacity and self-rated disability in patient with chronic low back pain: a retrospective chart review. Arch Phys Med Rehabil. 2000;81:1457-1463.
15. Chou R., Loeser J.D., Owens D.K., et al. Interventional therapies, surgery and interdisciplinary rehabilitation for low back pain. An evidence-based clinical practice guideline from the American Pain Society. Spine (Phila Pa 1976). 2009;34:1066-1077.
16. Nath S., Nath C.A., Pettersson K. Percutaneous lumbar zygapophysial (facet) joint neurotomy using radiofrequency current, in management of chronic low back pain. Spine (Phila Pa 1976). 2008;33:1291-1297.
17. Assendelft W.J., Morton S.C., Yu E.L., et al. Spinal manipulative therapy for low back pain. A meta-analysis of effectiveness relative to other therapies. Ann Intern Med. 2003;138:871-881.
18. Furlan A.D., Brosseau L., Inamura M., et al. Massage for low back pain: a systematic review within the framework of the Cochrane Collaboration Back Review Group. Spine (Phila Pa 1976). 2002;27:1896-1910.
19. Furlan A.D., van Tulder M.W., Cherkin D.C., et al. Acupuncture and dry-needling for low back pain. Cochrane Database Syst Rev. 2005:CD001351.
Spinal Fusion: Ventral Approach
The ventral approach is a valuable tool for treatment of many pathologic processes in the lumbar spine. In this section we consider the role of ventral surgical options in the treatment of a 45-year-old male with mechanical back pain due to degeneration at L4-5 with or without instability. As with any surgical option ventral approaches, though versatile, are not amenable to all pathologies. In some indications there are clear benefits to the ventral approach, but it has limitations.1,2 This discussion concentrates on the anterior mini-open retroperitoneal approach because this is the most common approach to L4-5. Transperitoneal approaches are an option but are not widely used currently.3 The laparoscopic transperitoneal approach has now been mostly abandoned because it did not lead to better outcomes than the retroperitoneal approach and had higher rates of complications.4–7 In recent years there has been increased interest in the direct lateral access corridor to the ventral aspect of the spine.8 The use of newer retractor systems has facilitated this approach. It is of more use higher in the lumbar spine due to anatomic constraints from the iliac wing and from the lumbosacral plexus.9,10
Establish the Diagnosis
In deciding treatment options the key is to establish the diagnosis. In a patient with low back pain in the absence of neurologic symptoms, this is often the most difficult and important piece of information. The causes of back pain and the workup are addressed in other chapters. The AANS Guidelines on Surgery for Axial Back Pain indicate that surgery for axial back pain may be an option in those patients with a clear symptomatic level or levels who have failed an appropriate course of active nonoperative treatment.11 Surgical treatment for significant instability in the spine is less controversial, though there is no uniform definition of instability.12
In the context of this case the establishment of instability may alter the treatment options selected. Biomechanical studies of stand-alone anterior interbody constructs show that the absence of an intact posterior tension band makes these implants much less stable and that supplemental fixation may be indicated.13 In the setting of isthmic spondylolisthesis ventral stand-alone constructs have been used without supplemental fixation in low-grade slips with reasonable results.14,15 In degenerative spondylolisthesis there has been little written.16
Disc collapse may also be an important factor in choosing an approach. Stand-alone constructs rely on tension of the fibers of the disc anulus for stability.17,18 Discs with collapse have shortened fibers and may be more stable. In clinical terms post hoc analysis of patients in FDA trials of a tapered threaded interbody device showed improved outcomes with increasing loss of disc height preoperatively.19
Why a Ventral Approach?
In the lumbar spine, the majority of the axial load passes through the disc space. Bone forms better under compressive loads. As a result interbody constructs place the fusion mass in the area of highest load and under the best loading conditions for fusion. Surface area for fusion is also larger in interbody constructs. Predominant loads in the intertransverse region of the spine are tension or shear and the area for fusion is smaller. As a result of the wide anular opening in the ventral approach, disc removal and endplate preparation are easier and more complete than with posterior interbody techniques.20
Fusion rates with anterior interbody fusion are high. In prospective studies fusion rates in the 90% range are common.21–24 In one large study the radiographic rate of fusion on reconstructed CT imaging was 100%.22 In comparative studies fusion rates with ventral stand-alone constructs are equal to or better than with dorsal fusion or dorsal interbody constructs.2,14,25 In conjunction with dorsal instrumentation, anterior interbody fusions probably have the most consistently high fusion rates and, in comparison studies against dorsal and dorsal interbody constructs, have shown equivalent or better fusion rates and equivalent or better clinical outcomes.26–28
The ventral approach in the lumbar spine is technically demanding but with experience can be done quickly and safely.1,29–31 Vascular injury rates range from 0% to 11% and decrease as experience increases.29,31,32 Ureteric and enteric injuries are rare.1,33,34 Retrograde ejaculation rates have been highly variable, but in large series from experienced centers this rate can be reduced to the 1% to 3% range. Shorter hospital stays are reported for ventral-only procedures when compared to open dorsal fusions. Blood loss and operative time are also lower. Though the use of an access surgeon is common, at least two studies have shown that operative times and complications are not decreased through use of an access surgeon.35,36 Surgical volume and experience with the approach may be more important than the surgical background of the surgeon doing the approach.
Numerous studies have shown that dorsal open surgical approaches lead to histochemical radiographic and pathologic changes to the dorsal musculature.37–43 Muscle strength is compromised.37 Placement of pedicle screws leads to increased loads on the suprajacent facet.44 This constellation of pathology has been termed fusion disease by Zdeblick. The anterior perirectus approach and the minimal access lateral muscle splitting approach avoid these problems. Some authors have suggested that anterior interbody fusion decreases the risk of adjacent segment degeneration as a result.45–48
Not all patients or pathologic processes are amenable to a ventral approach and in some cases dorsal options alone or combined approaches are a better option. There is limited ability to directly decompress the spinal canal through the ventral approach. Ventral compression by bulging or herniated discs can be dealt with through ventral-only approaches. Dorsal pathology, such as facet or ligamentous hypertrophy, cannot be directly addressed. Indirect decompression is possible. Restoration of disc height can increase foraminal height and volume, thus indirectly decompressing foraminal stenosis.49–53 Reduction of a spondylolisthesis will also improve foraminal stenosis and in the case of a degenerative spondylolisthesis will also improve central canal diameter.49,54 In this case, in which no neurogenic symptoms are present, decompression is not a major goal of surgery.
To perform the discectomy and place an interbody graft, it is necessary to have linear access to the disc space. In patients with high slip angles it may not be possible to access the disc; therefore, it may not be possible to do an anterior interbody fusion. When considering a ventral approach, a standing lateral radiograph with the pelvic symphysis visible on the film will allow assessment of the trajectory. As seen in Figure 224-1A, a line drawn along the superior endplate of S1 passes above the symphysis and a ventral approach is possible. In Figure 224-1B the line goes below the symphysis and a ventral approach would not be possible. In general the L4-5 level is more horizontal, so in this case it is unlikely that a ventral approach would not be possible due to approach angle.
The bifurcation of the inferior vena cava to the common iliac veins generally occurs at or near the L4-5 disc. It is generally visible on the preoperative MRI,55 although some advocate a preoperative CT angiogram to assess its position.56 It is generally possible to mobilize the vessels off the disc, but in rare cases it is impossible and a ventral approach must be aborted. Extensive vascular calcification and aneurysm of the aorta and common iliac vessels are also relative contraindications to a ventral approach to the lumbar spine due to the possibility of compromising the circulation of the lower extremities. In patients who have had previous retroperitoneal surgery, it is very difficult to access and mobilize the vessels; a ventral approach should be avoided.
In some patients, a minimal access lateral approach allows ventral access to the L4-5 level without mobilizing the vessels. This approach is limited by the height of the iliac wing and there is a risk to the lumbosacral plexus even with EMG monitoring.8,9
What Conditions Are Amenable to a Ventral Approach?
The ventral approach can be used for most lumbar pathologies. The treatment of intractable low back pain with disc degeneration has been the main indication for stand-alone interbody fusion and has had good short- and long-term results.21–23,47,57 Other authors have reported much poorer results.58–60 The use in both isthmic and degenerative spondylolisthesis has also been established.14–16,27,28,61 Anterior interbody fusion has been used in the treatment of herniated discs in those patients in whom fusion is indicated.62,63
Interbody Fusion Options
Interbody fusions typically involve a structural component to increase and maintain disc height and a biological component to ensure fusion. The early descriptions of anterior interbody fusion used iliac crest bone graft as a tricortical strut.63 Iliac crest bone provides structural support and is osteogenic. Allograft femoral rings have also been widely and successfully used.21,45,47,64,65 They provide a strong strut and some osteoconductive properties. Cancellous autograft is typically placed inside the ring, although recent studies have used recombinant human bone morphogenetic protein-2 (rhBMP-2).66,67 Studies with BMP have shown a higher failure rate when used as a stand alone.67,68
Numerous interbody implants have been developed using multiple materials. The intitial implants were threaded interbody devices, typically metallic.20,21,24 Threaded cortical allograft dowels were used in the past, although they are no longer widely used.22 Impacted metallic cages were developed to provide a broader footprint to lessen subsidence.69 Metallic interbody implants make imaging to assess fusion difficult and have a modulus of elasticity much higher than cancellous bone. As a result, carbon fiber70 and PEEK71 implants have been developed. Multiple studies have compared the biomechanics of these implants, but there has been little written on clinical outcome differences between them.70,72
Cages can either be used as a stand-alone device or augmented with other stabilizers. Dorsal instrumentation with pedicle screws through a traditional open approach with or without dorsal fusion is commonly used.13,15,25,27 Transfacet screws have also been widely used to augment interior interbody fusion.73,74 In one study translaminar screws were associated with a higher pseudarthrosis rate.75 In recent years anterior tension band plates and cages with screw augmentation have also been developed. Although biomechanical studies have compared these implants, there is a lack of comparative clinical studies.76–80
Motion Preservation Options
In recent years there has been a lot of interest in motion preservation implants using the ventral approach. The best data to date is likely from the FDA trials of the implants that have come to market in the United States. The Charité81 and ProDisc82 trials show short-term results comparable to fusion at 2 years and with limited follow-up at 5 years.83 It is unclear how this technology will fit into our armamentarium in the future.
Brau S.A. Mini-open approach to the spine for anterior lumbar interbody fusion: description of the procedure, results and complications. Spine J. 2002;2(3):216-223.
Burkus J.K., Gornet M.F., Schuler T.C., et al. Six-year outcomes of anterior interbody arthodesis with use of interbody fusion cages and recombinant human bone morphogenetic protein-2. J Bone Joint Surg [Am]. 2009;91(5):1181-1189.
Kwon B.K., Hilibrand A.S. A critical analysis of the literature regarding surgical approach and outcome for adult low-grade isthmic spondylolisthesis. J Spinal Disord Tech. 2005;18(Suppl):S30-S40.
Pradham B.B., Bae H.W., Dawson E.G., et al. Graft reabsorption with the use of bone morphogenetic protein: lessons from anterior lumbar interbody fusion using femoral ring allografts and recombinant human bone morphogenetic protein-2. Spine (Phila Pa 1976). 2006;31(10):E277-E284.
Resnick D.K., Choudhri T.F., Dailey A.T., et al. Guidelines for the performance of fusion procedures for degenerative disease of the lumbar spine. Part 7: Intractable low back pain without stenosis or spondylolisthesis. J Neurosurgery Spine. 2005;2(6):670-672.
Schuler T.C., Burkus J.K. The correlation between preoperative disc height and clinical outcomes after anterior lumbar interbody fusion. J Spinal Disord Tech. 2005;18(5):396-410.
Swan J., Hurwitz E., Malek F., et al. Surgical treatment for unstable low-grade isthmic spondylolisthesis in adults: a prospective controlled study of posterior instrumented fusion compared with combined anterior-posterior fusion. Spine J. 2006;6(6):606-614.
Wai E.K., Santos E.R., Morcom R.A., Fraser R.D. Magnetic resonance imaging 20 years after anterior lumbar interbody fusion. Spine (Phila Pa 1976). 2006;31(17):1952-1956.
1. Brau S.A. Mini-open approach to the spine for anterior lumbar interbody fusion: description of the procedure, results and complications. Spine J. 2002;2(3):216-223.
2. Pradham B.B., Nassar J.A., Delamarter R.B., Wang J.C. Single level lumbar spine fusions: a comparison of anterior and posterior approaches. J Spinal Disord Tech. 2002;15(5):355-361.
3. Gumbs A.A., Bloom N.D., Bitan F.D., Hanan S.H. Open anterior approaches for lumbar spine procedures. Am J Surg. 2007;194(1):98-102.
4. Rodriguez H.E., Connolly M.M., Dracopoulos H., et al. Anterior access to the lumbar spine: laparoscopic versus open. Am Surg. 2002;68(11):978-982.
5. Zdeblick T.A., David S.M. A prospective comparison of surgical approach for anterior L4-L5 fusion: laparoscopic versus mini anterior lumbar interbody fusion. Spine (Phila Pa 1976). 2000;25(20):2682-2687.
6. Kaiser M.G., Haid R.W., Subach B.R., et al. Comparison of the mini-open versus laparoscopic approach for anterior lumbar interbody fusion: a retrospective review. Neurosurgery. 2002;51(1):97-103.
7. Liu J.C., Ondra S.L., Angelos P., et al. Is laparoscopic anterior lumbar interbody fusion a useful minimally invasive procedure? Neurosurgery. 2002;51(Suppl 5):S155-S158.
8. Ozgur B.M., Aryan H.E., Pimenta L., Taylor W.R. Extreme Lateral Interbody Fusion (XLIF): a novel surgical technique for anterior lumbar interbody fusion. Spine J. 2006;6(4):435-443.
9. Knight R.Q., Schwaegler P., Hanscom D., Roh J. Direct lateral lumbar interbody fusion for degenerative conditions; early complication profile. J Spinal Disord Tech. 2009;22(1):34-37.
10. Benglis D.N., Vanni S., Levi A.D. An anatomical study of the lumbosacral plexus as related to minimally invasive transpsoas approach to the lumbar spine. J Neurosurg Spine. 2009;10(2):139-144.
11. Resnick D.K., Choudhri T.F., Dailey A.T., et al. Guidelines for the performance of fusion procedures for degenerative disease of the lumbar spine. Part 7: intractable low back pain without stenosis or spondylolisthesis. J Neurosurg Spine. 2005;2(6):670-672.
12. Resnick D.K. Evidenced based guidelines for the performance of lumbar fusions. Clin Neurosurg. 2006;53:279-284.
13. Oxland T.R., Lund T. Biomechanics of stand alone cages and cages in combination with posterior fixation: a literature review. Eur Spine J. 2000;9(Suppl 1):S95-S101.
14. Min J.H., Jang J.S., Lee S.H. Comparison of anterior and posterior approach instrumented lumbar interbody fusion for spondylolisthesis. J Neurosurg Spine. 2007;7(1):21-26.
15. Kim J.S., Lee K.Y., Lee S.H., Lee H.Y. Which lumbar interbody fusion technique is better in terms of level for the treatment of unstable isthmic spondylolisthesis. J Neurosurg Spine. 2010;12(2):171-177.
16. Takahasi K., Kitahara H., Yamagata M., et al. Long term results of anterior interbody fusion for treatment of degenerative spondylolisthesis. Spine (Phila Pa 1976). 1990;15(11):1211-1215.
17. Tencer A.F., Hampton D., Eddy S. Biomechanical properties of threaded inserts for lumbar interbody spinal fusion. Spine (Phila Pa 1976). 1995;20(22):2408-2414.
18. Patwardhan A.G., Carandang G., Ghanayem A.J., et al. Compressive preload improves the stability of anterior lumbar interbody fusion cage constructs. J Bone Joint Surg [Am]. 2003;85(9):1749-1756.
19. Schuler T.C., Burkus J.K. The correlation between preoperative disc height and clinical outcomes after anterior lumbar interbody fusion. J Spinal Disord Tech. 2005;18(5):396-410.
20. Burkus J.K. Intervertebral fixation: clinical results with anterior cages. Orthop Clin North Am. 2002;33(2):349-357.
21. Sasso R.C., Kitchel S.H., Dawson E.G. A prospective, randomized controlled clinical trial of anterior lumbar interbody fusion using a titanium cylindrical threaded fusion device. Spine (Phila Pa 1976). 2004;29(2):113-122.
22. Burkus J.K., Transfeldt E.E., Kitchel S.H., et al. Clinical and radiographic outcomes of anterior lumbar interbody fusion using recombinant human bone morphogenetic protein-2. Spine (Phila Pa 1976). 2002;27(21):2396-2408.
23. Burkus J.K., Gornet M.F., Dickman C.A., Zdeblick T.A. Anterior lumbar interbody fusion using rhBMP-2 with tapered interbody cages. J Spinal Disord Tech. 2002;15(5):337-349.
24. McAfee P.C., Lee G.A. Anterior BAK instrumentation and fusion: complete versus partial discectomy. Clin Orthop Relat Res. 2002;394:55-63.
25. Maden S.S., Boeree N.R. Comparison of instrumented anterior interbody fusion with instrumented circumferential lumbar fusion. Eur Spine J. 2003;12(6):567-575.
26. Faundez A.A., Schwender J.D., Safriel Y., et al. Clinical and radiographic outcomes of anterior posterior fusion versus transforaminal lumbar interbody fusion for symptomatic disc degeneration: a retrospective comparative study of 133 patients. Eur Spine J. 2009;18(2):203-211.
27. Kim J.S., Choi W.G., Lee S.H. Minimally invasive anterior lumbar interbody fusion followed by percutaneous pedicle screw fixation for isthmic spondylolisthesis: minimum 5-year follow-up. Spine J. 2010;10(5):404-409.
28. Swan J., Hurwitz E., Malek F., et al. Surgical treatment for unstable low-grade isthmic spondylolisthesis in adults: a prospective controlled study of posterior instrumented fusion compared with combined anterior-posterior fusion. Spine J. 2006;6(6):606-614.
29. Bianchi C., Ballard J.L., Abou-Zamzam A.M., et al. Anterior retroperitoneal lumbosacral spine exposure: operative techniques and results. Ann Vasc Surg. 2003;17(2):137-142.
30. Brewster L., Trueger N., Schermer C., et al. Infraumbilical anterior retroperitoneal exposure of the lumbar spine in 128 consecutive patients. World J Surg. 32(7), 2008. 1414–1149
31. Sasso R.C., Best N.M., Mummaneni P.V., et al. Analysis of operative complications in a series of 471 anterior lumbar interbody fusion procedures. Spine (Phila Pa 1976). 2005;30(6):670-674.
32 Hamdan A.D., Malek J.Y. Vascular injury during anterior exposure of the spine. J Vasc Surg. 2008;48(3):650-654.
33. Tiusanen H., Seitsalo S., Osterman K., Soini J. Retrograde ejaculation after anterior interbody lumbar fusion. Eur Spine J. 1995;4(6):339-342.
34. Sasso R.C., Burkus J.K., LeHuec J.C. Retrograde ejaculation after anterior lumbar interbody fusion: transperitoneal versus retroperitoneal approach. Spine (Phila Pa 1976). 2003;28(10):1023-1026.
35. Jarrett C.D., Heller J.G., Tsai L. Anterior exposure of the lumbar spine with and without an “access surgeon”: morbidity analysis of 265 consecutive cases. J Spinal Disord Tech. 2009;22(8):559-564.
36. Holt R.T., Majd M.E., Vadhava M., Castro F.P. The efficacy of anterior spine exposure by an orthopedic surgeon. J Spinal Disord Tech. 2003;16(5):477-486.
37. Gejo R., Matsui H., Kawaguchi Y., et al. Serial changes in trunk muscle performance after posterior lumbar surgery. Spine (Phila Pa 1976). 1999;24(10):1023-1028.
38. Kawaguchi Y., Matsui H., Tsuji H. Changes in serum creatine phosphokinase MM isoenzyme after lumbar spine surgery. Spine (Phila Pa 1976). 1997;22(9):1018-1023.
39. Kawaguchi Y., Yabuki S., Styf J., et al. Back muscle injury after posterior lumbar spine surgery. Topographic evaluation of intramuscular pressure and blood flow in the porcine back muscle during surgery. Spine (Phila Pa 1976). 1996;21(22):2683-2688.
40. Kawaguchi Y., Matsui H., Tsuji H. Back muscle injury after posterior lumbar spine surgery. A histologic and enzymatic analysis. Spine (Phila Pa 1976). 1996;21(8):941-944.
41. Kawaguchi Y., Matsui H., Tsuji H. Back muscle injury after posterior lumbar spine surgery. Part 2: Histologic and histochemical analyses in humans. Spine (Phila Pa 1976). 1994;19(22):2598-2602.
42. Styf J.R., Willén J. The effects of external compression by three different retractors on pressure in the erector spine muscles during and after posterior lumbar spine surgery in humans. Spine (Phila Pa 1976). 1998;23(3):354-358.
43. Weber B.R., Grob D., Dvorak J., Muntener M. Posterior surgical approach to the lumbar spine and its effect on the multifidus muscle. Spine (Phila Pa 1976). 1997;22(15):1765-1772.
44. Chen S.H., Tai C.L., Lin C.Y., et al. Biomechanical comparison of a new stand-alone anterior lumbar interbody fusion cage with established fixation techniques—a three-dimensional finite element analysis. BMC Musculoskelet Disord. 2008;9:88.
45. Van Horn J.R., Bohnen L.M. The development of discopathy in lumbar discs adjacent to a lumbar anterior interbody spondylodesis. A retrospective matched pair study with a postoperative follow-up of 16 years. Acta Orthop Belg. 1992;58(3):280-286.
46. Rao R.D., David K.S., Wang M. Biomechanical changes at adjacent segments following anterior lumbar interbody fusion using tapered cages. Spine (Phila Pa 1976). 2005;30(24):2772-2776.
47. Wai E.K., Santos E.R., Morcom R.A., Fraser R.D. Magnetic resonance imaging 20 years after anterior lumbar interbody fusion. Spine (Phila Pa 1976). 2006;31(17):1952-1956.
48. Min J.H., Jang J.S. The clinical characteristics and risk factors for adjacent segment degeneration in instrumented lumbar fusion. J Spinal Disord Tech. 2008;21(5):305-309.
49. Vamvanij V., Ferrara L.A., Hai Y., et al. Quantitative changes in spinal canal dimensions using interbody distraction for spondylolisthesis. Spine (Phila Pa 1976). 2001;26(3):E13-E18.
50. Motosuneya T., Asazuma T., Nobuta M., et al. Anterior lumbar interbody fusion: changes in area of the dural tube, disc height, and prevalence of cauda equine adhesions on magnetic resonance imaging. J Spinal Disord Tech. 2005;18(1):18-22.
51. Wang M., Delai S. Changes in the lumbar foramen following anterior interbody fusion with tapered cylindrical cages. Spine J. 2007;7(5):563-569.
52. Nibu K., Panjabi M.M., Oxland T., Cholewicki J. Intervertebral disc distraction with a laparoscopic anterior spinal fusion system. Eur Spine J. 1998;7(2):142-147.
53. Chen D., Fay L.A., Lok J., et al. Increasing neuroforaminal volume by anterior interbody distraction in degenerative lumbar spine. Spine (Phila Pa 1976). 1995;20(1):74-79.
54. Kim N.H., Kim H.K., Suh J.S. A computed tomographic analysis of changes in the spinal canal after anterior lumbar interbody fusion. Clin Orthop Relat Res. 1993;286:180-191.
55. Kang B.U., Lee S.H., Jeon S.H., et al. An evaluation of vascular anatomy for minilaparotomic anterior L4-5 procedures. J Neurosurg Spine (Phila Pa 1976). 2006;5(6):508-513.
56. Inamasu J., Kim D.H., Logan L. Three dimensional computed tomographic anatomy of the abdominal great vessels pertinent to L4-L5 anterior lumbar interbody fusion. Minim Invasive Neurosurg. 2005;48(3):127-131.
57. Burkus J.K., Gornet M.F., Schuler T.C., et al. Six-year outcomes of anterior interbody arthodesis with use of interbody fusion cages and recombinant human bone morphogenetic protein-2. J Bone Joint Surg [Am]. 2009;91(5):1181-1189.
58. Pellise F., Puig O., Rivas A., et al. Low fusion rate after laparoscopic anterior lumbar interbody fusion using twin stand-alone carbon fiber cages. Spine (Phila Pa 1976). 2002;27(15):1665-1669.
59. Choi J.Y., Sung K.H. Subsidence after anterior lumbar interbody fusion using paired stand-alone rectangular cages. Eur Spine J. 2006;15(1):16-22.
60. Buttton G., Gupta M. Three- to six-year follow-up of stand-alone BAK cages implanted by a single surgeon. Spine J. 2005;5(2):155-160.
61. Kwon B.K., Hilibrand A.S. A critical analysis of the literature regarding surgical approach and outcome for adult low-grade isthmic spondylolisthesis. J Spinal Disord Tech. 2005;18(Suppl):S30-S40.
62. Vishteh A.G., Dickman C.A. Anterior lumbar microdiscectomy and interbody fusion for the treatment of recurrent disc herniation. Neurosurgery. 2001;48(2):334-337.
63. Inoue S., Watanabe T. Anterior discectomy and interbody fusion for lumbar disc herniation. A review of 350 cases. Clin Orthop Relat Res (183). 1984:22-31.
64. Sacks S. Anterior interbody fusion of the lumbar spine. J Bone Joint Surg [Br]. 1965;47:211-223.
65. Penta M.R.D., Fraser R.D. Anterior lumbar interbody fusion. A minimum 10-year follow-up. Spine (Phila Pa 1976). 1997;22(20):2429-2434.
66. Burkus J.K., Sandhu H.S., Gornet M.F., Longley M.C. Use of rh-BMP-2 in combination with structural cortical allografts: clinical and radiographic outcomes in anterior lumbar spinal surgery. J Bone Joint Surg [Am]. 2005;87(6):1205-1212.
67. Pradhan B.B., Bae H.W., Dawson E.G., et al. Graft reabsorption with the use of bone morphogenetic protein: lessons from anterior lumbar interbody fusion using femoral ring allografts and recombinant human bone morphogenetic protein-2. Spine (Phila Pa 1976). 2006;31(10):E277-E284.
68. Vaidya R., Weir R., Sethi A., et al. Interbody fusion with allograft and rhBMP-2 leads to consistent fusion but early subsidence. J Bone Joint Surg [Br]. 2007;89(3):342-345.
69. Oxland T.R., Hoffer Z., Nydegger T., et al. A comparative biomechanical investigation of anterior lumbar interbody cages: central and bilateral approaches. J Bone Joint Surg [Am]. 2000;82(3):383-393.
70. Jost B., Cripton P.A., Lund T., et al. Compressive strength of interbody cages in the lumbar spine: the effect of cage shape, posterior instrumentation and bone density. Eur Spine J. 1998;7(2):132-141.
71. Vadapalli S., Sairyear K., Goel V.K., et al. Biomechanical rationale for using polyetheretherketone (PEEK) spacers for lumbar interbody fusion—A finite element study. Spine (Phila Pa 1976). 2006;31(26):E992-E998.
72. Tsantrizos A., Andreu A., Aebi M., et al. Biomechanical stability of five stand alone anterior lumbar interbody fusion constructs. Eur Spine J. 2000;9(1):14-22.
73. Best N.M., Sasso R.C. Efficacy of translaminar facet screw fixation in circumferential interbody fusions as compared to pedicle screw fixation. J Spinal Disord Tech. 2006;19(2):98-103.
74. Marchesi D.G., Boos N. Translaminar facet joint screws to enhance segmental fusion of the lumbar spine. Eur Spine J. 1992;1(2):125-130.
75. Anjarwalla N.K., Morcom R.K., Fraser R.D. Supplementary stabilization with anterior lumbar intervertebral fusion—a radiologic review. Spine (Phila Pa 1976). 2006;31(11):1281-1287.
76. Johnson W.M., Nichols T.A., Jethwani D., Guiot B.H. In vitro biomechanical comparison of an anterior and anterolateral lumbar plate with posterior fixation following single level anterior lumbar interbody fusion. J Neurosurg Spine. 2007;7(3):332-335.
77. Tzermiadianos M.N., Mekhail A., Vorono L.I., et al. Enhancing stability of anterior lumbar interbody fusion: a biomechanical comparison of anterior plate versus posterior transpedicular instrumentation. Spine (Phila Pa 1976). 2008;33(2):E38-E43.
78. Beaubien B.P., Derincek A., Lew W.D., et al. In vitro, biomechanical comparison of an anterior lumbar interbody fusion with an anteriorly placed low profile lumbar plate and posteriorly placed pedicle screws or translaminar screws. Spine (Phila Pa 1976). 2005;30(6):1846-1851.
79. Gerber M., Crawford N.R., Chamberlain R.H., et al. Biomechanical assessment of anterior lumbar interbody fusion with an anterior lumbosacral fixation screw-plate: comparison to stand-alone anterior lumbar interbody fusion and anterior lumbar interbody fusion with pedicle screws in an unstable human cadaver model. Spine (Phila Pa 1976). 2006;31(7):762-768.
80. Nichols T.A., Yantzer B.K., Alameda S., et al. Augmentation of an anterior lumbar interbody fusion with an anterior plate or pedicle screw fixation: a comparative biomechanical in vitro study. J Neurosurg Spine (Phila Pa 1976). 2007;6(3):267-271.
81. Blumenthal S., McAfee P.C., Guyer R.D., et al. A prospective, randomized, multicenter Food and Drug Administration investigational device exemptions study of lumbar total disc replacement with the CHARITE artificial disc versus lumbar fusion: part I: evaluation of clinical outcomes. Spine (Phila Pa 1976). 2005;30(14):1565-1575.
82. Zigler J., Delamarter R., Spivak J.M., et al. Results of the prospective, randomized, multicenter Food and Drug Administration investigational device exemption study of the ProDisc-L total disc replacement versus circumferential fusion for the treatment of 1-level degenerative disc disease. 32(11):1155–1162, 200783.Guyer RD, McAfee PC, Banco RJ, et al. Prospective, randomized, multicenter Food and Drug Administration investigational device exemption study of lumbar total disc replacement with the CHARITE artificial disc versus lumbar fusion: five-year follow-up. Spine (Phila Pa 1976). 2007;32(11):1155-1162.
83. Guyer R.D., McAfee P.C., Banco R.J., et al. Prospective, randomized, multicenter Food and Drug Administration investigational device exemption study of lumbar total disc replacement with the CHARITE artificial disc versus lumbar fusion: five-year follow-up. Spine J. 2009;9(5):374-386.
Spinal Fusion: Dorsal Approach
Mechanical low back pain is an extremely common entity in the United States, affecting 15% to 85% of all adults at some point in their lives.1,2 It is the second most common diagnosis-linked cause for a physician visit and has a point prevalence of approximately 30%. Despite the expenditure of nearly $100 billion annually in the United States for the diagnosis and treatment of low back pain–related disorders, low back pain continues to be poorly understood, and a discrete anatomic cause for the pain can be found in only 20% of cases.3 Although the majority of patients experience improvement of their symptoms within 2 to 4 weeks and 90% are improved within 3 months, a small percentage have symptoms persistent and severe enough to warrant surgical intervention.
Although a myriad of surgical procedures have been proposed for the treatment of low back pain secondary to either degenerative disc disease or spondylolisthesis, arthrodesis to stabilize the painful motion segment has been the mainstay of surgical treatment since its introduction in the 1920s.4 Broadly speaking, surgical alternatives for fusion include dorsal, ventral, and combined techniques. The latter can be performed through either an all-dorsal approach (posterior lumbar interbody fusion [PLIF], and transforaminal lumbar interbody fusion [TLIF]), or can be done through separate ventral and dorsal incisions. Within the spectrum of dorsal fusion procedures, dorsolateral intertransverse fusion with or without pedicle screw instrumentation is commonly performed, although other procedures, such as facet fusion, have been more recently developed.5
Mechanical Low Back Pain without Spondylolisthesis
In this chapter, a 45-year-old male patient with mechanical low back pain is discussed. In the patient with degenerative disc disease and low back pain without spondylolisthesis, fusion surgery is still considered controversial but has been successfully performed when using strict operative indications.6,7 Anterior lumbar interbody fusion (ALIF) has been reported as an alternative to posterior fusion and has the benefit of decreased blood loss and operative time, as well as avoiding the morbidity associated with the muscle dissection necessary for posterior fusion. However, concerns with the ventral approach include the risk of visceral or potentially life-threatening vascular injury and deep venous thrombosis. In addition, pseudarthrosis rates after ALIF have been reported to be as high as 31%, and retrograde ejaculation can be a significant issue in young males who wish to reproduce.8 In addition, graft extrusion requiring high-risk anterior revision surgery is a rare but potentially catastrophic complication of this procedure and of lumbar total disc arthroplasty.9 TLIF and PLIF-type procedures are theoretically desirable because they increase the surface area for fusion compared to posterior-alone techniques, but the longer operative times, potential for neurologic injury, and the more recently described potential for postoperative radiculitis and heterotopic bone formation within the spinal canal with the use of bone morphogenetic protein (BMP) are cause for concern.10,11
Dorsolateral intertransverse fusion, in the appropriately selected patient, can avoid the complications described previously and has been shown to provide acceptable clinical results at mid- and long-term follow-up. Glassman et al. studied 224 patients undergoing single-level dorsolateral arthrodesis with iliac crest autograft for lumbar degenerative disease and found significant improvements in both the Short Form-36 and Oswestry Disability Index scores at up to 2-year follow-up.12 They observed a fusion rate of 88% to 95% at 2-year follow-up (in younger and older patients, respectively) and reported modest improvements in back pain, leg pain, and return to work. Dimar et al. performed dorsolateral instrumented fusions on 463 patients with lumbar degenerative disease and up to grade I degenerative spondylolisthesis.13 They reported fusion rates of 96% with use of rhBMP-2 and 89% with iliac crest and had significant improvements in back pain, leg pain, and return to work status at 2-year follow-up. In conclusion, dorsolateral instrumented fusion can result in significant improvements in pain score and health-related quality of life as well as obtaining a high fusion rate in the appropriately selected patient with mechanical low back pain.
Mechanical Low Back Pain with Spondylolisthesis
In the setting of low back pain secondary to degenerative spondylolisthesis, multiple surgical treatment options have been proposed, including laminectomy without fusion, noninstrumented dorsolateral fusion, and instrumented fusion. In addition, several small case series have been performed looking at the short-term results of interbody techniques, including PLIF and TLIF, but at this time no high-quality randomized, controlled trials exist to advocate these techniques.14,15
In the 1991 landmark study by Herkowitz and Kurz, 50 patients with lumbar stenosis and degenerative spondylolisthesis were randomly assigned to either decompression alone (25 patients) or decompression with bilateral noninstrumented intertransverse process arthrodesis (25 patients).16 They reported that 24 patients in the fusion group had excellent or good clinical outcome, whereas 11 patients in the nonfusion group had excellent or good results. In addition, back pain, leg pain, and progression of the spondylolisthesis were all greater in the decompression-alone group.
Although the superior outcomes of dorsolateral fusion versus both nonoperative care and decompression alone have been validated by several studies, the use of instrumentation is more controversial.17,18 Fischgrund et al. reported on 76 patients randomized to either decompression and fusion without instrumentation or decompression and fusion augmented with pedicle screw instrumentation for single-level degenerative spondylolisthesis with lumbar spinal stenosis.19 While they noted a significantly greater fusion rate in the instrumented group, this did not translate into improved clinical outcomes, with good or excellent outcomes seen in 76% of the patients with instrumented fusions, and 85% in patients treated with fusion without instrumentation. Similarly, Thomsen et al. reported on 130 patients randomly assigned to either instrumented or noninstrumented posterolateral fusion for Grades 1 and 2 degenerative spondylolisthesis.20 Although they found a slightly higher global satisfaction rate in the instrumented group (not significant), there was significantly higher operative time, blood loss, and early reoperation rate in the instrumented group, while both groups had similar improvement in pain and disability scores. The authors concluded that instrumentation results in similar outcomes to noninstrumented fusion, which does not justify the increased operative times, risk of neurologic injury, and higher reoperation rate. Despite these studies, pedicle screw instrumentation is widely used to increase stability in the early postoperative period during fusion healing, with many arguing that an increased fusion rate results in better clinical outcomes, and therefore if instrumentation increases the fusion rate then clinical outcomes should follow.21
Fischgrund J., Mackay M., Herkowitz H., et al. 1997 Volvo Award winner in clinical studies. Degenerative lumbar spondylolisthesis with spinal stenosis: a prospective, randomized study comparing decompressive laminectomy and arthrodesis with and without spinal instrumentation. Spine (Phila Pa 1976). 1997;22(24):2807-2812.
Fritzell P., Hagg O., Wessberg P., et al. 2001 Volvo award winner in clinical studies: lumbar fusion versus nonsurgical treatment for chronic low back pain. Spine (Phila Pa 1976). 2001;26(23):2521-2534.
Herkowitz H., Kurz L. Degenerative lumbar spondylolisthesis with spinal stenosis. A prospective study comparing decompression with decompression and intertransverse process arthrodesis. J Bone Joint Surg [Am]. 1991;73(6):802-808.
Parker E., Murrell L., Boden S., et al. The outcome of posterolateral fusion in highly selected patients with discogenic low back pain. Spine (Phila Pa 1976). 1996;21(16):1909-1916.
1. Andersson G. Epidemiological features of chronic low-back pain. Lancet. 1999;354(9178):581-585.
2. Frymoyer J., Pope M., Clements J. Risk factors in low-back pain. An epidemiological survey. J Bone Joint Surg [Am]. 1983;65:213-218.
3. Luo X., Pietrobon R., Sun S., et al. Estimates and patterns of direct health care expenditures among individuals with back pain in the United States. Spine (Phila Pa 1976). 2004;29(1):79-86.
4. Hibbs R., Swift W. Developmental abnormalities at the lumbosacral juncture causing pain and disability. Surg Gynecol Obstet. 1929;48:604-612.
5. Best N., Sasso R. Efficacy of translaminar facet screw fixation in circumferential interbody fusions as compared to pedicle screw fixation. J Spinal Disord Tech. 2006;19:98-103.
6. Fritzell P., Hagg O., Wessberg P., et al. 2001 Volvo award winner in clinical studies: lumbar fusion versus nonsurgical treatment for chronic low back pain. Spine (Phila Pa 1976). 2001;26(23):2521-2534.
7. Parker E., Murrell L., Boden S., et al. The outcome of posterolateral fusion in highly selected patients with discogenic low back pain. Spine (Phila Pa 1976). 1996;21(16):1909-1916.
8. Loguidice V., Johnson R., Guyer R. Anterior lumbar interbody fusion. Spine (Phila Pa 1976). 1988;13(3):366-369.
9. Blumenthal S., Baker J., Dossett A., et al. The role of anterior lumbar fusion for internal disc disruption. Spine (Phila Pa 1976). 1988;13(5):566-569.
10. Rihn J., Patel R., Makda J. Complications associated with single level transforaminal lumbar interbody fusion. Spine J. 2009;9(8):623-629.
11. Lewandrowski K., Nanson C., Calderon R. Vertebral osteolysis after posterior interbody lumbar fusion with recombinant human bone morphogenetic protein 2: a report of five cases. Spine J. 2007;7:609-614.
12. Glassman S., Polly D., Bono C., et al. Outcome of lumbar arthrodesis in patients sixty-five years of age or older. J Bone Joint Surg [Am]. 2009;91:783-790.
13. Dimar J., Glassman S., Burkus K., et al. Clinical and radiographic analysis of an optimized rhBMP-2 formulation as an autograft replacement in posterolateral spine arthrodesis. J Bone Joint Surg [Am]. 2009;91:1377-1386.
14. Yan D., Pei F., Li J., et al. Comparative study of PLIF and TLIF treatment in adult degenerative spondylolisthesis. Eur Spine J. 2008;17(10):1311-1316.
15. Sears W. Posterior lumbar interbody fusion for degenerative spondylolisthesis: restoration of sagittal balance using insert-and-rotate interbody spacers. Spine J. 2005;5(2):170-179.
16. Herkowitz H., Kurz L. Degenerative lumbar spondylolisthesis with spinal stenosis. A prospective study comparing decompression with decompression and intertransverse process arthrodesis. J Bone Joint Surg [Am]. 1991;73(6):802-808.
17. Bridwell K., Sedgewick T., O’Brien M. The role of fusion and instrumentation in the treatment of degenerative spondylolisthesis with spinal stenosis. J Spinal Disord. 1993;6(6):461-472.
18. Weinstein J., Lurie J., Tosteson T., et al. Surgical compared with nonoperative treatment for lumbar degenerative spondylolisthesis: four-year results in the Spine Patient Outcomes Research Trial (SPORT) randomized and observational cohorts. J Bone Joint Surg [Am]. 2009;91(6):1295-1304.
19. Fischgrund J., Mackay M., Herkowitz H., et al. 1997 Volvo Award winner in clinical studies. Degenerative lumbar spondylolisthesis with spinal stenosis: a prospective, randomized study comparing decompressive laminectomy and arthrodesis with and without spinal instrumentation. Spine. 1997;22(24):2807-2812.
20. Thomsen K., Christensen F., Eiskjaer S., et al. 1997 Volvo Award winner in clinical studies. The effect of pedicle screw instrumentation on functional outcome and fusion rates in posterolateral lumbar spinal fusion: a prospective, randomized clinical study. Spine (Phila Pa 1976). 22(24), 1997. 2813–2122
21. Kornblum M., Fischgrund J., Herkowitz H., et al. Degenerative lumbar spondylolisthesis with spinal stenosis: a prospective long-term study comparing fusion and pseudarthrosis. Spine (Phila Pa 1976). 2004;29(7):726-733.
Spinal Fusion: Dorsal and Ventral Approach
Low back pain is among the most common complaints. Roughly 60% to 85% of adults will suffer from low back pain at some point in their lives.1,2 Accurate diagnosis of low back pain is difficult because several anatomic structures are potential generators of pain. For the vast majority of patients, the symptoms are tolerable and greater than 90% of patients gain relief from conservative means within 6 weeks. Chronic low back pain affects roughly 15% to 45% of adults and is characterized by symptoms lasting greater than 3 months.3 Low back pain is a difficult disease to treat mainly because of its enigmatic etiology and inconsistent diagnostic and therapeutic approach among surgeons. Therefore, it is imperative that the spine surgeon has a thorough understanding of the degenerative process and a working knowledge of the effectiveness of the treatment tools at his or her disposal.
The term mechanical low back pain describes a deep, agonizing pain that is worsened with activity (loading) and improved with rest (unloading). Its biomechanical equivalent is the dysfunctional motion segment (Fig. 224-2). Dysfunctional segmental motion is a type of instability related to disc or vertebral body changes (whether degenerative or secondary to an external process such as a tumor) that results in a potential for pain of spinal origin.4 The pain pattern suggests that the spinal motion segment under consideration (whether it be one vertebral level or several) is no longer able to fully contribute to global spine stability through normal weight-bearing and therefore excessive reflex muscle activity is required to achieve this stability. The diagnosis of dysfunctional segmental motion may be implied by several diagnostic findings, such as disc interspace degeneration or a fixed lumbar vertebral subluxation on a lateral radiograph, hypermobility of a vertebral segment observed on dynamic radiographs, or by the determination of the instantaneous axis of rotation or the center of rotation.4 There is much debate as to how to precisely manage a dysfunctional motion segment, and there are many schools of thought as to the most appropriate surgical intervention. Treatments range from rigid internal fixation with pedicle screws and interbody fusion to total disc arthroplasty and motion preservation techniques.
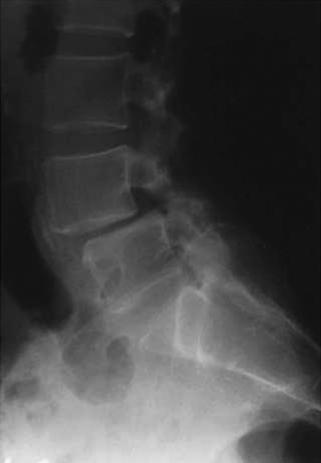
(From Benzel EC: Biomechanics of spine stabilization, New York, 2001, Thieme. Used with permission.)
Spondylolithesis is a term derived from the Greek spondyl [vertebra] and olisthesis [to slip].5 It describes a ventral translation of the cranial vertebra “on” the respective caudal vertebra of a given functional spinal unit. There are two major etiologies of spondylolisthesis: isthmic and degenerative. Isthmic spondylolisthesis is associated with a defect in the pars interarticularis (the isthmus), which is the bony junction of the pedicle, cranial and caudal articular processes, and the lamina. A fibrocartilaginous defect in the pars interarticularis, termed spondylolysis, is present in roughly 6% of the North American population.5 Degenerative spondylolisthesis is associated with arthritic degeneration, or spondylosis, of the facet joints and the intervertebral disc.6 Much like the management of the dysfunctional motion segment, much debate exists as to the proper management of spondylolisthesis. If conservative treatment fails, surgical options range from dorsal bony fusion alone to so-called 360-degree combined dorsal and ventral fusion procedures with interbody graft placement, facet osteotomies and manual reduction, and pedicle screw/rod fixation.
It is widely felt that patients with isolated mechanical low back pain (i.e., without radiculopathy or other signs of neural element compression and without evidence of tumor, infection, or progressive deformity) should only be considered as surgical candidates if a trial of nonoperative management (physical therapy, weight loss) has failed to relieve their symptoms.1,3,5,6 Nearly 90% of all patients with low back pain will experience relief with conservative treatment in 6 weeks to 3 months.1 Furthermore, as the causes of lower back pain are multiple, the mere presence of a spondylolisthesis does not guarantee that the pain will be cured if the spondylolisthesis is corrected.7 Much controversy surrounds the surgical management of isolated nonradicular mechanical back pain. As a general rule, however, initial conservative management of this disease with physical rehabilitation will be successful in the majority of patients. For the small subset of patients in whom initial conservative management has failed, surgery should then be considered.
Isolated Mechanical Low Back Pain without Spondylolisthesis: Surgical Indications
Chou et al.8 recently reviewed 20 randomized trials evaluating surgery for nonradicular low back pain. Of these trials, 4 were prospective, randomized trials evaluating spinal fusion versus nonsurgical therapy for patients with moderate to severe low back pain for at least 1 year and in whom conservative medical management had failed.9–12 Among these trials were the Swedish Lumbar Spine Study,12 which showed a statistically significant improvement in patient satisfaction, pain relief, Oswestry Disability Index (ODI) scores, and Visual Analog Scale (VAS) scores, and the Medical Research Council Spine Stabilization Trial,11 which showed a statistically significant improvement in ODI scores. The 2 other studies did not show any statistically significant differences between surgery and nonsurgical therapy at 1 year, although 1 study did show a trend toward better ODI scores and back pain scores in the surgical group.9,10 Reviews of other similar studies by Gibson et al. showed equally mixed results between surgical and nonsurgical groups.13 A possible explanation for this is the lack of standardization of nonsurgical management across the different studies.8 Resnick et al al.14 performed a meta-analysis of several prospective and retrospective studies, examining the performance of lumbar fusion in patients with intractable low back pain without stenosis or spondylolisthesis. They reviewed many of the same studies as Chou et al. and concluded that Class I medical evidence exists in support of the use of lumbar fusion as a treatment standard for the management of isolated low back pain intractable to the best medical management in carefully selected patients.14 Patients in all 4 studies received a variety of lumbar fusion procedures ranging from dorsolateral fusion alone to interbody fusion supplemented with pedicle screws and posterior noninstrumented fusion (PLF).
Isolated Mechanical Low Back Pain with Spondylolisthesis: Surgical Indications
Matsunaga et al.15 reviewed the outcomes of 145 patients with degenerative spondylolisthesis managed nonsurgically. They found that most of their patients noticed an improvement in their back pain over time, only about 30% of their patients suffered progressive slippage, and 76% of patients who were neurologically intact remained that way. Jacobs et al.16 reviewed eight prospective, randomized trials and five cohort studies’ management of low-grade adult isthmic spondylolisthesis. They concluded that evidence exists in support of fusion over conservative management for low-grade isthmic spondylolisthesis; however, this should not be considered the gold standard of management. Furthermore, they found no difference in outcomes or complications between circumferential fusion and PLF. Resnick et al.17 reviewed 85 studies examining fusion and degenerative spondylolisthesis, among them the landmark study by Herkowitz and Kurz,18 which provided Class II evidence in support of decompression in conjunction with fusion in patients with lumbar stenosis with spondylolisthesis versus decompression alone. They concluded that lumbar decompression with fusion is effective in the management of spinal stenosis associated with spondylolisthesis. Sengupta and Herkowitz19 listed three indications for surgical management of degenerative lumbar spondylolisthesis: persistent back pain and/or leg pain refractory to 3 months of the best medical management and significant reduction of quality of life (QOL), progressive neurologic deficit, and bladder or bowel symptoms. The presence of spinal stenosis with spondylolisthesis is important; several trials (including the much-discussed Spine Patient Outcomes Research Trial [SPORT]) indicate that surgical management is clearly helpful if medical management fails.20
Combined Ventral and Dorsal Approach
Mechanical low back pain is thought to be, in part, a result of intervertebral disc degeneration and dehydration of the nucleus pulposus, which results in altered distribution of load across the vertebral endplates.21 This explains why pain is worsened with daily activity (loading) and improved with rest (unloading). Although it is commonly thought that spinal instability in mechanical back pain and degenerative spondylolisthesis is a result of abnormal translation and motion at the affected segment, there is minimal evidence to support this.19 As a matter of fact, it has been shown that motion at the diseased segment is actually decreased in patients with degenerative spondylolisthesis.22 However, proponents of the combined approach hold that removal of the pain-generating disc and solid support of the anterior column with an interbody graft combined with posterior column fusion (with or without pedicle screws) provides the best chance for spinal fusion and optimal outcomes when compared to PLF alone.
Resnick et al.23–25 performed meta-analyses to study the use of fusion as an adjunct to decompression in patients with low back pain, with and without spondylolisthesis. Based on the medical evidence they analyzed, they concluded that PLF and interbody fusion (via TLIF, PLIF, or ALIF) should be considered as treatment options in patients with low back pain and degenerative disc disease at one or two levels. Interbody techniques are associated with higher fusion rates when compared to PLF alone, although the medical evidence supporting this statement is marginal. Furthermore, they state that Class II evidence exists to suggest that outcomes are improved in those patients who receive an interbody graft, and no Class I or II evidence exists suggesting worse outcomes with interbody grafts. They do stress that complication rates are significantly higher with the combined approach, and there is no evidence to support the position that outcomes are improved with the addition of a PLF to an ALIF or ALIF with pedicle screws, as this will simply add to morbidity and cost.
Kwon et al.26 performed a systematic literature review to examine outcomes in management of adult low-grade isthmic spondylolisthesis. They examined 34 studies, 4 of which were randomized, controlled studies. They found that patients undergoing a combined approach attained significantly higher fusion rates (98.2%) when compared to ventral (74%) or dorsal (83.3%) procedures alone, and had a higher rate of successful clinical outcome (86.4%) versus dorsal (74.8%) or ventral (89.6%) procedures. In many of the studies that they reviewed, there seemed to be a strong correlation between achievement of a solid fusion and successful clinical outcome. The authors do state that their data was based on pooled results from many different studies (many of which were retrospective) and therefore may be subject to a certain level of bias. Especially alarming is the fact that two of the better randomized studies showed no difference between the two groups.
Swan et al.27 performed a prospective randomized trial comparing single-level PLF to the combined approach in patients with unstable isthmic spondylolisthesis. They found superior clinical and fusion outcomes in patients undergoing a circumferential procedure compared to the PLF group. There was also a higher rate of complications in the circumferential group.
Videbaek et al.28 performed a prospective, randomized study of circumferential fusion versus instrumented PLF in patients with severe chronic low back pain. They found significantly better clinical outcomes in the circumferential group when compared to the instrumented PLF group at 5 to 9 years after surgery.
Combined Dorsal and Ventral Techniques
Transforaminal Lumbar Interbody Fusion
Several prospective and retrospective studies have examined outcomes of both open and MIS-TLIF in the management of degenerative lumbar spine disease with and without spondylolisthesis. Shunwu et al.29 found that MIS-TLIF resulted in reduced blood loss, fewer transfusions, less postoperative back pain, lower amounts of muscle breakdown, shorter time of hospital stay, greater improvements in ODI and VAS scores, and shorter time to ambulation despite shorter operating room times in the open group. Fusion rates were similar in both groups. Other studies confirm the reliability of TLIF in improving patient clinical outcomes, maintaining excellent fusion rates, and improving pain index scores.30–34 Kwon et al.35 evaluated postoperative radiographs from 35 consecutive patients who underwent TLIF and pedicle screw placement for adult isthmic spondylolisthesis. They found that TLIF reduced ventrolisthesis (average slip angle was not altered), increased disc height, and restored lordosis across the affected disc space with ventral placement of the interbody cage.
Anterior Lumbar Interbody Fusion
ALIF is performed via a ventral incision and extraperitoneal approach to the ventral lumbar spine. It is sometimes performed with the assistance of an approach surgeon (usually a general surgeon). This approach allows excellent visualization of the disc space and a large surface area for placement of a wide cage (theoretically increasing the chances of attaining bony fusion). If dorsal instrumentation is desired, a separate procedure must be performed. An MIS-ALIF performed through a much smaller ventral incision is another option. ALIF has been shown to have a high rate of success in attaining bony fusion and relieving back pain, especially in patients with pseudarthrosis after prior PLF.36
Ishihara et al.37 reviewed 35 patients who underwent stand-alone ALIF for treatment of isthmic spondylolisthesis. Low back pain scores worsened after 5 years, listhesis and decreased disc height recurred after bony fusion, and fusion rates were 83%. Most other studies have demonstrated higher fusion rates (92–97%)38 and high levels of patient satisfaction and good clinical outcomes with the addition of percutaneous pedicle screw fixation to ALIF.39–41 Kim et al.42 retrospectively reviewed 48 patients who underwent MIS-ALIF with percutaneous pedicle screws and compared them to 46 patients who underwent MIS-TLIF for adult isthmic spondylolisthesis. They concluded that at roughly 30 months postoperation, the MIS-ALIF group had a slightly higher rate of radiologic fusion and more optimal restoration of sagittal alignment; however, both groups showed similar clinical outcomes.
Posterior Lumbar Interbody Fusion
Popularized by Cloward in the 1950s, PLIF is a somewhat older technique, yet it is still proven to be useful in attaining solid bony fusion and producing good patient outcomes. In his prospective study of 32 patients with degenerative spondylolisthesis who underwent PLIF using an insert and rotate technique, Sears43 describes improvement in back pain scores, 92% correction of slip, increase of focal lordosis of 26%, and minimal complications from 12 to 32 months.
Despite the reported success rates with PLIF, it has recently fallen out of favor with many surgeons due to its increased invasiveness and associated morbidity when compared to the less invasive TLIF or MIS-TLIF. Nevertheless, it remains a good option for reduction of spondylolisthesis and restoration of lordosis in patients with low back pain. Yan et al.44 retrospectively reviewed 91 patients who underwent PLIF with pedicle fixation and compared them to 96 patients who underwent TLIF and pedicle screw fixation for adult degenerative spondylolisthesis. They concluded that both PLIF and TLIF achieve statistically similar good clinical outcomes and both are equally safe and effective; however, the TLIF is simpler to perform and may quicken patient recovery since it is somewhat less invasive and spares the dorsal interspinous tension band.
Lateral Transpsoas Interbody Fusion
LTIF is the newest of the techniques of interbody fusion. It is a minimally invasive technique that allows lumbar interbody fusion via a retroperitoneal direct lateral approach. Because it is such a new technique, long-term results have yet to be published. It is a relatively risky approach because the surgical route traverses the psoas muscle ventral to the lumbar plexus. It also places at risk structures ventral to the disc space such as the vena cava and the aorta. Evidence suggests that the highest risk of iatrogenic injury to the lumbar plexus is at the L4-5 level, which is commonly operated on in patients with spondylolisthesis and/or degenerative disc disease with back pain.45 Cappuccino et al.46 performed an in vitro biomechanical analysis of a lateral transpsoas implant and pedicle screw construct. They concluded that the LTIF construct resulted in reduction of segmental range of motion greater in magnitude than either ALIF or TLIF.
Circumferential (360-degree) Fusion
With regards to the combined dorsal and ventral approach, a 360-degree (ventral and dorsal) fusion is yet another option. It entails ventral discectomy and cage placement, dorsal column fusion (usually via PLF), and dorsal instrumentation (usually via pedicle screws). Some surgeons contend that 360-degree fusion results in significantly higher fusion rates than the aforementioned procedures.27 Kim et al.47 compared instrumented ALIF to 360-degree fusion for the treatment of low-grade isthmic spondylolisthesis. They concluded that ALIF is at least as effective as 360-degree fusion in terms of clinical outcomes and is superior with regard to reducing hospital stay and morbidity. Schofferman et al.48 conducted a prospective, randomized trial comparing instrumented ALIF to instrumented ALIF plus PLF. They found that both procedures reduced pain and improved function, but there was less morbidity, shorter hospital stay, and less utilization of health care resources in the instrumented ALIF group. Some studies, however, have demonstrated that 360-degree fusions may be helpful for a certain subset of patients.49,50
Fairbank J., Frost H., Wilson-MacDonald J., et al. Randomised controlled trial to compare surgical stabilisation of the lumbar spine with an intensive rehabilitation programme for patients with chronic low back pain: the MRC spine stabilisation trial. BMJ. 2005;330:1233.
Fritzell P., Hagg O., Wessberg P., Nordwall A. 2001 Volvo Award Winner in Clinical Studies: Lumbar fusion versus nonsurgical treatment for chronic low back pain: a multicenter randomized controlled trial from the Swedish Lumbar Spine Study Group. Spine (Phila Pa 1976). 2001;26:2521-2542.
Herkowitz H.N., Kurz L.T. Degenerative lumbar spondylolisthesis with spinal stenosis. A prospective study comparing decompression with decompression and intertransverse process arthrodesis. J Bone Joint Surg [Am]. 1991;73:802-808.
Resnick D.K., Choudhri T.F., Dailey A.T., et al. Guidelines for the performance of fusion procedures for degenerative disease of the lumbar spine. Part 9: fusion in patients with stenosis and spondylolisthesis. J Neurosurg Spine. 2005;2:679-685.
Sengupta D.K., Herkowitz H.N. Degenerative spondylolisthesis: review of current trends and controversies. Spine (Phila Pa 1976). 2005;30:S71-S81.
1. Madigan L., Vaccaro A.R., Spector L.R., Milam R.A. Management of symptomatic lumbar degenerative disk disease. J Am Acad Orthop Surg. 2009;17:102-111.
2. Frymoyer J.W. Back pain and sciatica. N Engl J Med. 1988;318:291-300.
3. Middleton K., Fish D.E. Lumbar spondylosis: clinical presentation and treatment approaches. Curr Rev Musculoskelet Med. 2009;2:94-104.
4. Benzel E.C. Biomechanics of spine stabilization. Rolling Meadows, IL: American Association of Neurological Surgeons; 2001.
5. Jones T.R., Rao R.D. Adult isthmic spondylolisthesis. J Am Acad Orthop Surg. 2009;17:609-617.
6. Kalichman L., Kim D.H., Li L., et al. Spondylolysis and spondylolisthesis: prevalence and association with low back pain in the adult community-based population. Spine (Phila Pa 1976). 2009;34:199-205.
7. Denard P.J., Holton K.F., Miller J., et al. Back pain, neurogenic symptoms, and physical function in relation to spondylolisthesis among elderly men. Spine J. 2010;10:865-873.
8. Chou R., Baisden J., Carragee E.J., et al. Surgery for low back pain: a review of the evidence for an American Pain Society Clinical Practice Guideline. Spine (Phila Pa 1976). 2009;34:1094-1109.
9. Brox J.I., Reikeras O., Nygaard O., et al. Lumbar instrumented fusion compared with cognitive intervention and exercises in patients with chronic back pain after previous surgery for disc herniation: a prospective randomized controlled study. Pain. 2006;122:145-155.
10. Brox J.I., Sorensen R., Friis A., et al. Randomized clinical trial of lumbar instrumented fusion and cognitive intervention and exercises in patients with chronic low back pain and disc degeneration. Spine (Phila Pa 1976). 2003;28:1913-1921.
11. Fairbank J., Frost H., Wilson-MacDonald J., et al. Randomised controlled trial to compare surgical stabilisation of the lumbar spine with an intensive rehabilitation programme for patients with chronic low back pain: the MRC spine stabilisation trial. BMJ. 2005;330:1233.
12. Fritzell P., Hagg O., Wessberg P., Nordwall A. 2001 Volvo Award Winner in Clinical Studies: Lumbar fusion versus nonsurgical treatment for chronic low back pain: a multicenter randomized controlled trial from the Swedish Lumbar Spine Study Group. Spine (Phila Pa 1976). 2001;26:2521-2542.
13. Gibson J.N., Grant I.C., Waddell G. The Cochrane review of surgery for lumbar disc prolapse and degenerative lumbar spondylosis. Spine (Phila Pa 1976). 1999;24:1820-1832.
14. Resnick D.K., Choudhri T.F., Dailey A.T., et al. Guidelines for the performance of fusion procedures for degenerative disease of the lumbar spine. Part 7: intractable low-back pain without stenosis or spondylolisthesis. J Neurosurg Spine. 2005;2:670-672.
15. Matsunaga S., Ijiri K., Hayashi K. Nonsurgically managed patients with degenerative spondylolisthesis: a 10- to 18-year follow-up study. J Neurosurg. 2000;93:194-198.
16. Jacobs W.C., Vreeling A., De Kleuver M. Fusion for low-grade adult isthmic spondylolisthesis: a systematic review of the literature. Eur Spine J. 2006;15:391-402.
17. Resnick D.K., Choudhri T.F., Dailey A.T., et al. Guidelines for the performance of fusion procedures for degenerative disease of the lumbar spine. Part 9: fusion in patients with stenosis and spondylolisthesis. J Neurosurg Spine. 2005;2:679-685.
18. Herkowitz H.N., Kurz L.T. Degenerative lumbar spondylolisthesis with spinal stenosis. A prospective study comparing decompression with decompression and intertransverse process arthrodesis. J Bone Joint Surg [Am]. 1991;73:802-808.
19. Sengupta D.K., Herkowitz H.N. Degenerative spondylolisthesis: review of current trends and controversies. Spine (Phila Pa 1976). 2005;30:S71-S81.
20. Weinstein J.N., Lurie J.D., Tosteson T.D., et al. Surgical compared with nonoperative treatment for lumbar degenerative spondylolisthesis. 4-year results in the Spine Patient Outcomes Research Trial (SPORT) randomized and observational cohorts. J Bone Joint Surg [Am]. 2009;91:1295-1304.
21. McNally D.S., Shackleford I.M., Goodship A.E., Mulholland R.C. In vivo stress measurement can predict pain on discography. Spine (Phila Pa 1976). 1996;21:2580-2587.
22. McGregor A.H., Anderton L., Gedroyc W.M., et al. The use of interventional open MRI to assess the kinematics of the lumbar spine in patients with spondylolisthesis. Spine (Phila Pa 1976). 2002;27:1582-1586.
23. Resnick D.K., Choudhri T.F., Dailey A.T., et al. Guidelines for the performance of fusion procedures for degenerative disease of the lumbar spine. Part 10: Fusion following decompression in patients with stenosis without spondylolisthesis. J Neurosurg Spine. 2005;2:686-691.
24. Resnick D.K., Choudhri T.F., Dailey A.T., et al. Guidelines for the performance of fusion procedures for degenerative disease of the lumbar spine. Part 11: Interbody techniques for lumbar fusion. J Neurosurg Spine. 2005;2:692-699.
25. Resnick D.K., Choudhri T.F., Dailey A.T., et al. Guidelines for the performance of fusion procedures for degenerative disease of the lumbar spine. Part 12: Pedicle screw fixation as an adjunct to posterolateral fusion for low-back pain. J Neurosurg Spine. 2005;2:700-706.
26. Kwon B.K., Hilibrand A.S., Malloy K., et al. A critical analysis of the literature regarding surgical approach and outcome for adult low-grade isthmic spondylolisthesis. J Spinal Disord Tech. 2005;18(Suppl):S30-S40.
27. Swan J., Hurwitz E., Malek F., et al. Surgical treatment for unstable low-grade isthmic spondylolisthesis in adults: a prospective controlled study of posterior instrumented fusion compared with combined anterior-posterior fusion. Spine J. 2006;6:606-614.
28. Videbaek T.S., Christensen F.B., Soegaard R., et al. Circumferential fusion improves outcome in comparison with instrumented posterolateral fusion: long-term results of a randomized clinical trial. Spine (Phila Pa 1976). 2006;31:2875-2880.
29. Shunwu F., Xing Z., Fengdong Z., Xiangqian F. Minimally invasive transforaminal lumbar interbody fusion for the treatment of degenerative lumbar diseases. Spine (Phila Pa 1976). 2010;35:1615-1620.
30. Xu H., Tang H., Li Z. Surgical treatment of adult degenerative spondylolisthesis by instrumented transforaminal lumbar interbody fusion in the Han nationality. J Neurosurg Spine. 2009;10:496-499.
31. Houten J.K., Post N.H., Dryer J.W., Errico T.J. Clinical and radiographically/neuroimaging documented outcome in transforaminal lumbar interbody fusion. Neurosurg Focus. 2006;20:E8.
32. Lauber S., Schulte T.L., Liljenqvist U., et al. Clinical and radiologic 2-to 4-year results of transforaminal lumbar interbody fusion in degenerative and isthmic spondylolisthesis grades 1 and 2. Spine (Phila Pa 1976). 2006;31:1693-1698.
33. Hackenberg L., Halm H., Bullmann V., et al. Transforaminal lumbar interbody fusion: a safe technique with satisfactory 3- to 5-year results. Eur Spine J. 2005;14:551-558.
34. Salehi S.A., Tawk R., Ganju A., et al. Transforaminal lumbar interbody fusion: surgical technique and results in 24 patients. Neurosurgery. 2004;54:368-374.
35. Kwon B.K., Berta S., Daffner S.D., et al. Radiographic analysis of transforaminal lumbar interbody fusion for the treatment of adult isthmic spondylolisthesis. J Spinal Disord Tech. 2003;16:469-476.
36. Barrick W.T., Schofferman J.A., Reynolds J.B., et al. Anterior lumbar fusion improves discogenic pain at levels of prior posterolateral fusion. Spine (Phila Pa 1976). 2000;25:853-857.
37. Ishihara H., Osada R., Kanamori M., et al. Minimum 10-year follow-up study of anterior lumbar interbody fusion for isthmic spondylolisthesis. J Spinal Disord. 2001;14:91-99.
38. Lee S.H., Choi W.G., Lim S.R., et al. Minimally invasive anterior lumbar interbody fusion followed by percutaneous pedicle screw fixation for isthmic spondylolisthesis. Spine J. 2004;4:644-649.
39. Anderson D.G., Sayadipour A., Shelby K., et al. Anterior interbody arthrodesis with percutaneous posterior pedicle fixation for degenerative conditions of the lumbar spine. Eur Spine J. 2011;20:1323-1330.
40. Spruit M., van Jonbergen J.P., de Kleuver M. A concise follow-up of a previous report: posterior reduction and anterior lumbar interbody fusion in symptomatic low-grade adult isthmic spondylolisthesis. Eur Spine J. 2005;14:828-832.
41. El Masry M.A., Badawy W.S., Rajendran P., Chan D. Combined anterior interbody fusion and posterior pedicle screw fixation in patients with degenerative lumbar disc disease. Int Orthop. 2004;28:294-297.
42. Kim J.S., Kang B.U., Lee S.H., et al. Mini-transforaminal lumbar interbody fusion versus anterior lumbar interbody fusion augmented by percutaneous pedicle screw fixation: a comparison of surgical outcomes in adult low-grade isthmic spondylolisthesis. J Spinal Disord Tech. 2009;22:114-121.
43. Sears W. Posterior lumbar interbody fusion for degenerative spondylolisthesis: restoration of sagittal balance using insert-and-rotate interbody spacers. Spine J. 2005;5:170-179.
44. Yan D.L., Pei F.X., Li J., Soo C.L. Comparative study of PLIF and TLIF treatment in adult degenerative spondylolisthesis. Eur Spine J. 2008;17:1311-1316.
45. Kepler C.K., Bogner E.A., Herzog R.J., Huang R.C. Anatomy of the psoas muscle and lumbar plexus with respect to the surgical approach for lateral transpsoas interbody fusion. Eur Spine J. 2011;20:550-556.
46. Cappuccino A., Cornwall G.B., Turner A.W., et al. Biomechanical analysis and review of lateral lumbar fusion constructs. Spine (Phila Pa 1976). 2010;35:S361-S367.
47. Kim J.S., Kim D.H., Lee S.H., et al. Comparison study of the instrumented circumferential fusion with instrumented anterior lumbar interbody fusion as a surgical procedure for adult low-grade isthmic spondylolisthesis. World Neurosurg. 2010;73:565-571.
48. Schofferman J., Slosar P., Reynolds J., et al. A prospective randomized comparison of 270 degrees fusions to 360 degrees fusions (circumferential fusions). Spine (Phila Pa 1976). 2001;26:E207-E212.
49. Suratwala S.J., Pinto M.R., Gilbert T.J., et al. Functional and radiological outcomes of 360 degrees fusion of three or more motion levels in the lumbar spine for degenerative disc disease. Spine (Phila Pa 1976). 2009;34:E351-E358.
50. Remes V., Lamberg T., Tervahartiala P., et al. Long-term outcome after posterolateral, anterior, and circumferential fusion for high-grade isthmic spondylolisthesis in children and adolescents: magnetic resonance imaging findings after average of 17-year follow-up. Spine (Phila Pa 1976). 2006;31:2491-2499.
Total Disc Arthroplasty
Total disc arthroplasty is an exciting and rapidly evolving technology for the treatment of spinal disc disease. This procedure has the potential to maintain a functional spinal motion segment while avoiding long-term consequences of spinal fusion such as adjacent segment degeneration. Spinal interbody fusion converts a mobile three-joint complex into a fixed, nonfunctional spinal unit.1 The abnormal loading that results may create mechanical instability or accelerated degenerative changes at adjacent spinal levels. The ideal artificial disc device would restore physiologic function of a diseased spinal segment by mimicking natural spinal kinematics, maintaining biomechanical function throughout the entire spine, replicating the motion and elasticity of the natural disc, and providing balanced and integrated motion of the spinal unit. On top of these characteristics the implant needs to be highly durable and biocompatible.
Discectomy
Discectomy is a well established method for treating radicular pain; however, its success ranges between 48% and 89% in the literature.2 Long-term follow-up of patients who underwent lumbar discectomy reveals that 50% to 60% will suffer significant back pain after 10 years and 20% to 30% will develop recurrent radicular pain.2 These outcomes are clearly suboptimal and likely due to a combination of ongoing degeneration, segmental instability, spinal stenosis, and recurrent disc herniation.
Reasons for long-term failure of surgical treatment include failure to address the primary pain generator and collapse of the disc space. Disc space collapse can lead to a decrease in the diameter of neural foramina, alterations in facet loading, and disruption of sagittal balance. The abnormal loading of the joints results in biochemical changes in the cartilage that can affect both the diseased level and adjacent segments. Chronic abnormal loading can also trigger hypertrophic changes of the articular processes. The disc space narrowing has the potential to cause rostral and anterior displacement of the superior facet joint, which may compress the exiting nerve root as it traverses the foramen.2
Ongoing pain may also result from destabilization of the spinal segment after discectomy. Motion segment stiffness is inversely related to the amount of nuclear material that is removed, and removal of even a portion of the disc has been associated with hypermobility in the sagittal, coronal, and axial planes.2,3 This instability can eventually extend to the rostral adjacent level and exacerbate the symptoms.2,4
Arthrodesis
Arthrodesis eliminates segmental instability, preserves sagittal balance, stops further degeneration at the treated level, and is able to restore disc space height with an interbody graft. For these reasons, it has become the primary treatment for symptomatic spondylosis. Positive results have been reported in 52% to 100% of patients who underwent anterior lumbar interbody fusion (ALIF) and in 50% to 95% of those who underwent posterior lumbar interbody fusion (PLIF).2,5,6 Despite these results, spinal arthrodesis has potential negative consequences. Fusing a spinal segment disrupts the biomechanical mobility of adjacent levels and potentially the entire spinal region. Over time this may lead to symptomatic hypertrophic facet arthropathy, spinal stenosis, disc degeneration, and osteophyte formation.2,7 Long-term follow-up of lumbar fusion patients has demonstrated that approximately 50% eventually develop postoperative low back pain, and approximately 15% may require additional surgery.2,7 In the cervical spine, hypermobility at segments adjacent to an anterior fusion construct has been repeatedly demonstrated and likely accelerates the degenerative process. Long-term follow-up studies show radiographic adjacent-level disease in up to 92% of patients after anterior cervical fusion.8 Although these findings do not correlate well with clinical symptoms, the accelerated spondylotic changes are becoming an increasing concern with this procedure.
Other problems associated with fusions include the potential for fixation with suboptimal sagittal balance, morbidities associated with autograft harvest, and disruption of muscular and ligamentous attachments. Although the loss of mobility is also a drawback, this has a greater functional impact in the cervical than in the lumbar spine.9
Arthroplasty
The concept of reconstructing a functional motion segment after decompressing impinged neural elements is not new. The first attempts at disc arthroplasty began more than 40 years ago.2,10 Ulf Fernstrom made the first attempt to reconstruct the disc space by using stainless steel balls in both the lumbar and cervical spine. He reported his results in 1966 but unfortunately this reconstruction method had a high rate (88%) of subsidence.10 Other early attempts were also associated with unacceptable complication rates involving migration and subsidence of the device as well as adjacent-segment hypermobility.11 As advancements have been made in our understanding of the degenerative process, the biomechanics of motion, and the biomaterials available for design, the possibility of replacing a degenerated disc with a functional, stable joint has become a reality.
Cervical
Anterior cervical discectomy and fusion (ACDF) is a well-established and widely used technique for treating patients with degenerative disease of the cervical spine. Because outcomes after ACDF are excellent for treating radiculopathy and myelopathy, it may seem difficult to justify a change in surgical strategy with the use of disc arthroplasty.2 Fusing a spinal segment in the cervical spine, however, has an impact on functional mobility, and accumulating evidence suggests that arthrodesis increases stress and accelerates degenerative changes at the adjacent spinal levels.
The risk of adjacent-level disease in the cervical spine has been estimated at 2.9% per year, with a reported symptomatic prevalence between 25% and 50% after long-term follow-up studies.12,13 Retrospective data reveal reoperation rates as high as 25.6% after the development of adjacent-level disease in patients who have undergone cervical fusion.13 Although other factors such as the natural history of the degenerative process play a role, it is likely that the increased strain and pathologic loads on spinal segments adjacent to the fused level are the most influential contributors to this process.
In biomechanical studies, cervical arthroplasty maintains motion and mechanics of the spinal segment within physiologic ranges at the treated level and decreases stress on adjacent levels.14,15 Many initial aspirations for the success of this technology focused on prevention of adjacent-level disease through a gradual (instead of sudden) loss of mobility at the treated segment, and eventual ankylosis was expected. Although this does occur in a portion of patients who undergo cervical arthroplasty, ongoing studies are increasingly demonstrating preservation of functional motion in most patients over the long term. Further long-term data will provide more information on this benefit and definitively demonstrate whether arthroplasty will significantly reduce the prevalence of clinically relevant adjacent-segment disease.
Advantages and Indications
Disc arthroplasty is advantageous over arthrodesis because of its ability to restore spinal segment mobility by mimicking the kinematics of the intervertebral disc while maintaining stability and normal physiologic loads (Fig. 224-3).
In terms of neurologic outcome, pain resolution, and functional mobility, arthroplasty offers equivalent if not superior results to segmental fusion in the cervical spine. Other advantages include the elimination of the risk for developing pseudarthrosis. The risk of pseudarthrosis after a fusion procedure is estimated to be as high as 10% and can result in chronic pain and pathologic loading of surrounding joints.5,6 Many advantages that have been associated with fusion techniques, such as elimination of future disc herniation, are also expected with arthroplasty.16 Expected positive outcomes similarly include a reduction in development of spondylosis, stenosis, and instability at the treated segment. The restoration of intervertebral height will increase foraminal diameter and diminish the occurrence of nerve root compression, and the morbidities associated with autograft harvest are avoided entirely.
Disadvantages
Not all patients with spinal disc disease are candidates for arthroplasty. Contraindications are numerous and include segmental instability, prior trauma resulting in compromise of bony structure, severe spondylosis with absence of motion and bridging osteophytes, infection, and presence of other spinal diseases, such as osteoporosis, rheumatoid arthritis, and known malignancy involving the spine. The presence of significant deformity such as scoliosis, spondylolisthesis, or kyphosis, is also a contraindication.16,17 Patients who have preoperative hypermobility that exceeds 11 degrees of flexion and extension are likely poor candidates for arthroplasty, because long-term stability may not be successfully maintained and subluxation or dislocation of the disc replacement can occur.1 A known allergy to any of the materials found in the implant eliminates this procedure as a surgical option, and a potential reaction to one of these elements is another disadvantage of the procedure.
Cadaveric studies assessing the facet force pressures before and after both cervical arthroplasty and cervical fusion found that although the changes in facet force were minimal in flexion, lateral bending, and rotation, they were significantly increased in extension at the treated level in the arthroplasty group.18 Findings such as these have precluded patients with facet joint disease from being acceptable candidates for disc arthroplasty and have raised concerns about escalation of degenerative changes in the normal facet joints in those patients who have undergone the procedure.
Fundamental Concepts
Biomechanics
The etiology of adjacent segment disease is multifactorial, but one element is felt to be increased pressure in the intervertebral disc caused by load alterations after anterior cervical fusion.18,19 Biomechanical studies measuring this phenomenon have found that adjacent disc pressures are elevated after fusion, especially in flexion and extension.18,20 After a lumbar fusion, the changes in intradiscal pressure seen in adjacent levels are even more pronounced, with elevations increasing relative to the length of the construct. In contrast, after a disc arthroplasty in the cervical spine, physiologic intradiscal pressures are maintained at adjacent levels.20 Biomechanical studies involving the lumbar spine have directly compared arthroplasty with fused and normal spinal segments. Arthroplasty maintains or reduces adjacent-level mobility and intradiscal pressure when compared to the intact spine. Fused levels are associated with elevated intradiscal pressures as well as compensatory hypermobility at both rostral and caudal adjacent levels.21–23
Alterations in intradiscal pressures are important given their relationship to the disc degeneration process. Because the intervertebral disc lacks a true blood supply, the disc depends on obtaining nutrients from diffusion through the extracellular matrix of peripheral blood vessels and vertebral endplates. Elevated pressure in the disc will alter the diffusion characteristics of small solutes such as oxygen, glucose, and sulfate, leading to an accumulation of waste products. This then leads to increased lactate levels, decreased pH, impaired metabolism, and eventual cell death. Impaired nutrition of the disc has been identified as the most significant cause of disc degeneration.18 As the nucleus of the disc degenerates, compressive load is transferred to the anulus fibrosus, disc height is lost, and loads throughout the spinal segment are altered.20
Normal physiologic load on the facet joints varies with the form of motion. Large loads are carried in extension, lateral bending, rotation, and compression. The facets are unloaded in flexion. In the lumbar spine, segmental fusion leads to decreased strain on the facets at the fused level and increased strain on facets at the adjacent caudal and rostral levels.17,18 In the cervical spine, segmental fusion similarly leads to decreased strain on the facets at the fused level; however, increased strain on adjacent-level facets is only seen in extension. After cervical arthroplasty, however, an increased strain on the facet joints is seen at the treated and adjacent levels when in extension. This increased load on adjacent-level facets in extension is more pronounced following fusion than following arthroplasty. Loads in flexion, rotation, compression, and lateral bending are unchanged after either procedure.18 The increased load on the facets at the treated level following an arthroplasty is likely due to the biomechanical design of the implant. Most artificial discs have an instantaneous axis of rotation located in the posterior portion of the device. This allows for restoration of the lordotic curve and will consequently increase the loading of the posterior elements.24
Many biomechanical studies have demonstrated the capacity for a cervical artificial disc to preserve mobility that is comparable to an intact spinal segment.18,20,24 Evaluation of sagittal rotation, horizontal translation, change in disc height, and center of rotation have demonstrated the preservation of preoperative kinematics following arthroplasty.20 Artificial discs in the lumbar spine have demonstrated restoration of physiologic motion at the operative level with flexion and lateral bending. An increase in motion is seen in axial rotation, however, and in some studies increased motion is seen during extension as well. The exact limitations of motion will vary depending on the device being assessed. Motion at adjacent levels is within physiologic range after arthroplasty and significantly increased after fusion in all planes of motion.21–23 The primary goal when designing most devices is to replicate full biomechanical function of a normal disc, including independent translation and rotation in all three planes of motion.
The biomechanical design and testing of disc arthroplasty devices has undergone extensive evolution and continues to improve as the procedure gains popularity. Material designs are typically a combination of metal, polymer, and ceramic. The devices can be categorized based on the extent of motion they allow and are typically described as constrained, semi-constrained, or unconstrained. Considerations during the construction of these devices include durability, wear properties, stiffness, and imaging compatibility. Thorough testing of these devices must characterize not only the above biomechanical parameters at the treated levels, but also the effect on adjacent segments, coupling patterns, facet loading, intradiscal pressures, and postural changes.11
Nucleus Replacement
Some of the initial efforts at disc restoration focused on replacing the nucleus pulposus. Nucleus replacement is a less invasive option than fusion or total disc replacement for treating degenerative disorders. These devices avoid major disruption of the anulus fibrosus because they can be placed through a small anular window. Advantages to nucleus replacement include multiple approach options, multiple revision options, and minimally invasive technique. Disadvantages include the risk for migration, expulsion, or subsidence. Contraindications to this type of procedure include an intervertebral space less than 5 mm, spondylolisthesis, and presence of Schmorl nodules. These contraindications unfortunately limit the usefulness of this technique as a restorative procedure for spinal degeneration.25
Indications for nucleus replacement can be categorized as prophylactic after microdiscectomy or as a treatment for low back pain in the setting of early to moderate degenerative disease with significant degeneration of the nucleus but an intact anulus. If the nucleus is the presumed pain generator, its removal and subsequent reconstruction with a replacement may improve symptoms and maintain functional motion. Alternatively, loss of disc tissue from herniation or microdiscectomy and its associated intervertebral height loss can accelerate degeneration of the spinal segment. Prophylactic placement of a nucleus prosthetic could restore disc height, absorb physiologic load, and deform with movement while maintaining structural integrity.25
A variety of nucleus replacements are being developed and studied. These devices can be classified as either elastomeric or mechanical. The elastomeric devices frequently consist of hydrogel polymers that absorb water and swell when placed in the intervertebral disc. Nonhydrogel polymers are low-friction materials that carry shock absorption characteristics. This category of implant is theoretically able to recreate the natural function of the normal nucleus pulposus with uniform stress distribution and the ability to absorb shock. Some of these devices are preformed and some are injectable. Preformed devices are deformable and therefore more likely to extrude; however, injectable devices can leak and carry concerns regarding their durability and biocompatibility. Mechanical nucleus replacements are designed with either one or two pieces and are constructed from various biomaterials, including metal, pyrolytic carbon, and PEEK.25 This type of device has more strength and durability than the elastomeric devices; however, it is not able to maintain an even stress distribution and lacks shock absorption capacity. A predisposition to subsidence and expulsion is also problematic given the lack of a mechanical anchor.25,26
The most widely used nuclear replacement is the Prosthetic Disc Nucleus (PDN; Raymedica, Minneapolis, MN). This elastomeric device consists of a hydrogel core constrained within a woven polyethylene jacket. One or two devices are placed in the disc space in an attempt to restore disc space height and replicate physiologic function. The jacket is flexible but inelastic and functions to constrain horizontal and vertical expansion and deformation of the hydrogel core. The core is designed to expand on hydration after implantation. This expansion occurs over 4 to 5 days, during which time the nucleus prosthesis widens the interspace.2,27,28 Biomechanical studies have demonstrated that this device is able to reproduce the range of motion and stiffness of a healthy spinal segment. The neutral zone is also maintained within physiologic limitations, providing stability.28,29 Short-term follow-up studies have demonstrated the effectiveness of this device, with successful maintenance of disc height and symptomatic relief seen in 88% of patients. Long-term follow-up, however, has not been as encouraging. The results are inferior to those of arthrodesis, and the complications rates are high.27,29 The tendency for device migration is especially concerning, and iatrogenic injury to the surrounding ligaments that may constrain the device needs to be minimized. Other preformed elastomeric devices under evaluation include the NeuDisc (Replication Medical, Cranbury, NJ) and the Percutaneous Nucleus Replacement (PNR; TranS1, Wilmington, NC). Injectable devices under evaluation include BioDisc (CryoLife, Atlanta, GA), Hydrafil (Synthes, West Chester, PA), and NuCore (Spine Wave, Shelton, CT).
Placement of a nucleus replacement device should only be undertaken in the setting of a valid indication and after weighing all other treatment options. Limited clinical information is available for these implants and they remain investigational despite some early encouraging results.26,30 Further clinical investigation is needed to determine if this technology has the capacity to slow down degenerative changes in the spine.
Total Disc Arthroplasty
Four artificial discs are currently approved by the Food and Drug Administration (FDA) for single level use in the United States. These include the lumbar Charité (DePuy Spine, Raynham, MA), the cervical and lumbar ProDisc (Synthes, West Chester, PA), the cervical Prestige (Medtronic, Fridley, MN), and the Bryan Cervical Disc (Medtronic). The Kineflex-C and Kineflex-L devices (SpinalMotion, Mountain View, CA), PCM cervical disc (NuVasive, San Diego, CA), and the CerviCore disc (Stryker Spine, Allendale, NJ) are currently being evaluated for FDA approval. Numerous other devices are in various stages of development or clinical use outside of the United States (Table 224-1).
Artificial Disc Replacement Devices
Charité
The Charité device is the artificial disc with the longest history of implantation and clinical investigation.2 Developed by Karin Buttner-Janz and Kurt Schellnack at the Charité University Hospital in Berlin in the mid-1980s, it was approved for use in the United States in 2004. The disc provides unconstrained kinematic motion of the spinal segment and consists of a three-piece articulating device with a biconvex polyethylene core spacer interfacing with two CoCrMo alloy endplates. The device endplates have dorsal and ventral teeth that anchor them into the bony endplates and prevent migration of the implant. Titanium and hydroxyapatite coat these endplates to promote bonding with the vertebral bodies.2,31,32
Five-year follow-up data from the FDA Investigational Device Exemption (IDE) prospective, randomized trial has been published for the Charité artificial disc. Comparison with ALIF was made at a single level from L4-S1. Overall success was 57.8% in the Charité group and 51.2% in the ALIF group. No major complications or neurologic deteriorations were demonstrated. Given the lack of statistical differences in clinical outcome, the device has been deemed a safe and effective alternative to fusion. Encouraging secondary outcomes included greater rates of part- and full-time employment and lower rates of long-term disability among patients who underwent placement of the Charité disc when compared to those who underwent an ALIF procedure.32
Because the Charité was the first implant to be used for total disc replacement, the longest follow-up data is available with this device. Retrospective data over an average of 17 years reveal a 60% rate of ankylosis at the treated level. Those patients who retained mobility had no observable evidence of adjacent-level disease; however, this group was significantly less satisfied with their outcome than those patients who had undergone fusion, and 11% required reoperation. These long-term results did not provide any clear evidence that total disc replacement in the lumbar spine is a superior treatment to spinal arthrodesis.33
ProDisc
Dr. Thierry Marnay developed the ProDisc system in the late 1980s in France. The ProDisc has been designed for both the lumbar spine (ProDisc-L) and the cervical spine (ProDisc-C). This artificial disc is constructed based on a ball-and-socket principle. A three-piece articulating device, it consists of a monoconvex polyethylene inlay and two CrCoMo alloy endplates. The inlay locks into the inferior endplate, and both endplates have a central keel that secures them to the vertebral bodies to prevent migration. Titanium Plasmapore coating of the endplates promotes bony integration. The monoconvex nature of the polyethylene inlay allows the implant to be inserted with less distraction than the Charité device. This inlay is inserted into the caudal endplate, allowing only two moving parts after implantation. This results in semiconstrained kinematic behavior (Fig. 224-4).2
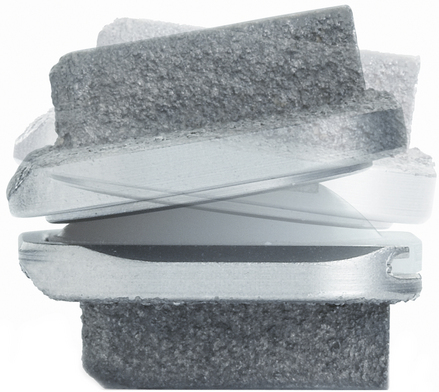
FIGURE 224-4 ProDisc device demonstrating mobility of the endplate over the monoconvex inlay during flexion and extension.
(Courtesy of Synthes Spine.)
The safety and efficacy of the ProDisc-C for treatment of symptomatic cervical disc disease has been established in a prospective, randomized study that compared use of the device to ACDF. Symptomatic outcome is not significantly different between the two procedures; however, after 2-year follow-up the rate of reoperation was significantly lower in the ProDisc-C group (1.8% compared to 8.5%). The use of narcotic pain medication was significantly lower in the disc replacement group as well, and 84.4% of patients with artificial discs retained at least 4 degrees of motion in flexion-extension at the treated segment after 2 years.34,35
A similar study with the ProDisc-L device in the lumbar spine demonstrated safety and efficacy in comparison to circumferential fusion. Lumbar disc arthroplasty was found to have significantly superior results when considering the Visual Analog Scale (VAS) for pain assessment. After a 2-year follow-up, 93.7% of patients retained a normal functional range of motion, with an average of 7.7 degrees mobility on flexion-extension films.36,37
Bryan
The Bryan Cervical Disc was created in the 1990s by the American neurosurgeon Vincent Bryan. This disc is designed to allow for normal, unconstrained physiologic cervical motion. The device consists of a polyurethane nucleus that is shaped to fit between two dome-shaped titanium shells. The shells have a rough-textured coating to encourage osseous ingrowth. A flexible polyurethane membrane surrounds the nucleus and prevents debris migration or ingrowth of connective tissue. A tight fit into a milled concavity is required to prevent migration because no hardware anchors the implant. This device allows for normal cervical range of motion in flexion, extension, lateral bending, axial rotation, and translation as well as providing shock absorption of axial loads (Fig. 224-5).2,38
Prospective, randomized studies have demonstrated that the Bryan disc is safe, effective, and compares favorably to cervical fusion for treatment of single-level disease. Patients who underwent arthroplasty showed significant improvement with the Neck Disability Index and VAS for neck and arm pain and returned to work almost 2 weeks earlier than those who underwent fusion. An average of 7.9 degrees of flexion-extension motion was retained after 2 years.39,40
Prestige
B.H. Cummins created the initial version of this artificial disc at the Frenchay Hospital in Bristol, UK, in 1989. After initial disappointing outcomes, the disc was redesigned and reintroduced after pilot studies demonstrated favorable results. In its modern version, the Prestige cervical disc provides unconstrained motion at the treated spinal segment.11 The initial device was constructed with stainless steel; however, the latest generation is a titanium and ceramic composite that is MRI compatible. A porous titanium plasma spray coating is present to encourage bone ingrowth. The disc consists of two articulating components in a ball-and-trough configuration. A ventral extension of each component anchors into the vertebral body with screws. This implant aims to maintain physiologic degrees of flexion, extension, lateral bending, and rotation (Fig. 224-6).
The prospective, randomized trial for the Prestige cervical disc demonstrated safety, efficacy, and preservation of segmental sagittal motion after 2 years with an average of more than 7 degrees of movement. Clinical outcomes were improved when compared to ACDF, and lower rates of secondary surgeries were seen in the arthroplasty group (Fig. 224-7).41
Complications
Potential complications will always coincide with the development of new operative techniques. Many of the potential adverse events that can occur with cervical or lumbar fusion are also risk factors for arthroplasty. These include CSF leak, postoperative hematoma, dysphagia and hoarseness (cervical spine), and injury to visceral or neurovascular structures. Complications more specific to total disc replacement include intraoperative or delayed migration of the implant, subsidence, device failure leading to segmental hypermobility or increased stiffness, heterotopic ossification, accelerated facet degeneration, and spontaneous fusion. Thus far, major operative and device-related complications have been infrequent. In the lumbar spine, arthroplasty has been associated with a device-related complication rate that ranges from 0 to 6.5%. The total perioperative complication rate after cervical arthroplasty has been reported at 6.2% per treated level.1
Adverse outcomes are frequently associated with inappropriate sizing or poor placement of implants. Overdistraction of the facet joints can lead to postoperative neck pain; careful preoperative assessment of disc space height should guide the surgical plan. Unfortunately, no clear data are available to clarify how much height loss would invalidate arthroplasty as a treatment option. A negative effect on sagittal alignment in the cervical spine has also been observed in scattered cases with reports of postoperative kyphotic deformity. Although this effect could have significant implications for long-term outcomes and rates of adjacent-level disease, no precise avoidance method has been determined. Factors that might affect the sagittal alignment include the angle of disc insertion, bone removal, and endplate preparation.1
Should disc arthroplasty fail, arthrodesis at the affected level is the appropriate treatment. Described revision strategies include fusion with posterior instrumentation while leaving the disc device in place or anterior explantation with conversion to arthrodesis. Success of fusion after removal of the disc prosthesis is slightly better than with posterior fusion alone.42 Appropriate patient selection will help to minimize complications.
Baaj A.B., Uribe J.S., Vale F.L., et al. History of cervical disc arthroplasty. Neurosurg Focus. 2009;27:E10.
Chang U.K., Kim D.H., Lee M.C., et al. Changes in adjacent-level disc pressure and facet joint force after cervical arthroplasty compared with cervical discectomy and fusion. J Neurosurg Spine. 2007;7:33-39.
Geisler F.H., Blumenthal S.L., Guyer R.D., et al. Neurological complications of lumbar artificial disc replacement and comparison of clinical results with those related to lumbar arthrodesis in the literature: results of a multicenter, prospective, randomized investigational device exemption study of Charité intervertebral disc. J Neurosurg Spine. 2004;1:143-154.
Sasso R.C., Smucker J.D., Hacker R.J., Heller J.G. Artificial disc versus fusion: a prospective, randomized study with 2-year follow-up on 99 patients. Spine (Phila Pa 1976). 2007;32:2933-2940.
1. Pickett G.E., Sekhon L.H., Sears W.R., Duggal N. Complications with cervical arthroplasty. J Neurosurg Spine. 2006;4:98-105.
2. Traynelis V.C. Spinal arthroplasty. Neurosurg Focus. 2002;13:10.
3. Goel V.K., Goyal S., Clark C., et al. Kinematics of the whole lumbar spine. Effect of discectomy. Spine (Phila Pa 1976). 1986;11:1008-1012.
4. Tibrewal S.B., Pearcy M.J., Portek I., et al. A prospective study of lumbar spinal movements before and after discectomy using biplanar radiography. Correlation of clinical and radiographic findings. Spine (Phila Pa 1976). 1985;10:455-460.
5. Kuslich S.D., Ulstrom C.L., Griffith S.L., et al. The Babgy and Kuslich method of lumbar interbody fusion. History, techniques, and 2-year follow-up results of a United States prospective, multicenter trial. Spine (Phila Pa 1976). 1998;23:1267-1279.
6. Yuan H.A., Garfin S.R., Dickman C.A., Mardjetko S.M. A historical cohort study of pedicle screw fixation in the thoracic, lumbar, and sacral spinal fusions. Spine (Phila Pa 1976). 1994;19:S2279-S2296.
7. Lehmann T.R., Spratt K.F., Tozzi J.E., et al. Long-term follow-up of lower lumbar fusion patients. Spine (Phila Pa 1976). 1987;12:97-104.
8. Le H., Thongtrangan I., Kim D.H. Historical review of cervical arthroplasty. Neurosurg Focus. 2004;17:E1.
9. Frymoyer J.W., Hanley E.N., Howe J., et al. A comparison of radiographic findings in fusion and nonfusion patients 10 or more years following lumbar disc surgery. Spine (Phila Pa 1976). 1979;4:435-440.
10. Fernstrom U. Arthroplasty with intercorporal endoprosthesis in herniated disc and in painful disc. Acta Chir Scand Suppl. 1966;357:154-159.
11. Baaj A.B., Uribe J.S., Vale F.L., et al. History of cervical disc arthroplasty. Neurosurg Focus. 2009;27:E10.
12. Goffin J., Geusens E., Vantomme N. Long-term follow-up after interbody fusion of the cervical spine. J Spinal Disord Tech. 2004;17:79-85.
13. Hilibrand A.S., Carlson G.D., Palumbo M.A., et al. Radiculopathy and myelopathy at segments adjacent to the site of previous anterior cervical arthrodesis. J Bone Joint Surg [Am]. 1999;81:519-528.
14. DiAngelo D.J., Foley K.T., Morrow B.R., et al. In vitro biomechanics of cervical disc arthroplasty with the ProDisc-C total disc implant. Neurosurg Focus. 2004;17:E7.
15. Puttlitz C.M., Rousseau M.A., Xu Z., et al. Intervertebral disc replacement maintains cervical spine kinetics. Spine (Phila Pa 1976). 2004;29:2809-2814.
16. Geisler F.H., Blumenthal S.L., Guyer R.D., et al. Neurological complications of lumbar artificial disc replacement and comparison of clinical results with those related to lumbar arthrodesis in the literature: results of a multicenter, prospective, randomized investigational device exemption study of Charité intervertebral disc. J Neurosurg Spine. 2004;1:143-154.
17. Little J.S., Ianuzzi A., Chiu J.B., et al. Human lumbar facet joint capsule strains: II. Alteration of strains subsequent to anterior interbody fixation. Spine J. 2004;4:153-162.
18. Chang U.K., Kim D.H., Lee M.C., et al. Changes in adjacent-level disc pressure and facet joint force after cervical arthroplasty compared with cervical discectomy and fusion. J Neurosurg Spine. 2007;7:33-39.
19. Eck J.C., Humphreys S.C., Lim T.H., et al. Biomechanical study on the effect of cervical spine fusion on adjacent-level intradiscal pressure and segmental motion. Spine (Phila Pa 1976). 2002;27:2431-2434.
20. Dmitriev A.E., Cunningham B.W., Hu N., et al. Adjacent level intradiscal pressure and segmental kinematics following a cervical total disc arthroplasty: an in vitro human cadaveric model. Spine (Phila Pa 1976). 2005;30:1165-1172.
21. Cunningham B.W., Gordon J.D., Dmitriev A.E., et al. Biomechanical evaluation of total disc replacement arthroplasty: an in vitro human cadaveric model. Spine (Phila Pa 1976). 2003;28:S110-S117.
22. Hitchon P.W., Eichholz K., Barry C., et al. Biomechanical studies of an artificial disc implant in the human cadaveric spine. J Neurosurg Spine. 2005;2:339-343.
23. Ingalhalikar A.V., Reddy C.G., Lim T.H., et al. Effect of lumbar total disc arthroplasty on the segmental motion and intradiscal pressure at the adjacent level: an in vitro biomechanical study. J Neurosurg Spine. 2009;11:715-723.
24. Pickett G.E., Rouleau J.P., Duggal N. Kinematic analysis of the cervical spine following implantation of an artificial cervical disc. Spine (Phila Pa 1976). 2005;30:1949-1954.
25. Coric D., Mummaneni P. Nucleus replacement technologies. J Neurosurg Spine. 2008;8:115-120.
26. Bertagnoli R., Schonmayr R. Surgical and clinical results with the PDN prosthetic disc-nucleus device. Eur Spine J. 2002;11:S143-S148.
27. Jin D., Qu D., Zhao L., et al. Prosthetic disc nucleus replacement for lumbar disc herniation: preliminary report with 6 months’ follow-up. J Spinal Disord Tech. 2003;16:331-337.
28. Buttermann G.R., Beaubien B.P. Biomechanical characterization of an annulus-sparing spinal disc prosthesis. Spine J. 2009;9:744-753.
29. Ma Y.Z., Xue H.B., Chen X., et al. The mid- or long-term clinical results of prosthetic disc nucleus replacement in the treatment of lumbar disc disease. Ahonghua Wai Ke Za Zhi. 2008;46:350-353.
30. Berlemann U., Schwarzenbach O. An injectable nucleus replacement as an adjunct to microdiscectomy: 2-year follow-up in a pilot clinical study. Eur Spine J. 2009;18:1706-1712.
31. Blumenthal S., McAfee P.C., Guyer R.D., et al. A prospective, randomized multicenter Food and Drug Administration investigational device exemptions study of lumbar total disc replacement with the Charité artificial disc versus lumbar fusion: part I: evaluation of clinical outcomes. Spine (Phila Pa 1976). 2005;30:1565-1575.
32. Guyer R.D., McAfee P.C., Banco R.J., et al. Prospective, randomized, multicenter Food and Drug Administration investigational device exemption study of lumbar total disc replacement with the Charité artificial disc versus lumbar fusion: 5-year follow-up. Spine J. 2009;9:374-386.
33. Putzier M., Funk J.F., Schneider S.V., et al. Charité total disc replaement—clinical and radiographical results after an average follow-up of 17 years. Eur Spine J. 2006;15:183-195.
34. Bertagnoli R., Yue J., Pfeiffer F., et al. Early results after ProDisc-C cervical disc replacement. J Neurosurg Spine. 2005;2:403-410.
35. Murrey D., Janssen M., Delamarter R., et al. Results of the prospective, randomized, controlled multicenter Food and Drug Administration investigational device exemption study of the ProDisc-C total disc replacement versus anterior discectomy and fusion for the treatment of 1-level symptomatic cervical disc disease. Spine J. 2009;9:275-286.
36. Ogon M., Howanietz N., Tuschel A., et al. Implantation of the ProDisc intervertebral disk prosthesis for the lumbar spine. Oper Orthop Traumatol. 2007;1:209-230.
37. Zigler J., Delamarter R., Spivak J.M., et al. Results of the prospective, randomized, multicenter Food and Drug Administration investigational device exemption study of the ProDisc-L total disc replacement versus circumferential fusion for the treatment of 1-level degenerative disc disease. Spine (Phila Pa 1976). 2007;32:1155-1162.
38. Bryan V.E.Jr. : Cervical motion segment replacement. Eur Spine J. 2002;11:S92-S97.
39. Sasso R.C., Smucker J.D., Hacker R.J., Heller J.G. Artificial disc versus fusion: a prospective, randomized study with 2-year follow-up on 99 patients. Spine (Phila Pa 1976). 2007;32:2933-2940.
40. Sasso R.C., Smucker J.D., Hacker R.J., Heller J.G. Clinical outcomes of Bryan cervical disc arthroplasty: a prospective, randomized, controlled, multicenter trial with 24-month follow-up. J Spinal Disord Tech. 2007;20:481-491.
41. Mummaneni P.V., Burkus J.K., Haid R.W., et al. Clinical and radiographic analysis of cervical disc arthroplasty compared with allograft fusion: a randomized controlled clinical trial. J Neurosurg Spine. 2007;6:198-209.
42. Punt I.M., Visser V.M., van Rhijn L.W., et al. Complications and reoperations of the SB Charité lumbar disc prosthesis: experience in 75 patients. Eur Spine J. 2008;1:36-43.
Nuclear Replacement
Fluoroscopically Guided Diagnostic Injections
To further discuss the available options in this case without spondylolisthesis, an ongoing and very passionate clinical controversy needs to be re-addressed; namely, whether test methods beyond conclusive radiographic and MRI should be utilized in the diagnosis of mechanical low back pain, especially if they are as invasive as provocation discography. With the disc and/or facet joints as the usual suspects for the source of pain, not only two different sources, but also two different treatment pathways need to be considered. The predominant current clinical opinion excludes patients with significant facet pain based on morphologically altered arthritic joints from treatment with a TDR or NR arthroplasty. For a clinical workup in anticipation of a surgical treatment, fluoroscopically guided injections for the facets (medial branch blocks) and potentially a provocation discography seem helpful. Aside from the pain response (provocation part) during the discography procedure itself, it is possible with subsequent CT of the disc to rate the degree of internal anulus disruption. The degree of disruption is the underlying cause for discogenic pain according to the current concept1 and is a sign of mechanical instability. Hence, it should not exceed a certain value to guarantee a functional anulus, one with the ability for load sharing with a NR device and also able to prevent its dislocation (data on file, unpublished).
Available Nucleus Replacement Devices
The use of NR devices in patients with mechanical back pain in the earlier stages of degeneration and with only moderately disrupted anuli has been investigated in several recent studies,2 including results for an injectable device (DASCOR System, Disc Dynamics, Eden Prairie, MN)3 and a mechanical device (NUBAC System, Pioneer Surgical Technology, Marquette, MN).4 Both have demonstrated their effectiveness and safety profiles. As of the end of 2009, several NR designs were in preliminary clinical investigation in the United States; however, only the NUBAC System is currently in an active Investigational Device Exemption (IDE) Food and Drug Administration (FDA) pivotal trial. Other NR designs are still under development or have been discontinued for technical reasons and/or adverse events. In Europe, certain NR devices have gained CE Mark approval and are still available. The different nucleus designs can be classified according to their material, their biomechanical behavior, or their surgical insertion features (Fig. 224-8). Chapter 163 has a more comprehensive overview of all NR devices.
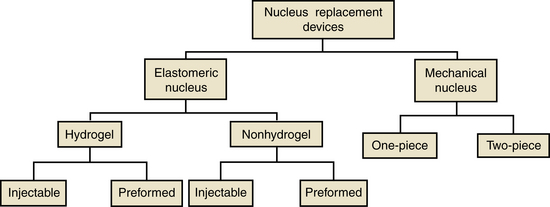
FIGURE 224-8 Classification of nuclear replacement devices.
(Data provided by Coric D, Mummaneni PV: Nucleus replacement technologies. J Neurosurg Spine 8:115–120, 2008.)
DASCOR Disc Arthroplasty System
The DASCOR device is a two-part in situ–cured polyurethane injected within an expandable polyurethane balloon to form the final NR device, which conforms to the nucleus cavity created (Fig. 224-9). The pressurized injection of the device into the disc space is controlled using a proprietary injection system.
Before the implantation, the system has the ability to verify intraoperatively the volume and size of the resultant DASCOR device using fluoroscopic imaging procedures in conjunction with a proprietary DASCOR imaging balloon. This imaging system also gathers important information about anulus integrity, enabling it to be used as a stand-alone system in conjunction with a discectomy procedure (Fig. 224-10). For the long-term functionality of a NR device, information about anulus integrity is vital. If the imaging step reveals an incompetent anulus, an alternative procedure for stabilization could be chosen. It is believed that many of the inherent design features of the DASCOR system contributed directly to its positive initial clinical results. For example, the combination of a pliable modulus of elasticity and a large cross-sectional area and surfaces that contour to the endplates supports the goal of creating a uniform load distribution on the endplates. Also, by filling the entire surgically created nucleus space under controlled pressure, load sharing between the nucleus and the anulus (prosthesis and endplates) is re-established. Migration is unlikely because only a small anulotomy is required for implantation of the catheter balloon; the device achieves its full size only after in situ injection. If ever required, revision can be easily performed.
NUBAC Disc Arthroplasty System
The NUBAC device is a two-piece mechanical nucleus. It has a ball-and-socket design with a large surface contact area to achieve load sharing, enable uniform stress distribution, and theoretically decrease the risk of subsidence and extrusion. The system is composed of PEEK-OPTIMA material, which offers well-established biocompatibility, biodurability, wear resistance, and radiolucency. The NUBAC is the first PEEK-on-PEEK articulated intradiscal arthroplasty device (Fig. 224-11).
Bao Q.B., Yuan H.A. New technologies in spine: nucleus replacement. Spine (Phila Pa 1976). 2002;27:1245-1247.
Bogduk N. Lumbar disc stimulation. In: Bogduk N., editor. Practice guidelines for spinal diagnostic and treatment procedures. San Francisco: International Spine Intervention Society, 2004.
Coric D., Mummaneni P.V. Nucleus replacement technologies. J Neurosurg Spine. 2008;8:115-120.
1. Bogduk N. Lumbar disc stimulation. In: Bogduk N., editor. Practice guidelines for spinal diagnostic and treatment procedures. San Francisco: International Spine Intervention Society, 2004.
2. Bao Q.B., Yuan H.A. New technologies in spine: nucleus replacement. Spine (Phila Pa 1976). 2002;27:1245-1247.
3. Ahrens M., Tsantrizos A., Donkersloot P., et al. Nucleus replacement with the DASCOR Disc Arthroplasty Device: interim 2-year efficacy and safety results from two prospective, non-randomized multicenter European studies. Spine (Phila Pa 1976). 2009;34:1376-1384.
4. Coric D., Mummaneni P.V. Nucleus replacement technologies. J Neurosurg Spine. 2008;8:115-120.