CHAPTER 91 Magnetic Resonance and Computed Tomographic Angiography of the Extracranial Carotid Arteries
There is clear evidence from multiple carotid trials, including a recent pooled data analysis, that surgical intervention with carotid endarterectomy (CEA) has significant benefits compared with medical therapy in symptomatic patients with severe carotid stenosis.1–3 Conventional catheter-based invasive angiography with cut film or intra-arterial digital subtraction angiography (DSA) has been the gold standard for measuring the carotid stenosis in these trials. The improved efficacy of noninvasive imaging techniques and the attendant risk of stroke during DSA has led many practices to adopt noninvasive modalities, such as Doppler ultrasound, magnetic resonance angiography (MRA), and/or computed tomography angiography (CTA) to replace this invasive study.
To understand how and when to apply these noninvasive modalities, we need to be able to balance our knowledge of the sensitivity and specificity of ultrasound, CTA, and MRA to identify a severe stenosis with the attendant risks of DSA as well as the risk of CEA. We can borrow a technique called decision analysis to help understand these various factors. Decision analysis takes the sensitivity and specificity of a noninvasive study, risk of stroke with DSA, risk of thromboembolic disease after CEA, risk of withholding CEA in patients with severe symptomatic stenosis, and risk of undergoing CEA with only moderate symptomatic carotid stenosis, as well as the costs of the noninvasive studies DSA, and CEA, into account.4 The results of this decision analysis allow us to test the risks or costs of various noninvasive carotid stenosis imaging strategies. Decision analysis demonstrates these various tradeoffs, which may not otherwise be apparent. Using DSA alone, all patients face the risk of procedural stroke. With MRA strategy, a few patients with false-positive results face an increased risk associated with CEA and a few patients with false-negative results face an increased risk because of the missed benefits of surgery. As Kuntz and colleagues4 have illustrated with decision analysis, it is better to have a false-positive result (and perform a CEA in patients with moderate stenosis, with its added slight morbidity per patient) than to have a false-negative result (and allow patients with severe stenosis not to undergo CEA, with a much higher morbidity per patient). Put another way, it is better to send a few extra patients for CEA who may not meet DSA requirements of severe stenosis than to miss patients with severe stenosis and withhold the important benefits of surgery.
Thus, choosing among the noninvasive tests, the option that yields the highest accuracy may not necessarily be better. One must consider the local sensitivity and specificity of a particular noninvasive imaging modality to detect a severe stenosis compared with DSA as well as the angiographic stroke risk and CEA stroke risk when deciding whether the locally acquired ultrasound, CTA, or MRA study can replace DSA in the preoperative evaluation of carotid stenosis (Fig. 91-1). Even when assuming a low angiographic stroke risk of 0.4%, as seen in the Veterans Affairs Cooperative Study, decision analysis has demonstrated that a noninvasive test with a sensitivity of 93% and specificity of 85% resulted in less morbidity than the DSA imaging strategy. This same analysis allows imagers and referring clinicians to compare the relative value of ultrasound, CTA, and MRA to replace DSA using site-specific data instead of relying on published results from outside facilities.
MAGNETIC RESONANCE ANGIOGRAPHY
Techniques
Indications
Despite the availability of high-quality ultrasound, CTA, and MRA, no consensus exists regarding the optimal noninvasive imaging strategy for preoperative evaluation of carotid stenosis. This is particularly true for individual MRA techniques as well. In the 1990s, time-of-flight (TOF) MRA was reported to have good sensitivity and specificity in detecting internal carotid artery stenosis greater than 70% using North American Symptomatic Carotid Endarterectomy Trial (NASCET) criteria identified on DSA.5–7 However, carotid MRA became more clinically viable with the introduction of CE MRA, which offered the opportunity to cover more of the carotid artery distribution in a fraction of the scan time requirements of TOF MRA. With the advent of elliptical-centric phase reordering and effective timing of the gadolinium contrast bolus, first-pass CE MRA moved from research into routine clinical practice.
Some authors still insist that despite the ability to perform reliable CE MRA, TOF MRA remains the most accurate technique.8 For those who prefer CE MRA, three competing techniques have evolved. One approach uses multiple time points during the gadolinium bolus arrival with multiple, rapid, three-dimensional acquisitions with relatively low spatial resolution. To achieve a higher spatial resolution and improved temporal resolution, three-dimensional acquisitions reconstructed with a novel oversampling of the center of k-space are possible. This technique is termed time-resolved imaging of contrast kinetics (TRICKS).
Contrast-Enhanced Magnetic Resonance Angiography
As experience with gadolinium bolus arrival timing improved, many authors abandoned the time-resolved approach to carotid CE MRA in favor of a higher spatial resolution three-dimensional MRA technique at a single time point (Fig. 91-2). Carotid CE MRA benefits from a large residual carotid retention of the initial bolus of contrast injection and relative lack of motion concerns, which enables the acquisition of longer duration, high spatial resolution three-dimensional MRA. A difficultly with carotid CE MRA, however, has been the relatively brief arteriovenous enhancement window. Jugular venous enhancement can be seen as quickly as 5 seconds following carotid artery enhancement secondary to the lack of Gd-chelate contrast agent penetration of an intact blood-brain barrier and the rapid return of gadolinium contrast through the brain parenchyma to the jugular veins. By modeling the elliptical-centric k-space phase-ordering scheme, which provides a very efficient and compact central k-space sampling, Fain and associates9 have demonstrated that there is sufficient image contrast for preferential arterial high spatial resolution with the longer acquisition times necessary for high spatial resolution carotid CE MRA. The use of elliptical-centric three-dimensional MRA, in combination with an accurate bolus arrival scan or fluoroscopic triggering, allows for high spatial resolution carotid CE MRA with a voxel size of 1 mm3 or smaller, with reliable and high intra-arterial contrast and very little venous contamination.10 Use of a neurovascular coil ensures proper coverage of the carotid arteries from the aortic arch through the circle of Willis.
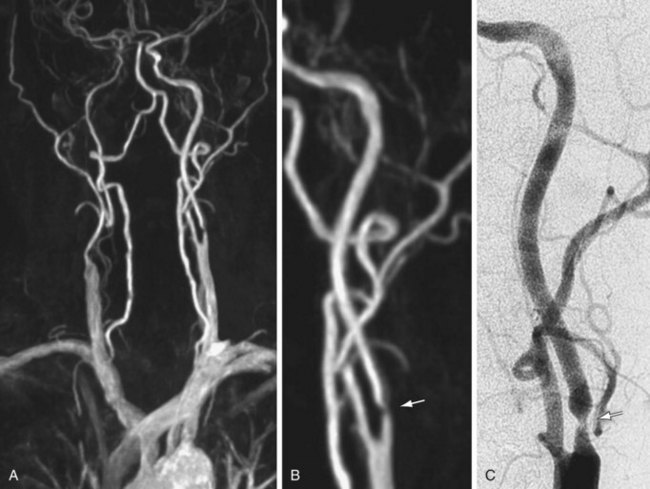
(Courtesy of Dr. Winfried. A. Willinek, Department of Radiology, University of Bonn, Bonn, Germany.)
Spatial Resolution
Hinatiuk and coworkers11 have reviewed the effect of increased spatial resolution in depicting carotid stenosis as seen on CE MRA at 1.5T. In their patients with carotid artery stenosis, decreasing the voxel volume from 0.9 to 0.53 mm3 by increasing the scan matrix while keeping the FOV constant caused the scan time to increase from 21 to 40 seconds. The resulting CE MRA with improved resolution from the 0.53 mm3 resulted in the sharpest depiction of the carotid stenosis. With modern gradient systems, TRs for elliptical-centric acquisitions are roughly half what they were during the early work on carotid CE MRA. The authors made use of the 50% reduction in TR to almost double the spatial resolution of elliptical-centric carotid CE MRA compared with the initial 0.8 to 1.0 mm3 voxel size while maintaining an imaging time of 40 seconds. This reduction in voxel volume to 0.53 mm3 resulted in a much better depiction of the carotid stenosis. A total of 30 mL of Gd-chelate contrast medium was used to achieve adequate signal-to-noise ratio (SNR). Further decreases in voxel volume, by extending the acquisition time to 50 to 60 seconds, did not improve the vessel depiction because of both a drop in SNR and sharpness losses, possibly from motion. Nael and colleagues12 have extended the resolution of elliptical-centric carotid CE MRA to 0.44 mm3 by making use of the extra SNR at 3.0T.
Parallel Imaging
Parallel imaging is another technique to shorten the acquisition time of first-pass elliptical-centric carotid CE MRA and/or support higher spatial resolution. In general, the efficacious use of parallel imaging allows one to maintain the recommended 1.0 mm or less in plane spatial resolution with a voxel volume of 0.8 mm3 or less while maintaining adequate SNR to achieve a good-image quality. Most authors have recommended using a twofold (i.e., 2×) acceleration factor at 1.5 T. The imaging volume is then increased by 50% in the z direction of the coronal CE MRA acquisition (anteroposterior direction). This simplifies the prescription of the imaging volume. The resulting CE MRA sequence is 25% faster compared with a smaller nonaccelerated prescription. By traversing the center of k-space faster with parallel imaging, the SNR benefits from the gadolinium bolus can be maximized. This helps offset the loss of SNR from the parallel imaging technique. Fourfold acceleration of 3.0-T carotid CE MRA using parallel imaging in phase- and detector row–encoding directions is also possible. Almost isotropic 0.7- × 0.7- × 0.9-mm resolution (0.44 mm3 voxel volume) CE MRA from the aortic arch through the circle of Willis have been reported with this 3.0-T MR technique.12 This study demonstrated some of the highest spatial resolution carotid CE MRA examinations ever achieved with a large FOV (Fig. 91-3).
Dedicated Carotid Coils
A dedicated neurovascular phased array coil is used in most cases because it not only provides an optimized SNR for carotid imaging and the ability to implement parallel imaging techniques, but also extends carotid artery coverage from its origin at the aortic arch through the circle of Willis. More recently, dedicated carotid surface coils that cover 14 to 18 cm of the midneck have become commercially available at 3.0 T. These dedicated surface coils provide another factor of improvement in SNR compared with the larger FOV clamshell design neurovascular coils that extend from the aortic arch through the circle of Willis. These dedicated carotid surface coils support even higher spatial resolution carotid CE MRA with a voxel size of 0.59 × 0.59 × 0.80 mm without zero filling. This corresponds to a voxel volume of 0.27 mm3 with dedicated carotid surface coils at 3.0 T compared with 0.44 mm3 as used by Nael and associates12 at 3.0 T using a large FOV clamshell neurovascular coil and 0.53 to 0.66 mm3 as used by Hnatiuk and coworkers11 and Willinek and colleagues13 at 1.5 T with a large FOV clamshell neurovascular coil. Carotid CE MRA at 3.0 T using dedicated surface coils results in the highest resolution study achieved to date with CE MRA, and competes favorably with the spatial resolution of the 64-detector CTA.
Field of View
Raw resolution alone may not fully explain the ability to dedicate the carotid lumen stenosis on the CE MRA. According to the study by Fain and associates,9 one key factor in improving the performance of elliptical-centric phase-reordered CE MRA as measured by the point spread function (PSF) is to minimize the phase FOV in both the y– and z-axes. By focusing on just the middle 14 to 18 cm of the neck, we can limit the phase FOV in both directions while generating substantially higher spatial resolution CE MRA compared with using a larger FOV and an eight-channel neurovascular coil. At 3.0 T, there is a sufficient carotid SNR to realize improved spatial resolution benefits using a small FOV and dedicated carotid surface coils. The improved PSF provided by the smaller phase FOV should result in a sharper depiction of the arterial lumen boundary compared with a similarly prescribed spatial resolution using a larger FOV. Stated another way, the same spatial resolution prescribed using a large FOV would result in inferior CE MRA compared with a small FOV because of the effect of the smaller phase FOV on the PSF. The arterial lumen boundaries would not be as sharp, despite the same prescribed resolution. This discussion assumes that we somehow generate enough SNR using the larger neurovascular coil, too. In addition to small FOV in the xy direction, we can acquire less coverage in the z direction by covering only the middle portion of the neck. Larger phase FOV in the z direction is required to cover the entire course of the carotid artery from the arch through the circle of Willis. If we desire the highest resolution carotid CE MRA, Fain9 would predict that a small phase FOV of approximately 18 cm in both the y and z direction would be optimal.
Initial results of dedicated carotid coil limited FOV carotid CE MRA have confirmed excellent correlation with DSA (Fig. 91-4).14 In patients for whom coverage of the carotid arteries that is limited to a 15 to 18 cm FOV in the neck is sufficient, dedicated carotid coil 3.0-T CE MRA represents the highest resolution noninvasive study possible today. This method is particularly well suited for clinical practices in which the decision to proceed to CEA is based on ultrasound without the need of the noninvasive testing to visualize the remainder of the carotid artery directly. If there is a carotid CTA that is limited because of extensive calcifications, the dedicated carotid coil 3.0-T CE MRA can depict the carotid stenosis without artifacts from the calcifications with similar or higher spatial resolution than CTA (Fig. 91-5). The use of such high SNR dedicated carotid surface coils at 3.0 T also makes carotid plaque imaging possible. Although this is still experimental, there is great potential to depict not only carotid stenosis but the underlying plaque causing the narrowing (Fig. 91-6). There is a strong possibility that carotid plaque characteristics as depicted by MRI may be a predictor of which patients with moderate asymptomatic carotid stenosis are at risk to proceed on to stroke or a transient ischemic attack (TIA).15