Chapter 97B Liver transplantation
Anesthesia, perioperative management, and postoperative care
Overview
Approximately 20,000 liver transplantations are performed annually worldwide (Matesanz et al, 2009), but the number of patients on the waiting list continues to exceed the donor organ availability. Because of the continuous donor shortage, dramatic changes in recipient and donor selection have emerged in the past decade, leading to an amplification of clinical challenges for perioperative liver transplant physicians. In fact, United Network for Organ Sharing (UNOS) data from 2009 (UNOS/OTPN, 2010) show that older patients are now accepted to the waiting list and transplanted; combined kidney and liver transplants are performed more often than in prior years; and extended-criteria donors, including donors after cardiac death, are increasingly utilized (see Chapter 97A).
Despite the increasing medical complexity, overall graft and patient outcomes have continued to improve. According to the UNOS database, survival rates for patients transplanted with a cadaveric graft are around 87% at 1 year, 80% at 3 years, and 74% at 5 years. These encouraging figures, achieved by experienced transplant teams, have resulted in part from the progressive advancement and standardization of surgical and anesthetic techniques (Mandell et al, 2008). If the current trends continue, with transplantation of increasingly older and sicker recipients (Costa et al, 2009; Xia et al, 2008) and use of marginal donor organs, the outcomes will greatly depend on accurate preoperative assessment and on appropriate and timely intraoperative medical management of comorbidities.
Preoperative Anesthetic Assessment
Liver transplantation is the current gold standard treatment for acute and chronic irreversible liver failure (see Chapters 70A, 72, and 97A); for selected clinical syndromes not manifesting with end-stage liver disease (ESLD), including polycystic liver disease (see Chapter 69B) and other metabolic diseases; for some malignancies confined to the liver (see Chapters 97D and 97E); and for cholestatic liver diseases in children, such as biliary atresia (see Chapters 40 and 71). Four contraindications to liver transplantation are widely accepted: 1) unstable cardiopulmonary conditions, such as symptomatic coronary artery disease (CAD), severe systolic dysfunction, advanced cardiomyopathy, severe valvular heart disease, and severe pulmonary hypertension; 2) substance abuse; 3) morbid obesity (body mass index [BMI] >40); and 4) uncontrolled sepsis (Gallegos-Orozco & Vargas, 2009; Murray & Carithers, 2005).
As part of a multidisciplinary transplant team, anesthesiologists should participate in the evaluation of candidate recipients. Ideally, an anesthetic evaluation should precede the acceptance of patients to the waiting list. The goals of this assessment are to appraise suitable candidates based on functional status and comorbidities and to design a patient-specific preoperative and perioperative treatment plan. For patients in whom the burden of comorbidities presents a prohibitive operative risk, alternative treatments should be sought (Merion, 2004).
Cardiovascular System
Hyperdynamic circulation, cardiomyopathy, and CAD are common clinical problems that impact postoperative outcomes in orthotopic liver transplantation (OLT) candidates (Fouad et al, 2009).
Hyperdynamic Circulation
Patients with ESLD develop high cardiac output hemodynamics secondary to reduced systemic vascular resistance (SVR) and abnormal distribution of central, splanchnic, and peripheral circulation. This condition is due to humoral and autonomic dysregulation (Møller & Henriksen, 2008). When this situation is associated with cardiac valve dysfunction, especially mitral or tricuspid valve insufficiency, more hemodynamic support is needed in the perioperative period (Alper et al, 2009).
Coronary Artery Disease
Perioperative complications and mortality rates are high in patients with CAD: up to 50% of patients with significant CAD may die perioperatively from cardiac complications (Plotkin et al, 1996). Safadi and colleagues (2009) recently reported that history of stroke, CAD, postoperative sepsis, and increased interventricular septal thickness were markers of adverse perioperative cardiac outcomes, whereas use of perioperative β-blockers was significantly protective. Occult CAD is also common: 5% to 26% of OLT candidates have at least one critical coronary stenosis. Computed tomography (CT) scan detection of coronary calcification is a sign of possible ischemic heart disease in asymptomatic patients, but the sensitivity and specificity are low (Mandell & Tsou, 2008; Tiukinhoy-Laing et al, 2006).
Dobutamine stress echocardiography and dobutamine myocardial perfusion imaging are considered the most sensitive noninvasive tests to assess occult CAD in liver transplant candidates. Both tests have a higher negative than positive predictive value (Donovan et al, 1996; Mandell & Tsou, 2008; Murray & Carithers, 2005; Plotkin et al, 1998; Williams et al, 2000). Cardiac catheterization is indicated in patients with a positive noninvasive test to assess coronary lesions and plan further treatment. Left-heart catheterization is associated with higher risk of minor complications but can be performed safely in candidates for OLT (Sharma et al, 2009).
Cirrhotic Cardiomyopathy
Cirrhotic cardiomyopathy is characterized by systolic dysfunction more evident under conditions of hemodynamic stress, impaired diastolic relaxation, and electrophysiologic alterations often manifesting with a prolonged QT interval. Diagnosis is based on electrocardiograph (ECG), cardiac echo, and elevated levels of brain natriuretic factor. Physiopathologic hallmarks of this disease are altered β-adrenergic activity, myocardial fibrosis, myocyte hypertrophy, and ion-channel defects. Medical treatment is based on sodium restriction and administration of β-blockers and aldosterone antagonists; however, liver transplantation can reverse this condition (Fukazawa et al, 2009; Mandell & Tsou, 2008; Wong, 2009).
Nonischemic Cardiomyopathy
Nonischemic cardiomyopathy can complicate alcoholic cirrhosis, amyloidosis, hemochromatosis, and Wilson disease, and it must be considered in the anesthetic preoperative assessment and intraoperative management. Dilated and hypertrophic cardiomyopathy can also be present in OLT candidates, and these rarely revert to normal even after OLT (Mandell & Tsou, 2008).
Pulmonary System
As many as 70% of patients with chronic liver disease (CLD) suffer from respiratory problems, that manifest as impaired respiratory mechanics and gas exchange (Kim et al, 2009). Most pulmonary diseases are independent from the liver disease but are in some instances related to liver disease (e.g., α1-antitrypsin deficiency and cystic fibrosis) (Yeshua et al, 2009). Pulmonary evaluation includes history and physical examination, radiologic studies, arterial blood gas, and pulmonary function tests. Right-heart catheterization is indicated when clinical or ECG evidence suggests pulmonary hypertension.
Chronic Obstructive Pulmonary Disease and Smoking
Chronic obstructive pulmonary disease (COPD) is common and is often undiagnosed in candidates for liver transplantation. In a recent prospective study involving several academic centers in the United States, 18% of OLT candidates had COPD, and 80% of those patients had not been previously diagnosed. Older age and smoking were significant risk factors for this condition, although the impact of COPD on perioperative outcome in liver transplantation has not been well defined (Ryback et al, 2008).
Hepatic Hydrothorax
Hepatic hydrothorax is defined as a pleural effusion greater than 500 mL not caused by cardiac or pulmonary diseases in a patient with cirrhosis (Singh & Sager, 2009). A diaphragmatic defect allowing passage of ascites from the peritoneal to the pleural cavity is considered the main mechanism leading to this complication. Pleural effusions are symptomatic in 6% to 10% of patients with ESLD (Kiafar & Gilani, 2008), and the main respiratory impairment is hypoxemia secondary to atelectasis and shunting; chest radiograph confirms the diagnosis. Treatment is based on medical management of ascites with paracentesis and possible placement of a transjugular intrahepatic portosystemic shunt (TIPS). In cases of refractory hydrothorax, pleurodesis and diaphragmatic repair can be considered; thoracentesis is performed only in emergency situations, because avoidance of tube thoracostomy is preferred.
Portopulmonary Hypertension
Portopulmonary hypertension (PPHTN) is a specific type of pulmonary artery hypertension. It comprises increased pulmonary vascular resistance (PVR) and portal hypertension with or without advanced liver disease. From 2% to 10% of patients with cirrhosis are affected by PPHTN (Yeshua et al, 2009). The physiologic mechanism is multifactorial and incompletely understood, but hyperdynamic circulation, imbalance between pulmonary vasodilators (nitric oxide and prostacyclin) and vasoconstrictors (endothelin-1 and thromboxane), and proliferative pulmonary arteriopathy all play a role in the genesis of this syndrome (Singh & Sager, 2009).
Diagnosis requires presence of portal hypertension, mean pulmonary arterial pressure (mPAP) greater than 25 mm Hg, PVR greater than 240 dynes/sec/cm−5, and pulmonary artery occlusion pressure of less than 15 mm Hg (Rodriguez-Roisin et al, 2004); right-heart catheterization is indicated if pulmonary artery systolic pressure is higher than 50 mm Hg on ECG screening. The severity of PPHTN is then evaluated based on hemodynamic data obtained by right-heart catheterization at rest and is classified as mild (mPAP of 24 to 34 mm Hg), moderate (mPAP of 35 to 44 mm Hg), or severe (mPAP ≥45 mm Hg) (Singh & Sager, 2009).
Medical optimization is indicated before transplantation, and treatment is based on vasodilators. Prostaglandins (intravenous epoprostenol or inhaled iloprost), phospodiesterase inhibitors (sildenafil), and endothelin-1 antagonists (bosentan) are often used in combination therapy. Most liver transplant centers do not transplant patients with an mPAP greater than 50 mm Hg or a PVR greater than 240 dyne/sec/cm−5; however, 5-year survival is 14% without treatment, 45% with medical management, and 65% for those who had an OLT following pretreatment with prostacyclin (Swanson et al, 2008).
Hepatopulmonary Syndrome
Hepatopulmonary syndrome (HPS) is a vascular disorder characterized by hypoxemia secondary to pulmonary capillary vasodilation in patients with liver disease. The estimated incidence in patients with cirrhosis is between 5% and 32% (Singh & Sager, 2009). The natural course of HPS is characterized by poor survival, especially in patients with a Pao2 level of less than 50 mm Hg: median survival without liver transplantation is approximately 24 months (Schenk et al, 2002; Umeda & Kamath, 2009).
The pathologic feature of HPS is gross dilation of the pulmonary precapillary and capillary vessels (Rodriguez-Roisin & Krowka, 2008) leading to a ventilation-perfusion mismatch. The clinical symptoms are dyspnea with or without orthodeoxia (arterial oxygen desaturation while standing). The diagnosis is confirmed with arterial blood gas measurement and contrast-enhanced ECG or macroaggregated albumin lung perfusion scan. The ECG diagnostic sign is the visualization of saline bubbles in the left heart, three to six beats after the appearance of bubbles in the right heart, following the injection of agitated saline in a peripheral vein.
No effective medical therapy for HPS has been found, and liver transplantation is the only treatment. UNOS criteria consider a Pao2 level below 60 mm Hg an indication of high priority for OLT, even if the allocation of “exception points” to patients with HPS has now become controversial (Sulieman et al, 2008). About 85% of patients who underwent OLT for HPS showed resolution or improvement of hypoxemia. Still, the mortality after liver transplantation is significantly increased in patients with HPS (Fallon et al, 2008).
Hemostasis in End-Stage Liver Disease
The liver synthesizes most of the factors involved in clot formation and, equally importantly, factors opposing clot formation or mediating its lysis. The traditional concept that patients with advanced liver disease are hypocoagulable has been challenged. The current view emphasizes complex disturbances of platelet function, procoagulant, anticoagulant, and fibrinolytic pathways that result in rebalancing of the hemostatic system (Senzolo et al, 2006). The net effect may be a hypocoagulable or hypercoagulable state with a tendency for bleeding or thromboembolism (Warnaar et al, 2008b). Understanding the hemostatic system in ESLD is essential to perioperative coagulation management and optimization of blood product transfusion practices, which are directly related to morbidity and mortality in liver transplantation (de Boer et al, 2008; Massicotte et al, 2005).
Deficiency in primary hemostasis is due to quantitative and qualitative platelet changes (Pereboom et al, 2008). Mild to moderate thrombocytopenia is common. Causes are splenomegalic platelet sequestration in portal hypertension, impaired megakaryocytopoiesis as a result of reduced production of thrombopoietin by the cirrhotic liver, folic acid deficiency in alcoholic cirrhosis or acute hepatitis C infection, and reduced platelet half-life related to autoantibodies (Lisman & Leebeek, 2007; Senzolo et al, 2006). Evidence for impaired platelet aggregation and adhesion in cirrhosis is controversial. The proposed mechanisms are complex and multifactorial, including the important role of two potent endothelium-derived platelet inhibitors, nitric oxide and prostacyclin. Contrary to this, more recent work suggests that the functional capacity of platelets in cirrhotic patients may be normal when platelet number and hematocrit are normalized (Lisman & Leebeek, 2007; Tripodi et al, 2007), suggesting that the main defect in primary hemostasis is thrombocytopenia. An important compensatory mechanism for the overall deficient platelet function in cirrhosis is an increase in endothelium-synthesized von Willebrand factor (vWf). The markedly upregulated vWf promotes adhesion of platelets to injured vasculature and seems to be more effective than plasma from healthy volunteers (Lisman & Leebeek, 2007).
All proteins involved in fibrinolysis are synthesized by the liver except tissue plasminogen activator (tPA) and plasminogen activator inhibitor type 1 (PAI-1). Consequently, reduced levels of liver-synthesized factors—plasminogen, plasmin inhibitor, thrombin activatable fibrinolysis inhibitor (TAFI), and factor XIII—are found in both acute and chronic liver failure. Cirrhosis is classically associated with hyperfibrinolysis, whereas patients in ALF may have hypofibrinolysis as a result of increased production of PAI-1. The recent expert viewpoint is contrary to the traditionally held view and emphasizes that the existing in vitro evidence of hyperfibrinolysis in cirrhosis is weak (Lisman & Leebeek, 2007). Increased levels of fibrinogen degradation indicators—d-dimers, plasmin-antiplasmin and thrombin-antithrombin complexes, thrombin fragment F1+2—could be explained by their delayed clearance by the diseased liver.
Coagulation tests, such as the prothrombin time (PT) and activated partial thromboplastin time (aPTT), are used to assess the severity of synthetic dysfunction, and PT-INR is a part of the Child-Turcotte-Pugh (CTP) and Model for End-Stage Liver Disease (MELD) prognostic indexes (Ng, 2009). As tests of in vivo coagulation function, PT-INR and PTT are conceptually deficient because they measure only the procoagulant pathway and ignore the anticoagulant function. This inadequacy is best illustrated by the observation that when the (prolonged) PT is modified by including thrombomodulin to allow full activation of protein C, the thrombin generation in cirrhotic patients is close to normal (Tripodi et al, 2005). This may in part explain why PT-INR and PTT are poor predictors of bleeding in cirrhosis and in liver transplantation (Massicotte et al, 2008). Thromboelastogram (TEG; Haemoscope Corp., Niles, IL) and rotation thromboelastometry (ROTEM; Pentapharm, Munich, Germany) have the advantage of assaying whole-blood clot formation and lysis, including the platelet contribution, but these assays do not incorporate the endothelial component (see Intraoperative Monitoring, below). Importantly, these assays allow easy recognition of hypercoagulable states (Fig. 97B.1A).
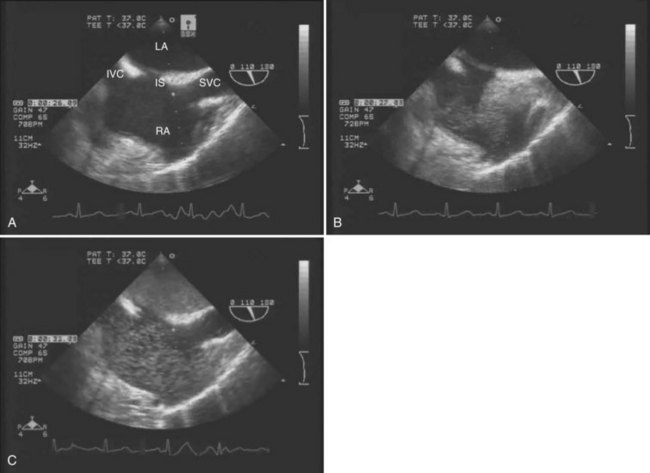
FIGURE 97B.1 Intraoperative transesophageal echocardiography (TEE) in a patient with hepatopulmonary syndrome and intrapulmonary shunting demonstrates a positive bubble study. A, Midesophageal bicaval view. B, Bolus of contrast injectate (agitated saline-blood mixture) is visible entering the right atrium via the superior vena cava (SVC) following an injection through the central venous port. C, Several heartbeats later, the injectate is present in the left atrium (LA), indicating transpulmonary passage. RA, right atrium; IS, interatrial septum; IVC, inferior vena cava.
Renal System
Renal failure confers increased risk of death to cirrhotic patients, both while on the transplant waiting list and after liver transplantation. Implementation of the MELD score in 2002 (Moreau et al, 2003) has prioritized patients with renal failure waiting for a liver transplant, resulting in reduced overall mortality on the transplant list (Ojo, 2009).
With progression of cirrhosis, sympathetic and rennin-angiotensin vasoconstrictor systems are activated that temporarily maintain arterial blood pressure, but they also cause renal vasoconstriction and hypoperfusion. Activation of nonosmotic hypersecretion of arginine vasopressin, aimed at preserving circulatory volume, results in retention of solute-free water with attendant hyponatremia, edema, and ascites. HRS is almost invariably associated with ascites and rarely develops in its absence (see Chapter 74). HRS is classified into one of two types: HRS 1 is characterized by a rapid decline in renal function reflected in doubling of serum creatinine (SCr) to above 2.5 mg/dL over a 2-week period; this type of HRS has a particularly ominous clinical course, leading to multiorgan dysfunction that affects the heart, brain, and other organs. HRS 2 has a more benign, slowly progressive course. It is manifested by a milder elevation of SCr (1.5 to 2.5 mg/dL) and refractory ascites. Onset of HRS is often triggered by a discrete event, such as spontaneous bacterial peritonitis, use of nonsteroidal antiinflammatory drugs (NSAIDs), or hypovolemia as a result of diuretic use, gastrointestinal (GI) bleeding, or fluid losses.
Measurement of SCr remains the primary test of renal function despite its well-known limitations. By consensus, SCr above 1.5 mg/dL (133 µmol/L) defines renal insufficiency in cirrhosis (Gines & Schrier, 2008; Salerno et al, 2007). GFR and creatinine clearance are more accurate but less practical tests when repeated assessments are needed. Other tests measure serum and urinary electrolytes, fractional sodium excretion (FENa), albumin, osmolarity, sediment, and biologic markers. Specific diagnostic tests for HRS do not exist, rather diagnosis is based on the presence of liver disease, a precipitating factor, and FENa less than 1%, signifying preserved tubular reabsorption; however, the latter test is invalidated by the use of diuretics.
The main pharmacologic approach utilizes vasoconstrictors, including a selective vasopressin V1 receptor agonist, such as terlipressin, or α-adrenergic agonists, such as norepinephrine and midodrine. Vasoconstrictors in conjunction with albumin are only modestly beneficial but are the best medical therapy currently available. Two recent studies showed a marked improvement in renal function in 40% of patients treated with terlipressin and albumin compared with controls treated with albumin alone (Martin-Liahi et al, 2008; Sanyal et al, 2008). Although the overall survival in the treatment group was not better than in the control group, the probability of 3-month survival in patients who responded with improved renal function was significantly higher (58% vs. 15%) than in nonresponders (Martin-Liahi et al, 2008); however, organ ischemia is a concerning side effect of vasoconstrictor administration.
Placement of a TIPS improves renal function and GFR and reduces sympathetic and renin-angiotensin-aldosterone axis activation in about 60% of patients (Cardenas & Gines, 2009; see Chapter 76E). TIPS is less applicable in HRS 1 as a result of severe liver disease, but it may delay progression of HRS 2 to HRS 1. No advantage in survival has been demonstrated in patients with refractory ascites treated by TIPS compared with repeated paracentesis and intravascular volume replacement with albumin.
Renal replacement therapy (RRT) offers a bridge to transplant, but the optimal RRT method in HRS and the benefit to patient outcomes are not known (Gines & Schrier, 2009). Continuous venovenous hemodialysis (CVVHD) seems to be hemodynamically the most favorable form of RRT (Davenport, 2009). CVVHD can be used to effectively control intravascular volume, pH levels, and solutes (Na, K, SCr, urea, ammonia); in addition, it corrects sodium in a time-controlled fashion, which is particularly relevant to minimize the risk of pontine demyelination in patients with hyponatremia.
Liver transplantation is the treatment of choice for patients with cirrhosis and HRS (Cardenas & Gines, 2009; see Chapter 97A). About 90% of patients with HRS recover kidney function after successful liver transplantation. The remaining 10% do not recover their kidney function and require prolonged RRT and eventual kidney transplantation. When the cause of renal failure in cirrhosis is HRS, the best predictor of need for a combined liver-kidney transplant is pretransplantation RRT for longer than 8 weeks.
Central Nervous System
Hepatic encephalopathy (HE) is a progressive but potentially reversible metabolic disorder of the central nervous system (CNS), and it is associated with variable degrees of brain edema. HE is an overt manifestation of cirrhosis in up to 45% of patients, and it is a defining sign and main cause of death in ALF (Eroglu et al, 2009). The World Congress of Gastroenterology recognizes three classifications of HE based on the etiology (Ferenci et al, 2002): type A is associated with ALF, type B with TIPS, and type C with cirrhosis. Based on the severity of neurologic impairment, HE ranges from normal (grade 0) to mildly impaired cognitive and neuromuscular function (grade 1), progressive lethargy (grade 2), confusion with muscle rigidity or clonus (grade 3), to coma and brain death (grade 4). Although presence and severity of HE has prognostic and therapeutic significance in cirrhosis and ALF, it is not a part of the MELD score.
The pathophysiology of HE is complex and incompletely understood. Multiple putative mediators have been implicated that include ammonia, endogenous benzodiazepine-like or γ-aminobutyric acidlike agonists, reactive oxygen species, inflammatory cytokines, hyponatremia, and manganese. The exact pathogenesis of cerebral edema remains controversial, but good evidence supports the role of ammonia, which promotes astrocyte and neuron swelling by increasing intracellular glutamine content. Transported to the mitochondria, glutamine contributes to production of reactive oxygen species and activation of mitochondrial transition permeability pore and various kinases, resulting in cellular swelling. Increased ammonia levels have been shown to alter cerebral blood flow and brain glucose utilization (Bjerring et al, 2009; Sundaram et al, 2009). High arterial and brain levels of ammonia in ALF correlate well with cerebral edema and increased ICP, with consequent high risk of mortality from brain herniation and hypoxia (Wendon et al, 2008).
HE in cirrhosis features slow onset, a progressive course, and only partial responsiveness to therapy. Significant cerebral edema typically does not develop, and a precipitating event—such as infection, excessive dietary protein, dehydration, or GI bleeding—can be often identified. TIPS is a known risk factor and is associated with 30% of new or worsening encephalopathy (see Chapter 76E). In addition to correction of any precipitating factors, treatment includes nonabsorbable disaccharides, which reduce the intestinal production and absorption of ammonia; antibiotics that target urease-producing bacteria; ornithine and acarbose; benzodiazepine receptor antagonists; and probiotics.
The hallmarks of HE in ALF are cerebral edema and increased intracranial pressure (ICP). Both are life-threatening complications of grade 3 and 4 encephalopathy. Treatment includes a number of general and specific interventions (Table 97B.1) aimed at promoting cerebral perfusion pressure (CPP) above 60 mm Hg; this is achieved by maintaining mean arterial pressure (MAP) and reducing ICP to below 20 to 25 mm Hg by reducing brain edema. Grade 3 or 4 HE requires endotracheal intubation for airway protection. Mild hyperventilation temporarily relieves brain hyperemia, but prolonged hyperventilation is not beneficial and may cause CNS ischemia. Mannitol is effective in reducing ICP in the short term, but its prophylactic use is not recommended. Recent evidence demonstrates that N-acetylcysteine treatment may also prolong transplant-free survival in ALF unrelated to paracetamol/acetaminophen toxicity (Lee et al, 2007).
Table 97B.1 Management of Encephalopathy and Elevated Intracranial Pressure in Acute Liver Failure
General Measures | Specific Interventions |
---|---|
Head elevation to 30 degrees | Endotracheal intubation for grades 3 and 4 hepatic encephalopathy |
Judicious (restrictive) fluid management | Hyperventilation: limited, temporary benefit |
Judicious if any sedation | N-acetylcysteine: benefits all causes of acute liver failure |
Cerebral perfusion pressure >60 mm Hg | Renal replacement therapy: CVVHD |
Osmotic diuresis, hypertonic saline | ICP monitoring (>10% bleed); transcranial Doppler |
Empiric: antibiotics, disaccharides | Hypothermia: mild, bridge to transplantation |
CVVHD, continuous venovenous hemodialysis; ICP, intracranial pressure
The role of ICP monitoring is controversial, as ICP monitor placement is associated with high risk of bleeding in this patient population, and no data exist to support its role in improving patient outcomes. The Acute Liver Failure Study Group recommends this intervention only for liver transplant candidates but recognizes regional practice differences (Stravitz et al, 2007). An attractive, noninvasive alternative to ICP monitors is transcranial Doppler (TCD) sonography. By measuring blood flow velocities in middle and anterior cerebral arteries and calculating resistance and pulsatility indexes, TCD allows serial estimates of cerebral blood flow in the setting of impaired autoregulation and developing brain edema (Bhatia & Gupta, 2007). In a small series of ALF patients, confirmation of adequate cerebral blood flow by TCD was successfully used as a basis to proceed with OLT (Bindi et al, 2008). Intraoperative use of TCD in OLT has not been reported.
Mild to moderate hypothermia (32° C to 35° C) is an emerging therapeutic modality. Hypothermia reduces brain ammonia and cytokine levels and in part restores cerebrovascular autoregulation and brain glucose metabolism. Although experimental evidence provides a strong rationale, only limited clinical data on efficacy and safety of hypothermia in humans in ALF are available, and future studies are warranted (Stravitz et al, 2009).
Intraoperative Monitoring
Hemodynamic Management
Hemodynamic management is a cornerstone in the perioperative care of liver transplant patients, but no consensus has been reached on the standards of hemodynamic monitoring (Claus-Georg & De Wolf, 2008). Continuous invasive arterial pressure monitoring may be the only ubiquitously used hemodynamic monitor in liver transplantation. When measured at the most common site, the radial artery, peripheral arterial pressure may underestimate the true aortic pressure, particularly during periods of hypotension. A femoral arterial line reflects central aortic pressure with higher fidelity and consistently records higher systolic pressures than the radial artery. The difference between the femoral and the arterial pressure waveform is particularly pronounced after graft reperfusion or when high doses of vasopressors are used (De Wolf, 2006; Shin et al, 2007). Because of this discrepancy, and because of the need for frequent arterial blood sampling, some centers routinely use both femoral and radial arterial lines.
The pulmonary artery catheter (PAC, see Chapter 22) has long been the mainstay of hemodynamic monitoring in liver transplantation (De Wolf et al, 1993); however, the clinical utility of PACs has recently been questioned because of criticisms that its invasiveness is not justified by improved outcomes (Sandham et al, 2003; Wheeler et al, 2006) and that measured pressures are not good estimates of ventricular preload (Kumar et al, 2004). Although a number of less invasive technologies have emerged, resulting in a decrease in their use, PACs are still advocated by many for OLT (Della Rocca et al, 2008; De Wolf, 2008; Pinsky, 2006), and the ability of PACs to continuously display pulmonary artery pressures is particularly advantageous when managing patients with portopulmonary hypertension. Transesophageal echocardiography (TEE) provides different but complementary information to that provided by PACs (see below), and concurrent use of both devices is considered superior (De Wolf & Aggarwal, 2008). A notable shortcoming of PACs in OLT is the inaccuracy of thermodilution cardiac output in the period following liver reperfusion because of postreperfusion hypothermia and subsequent instability of central temperature.
Newer, less invasive hemodynamic monitors for OLT have been introduced. The PiCCO system (Pulsion Medical Systems, Munich, Germany) combines transpulmonary thermodilution and arterial pressure contour analysis, and it provides continuous cardiac output in alignment with PAC thermodilution and a number of indices of preload that include global end-diastolic volume (GEDV) and intrathoracic blood volume (ITBV), which agree better with cardiac index than filling pressures (Della Rocca et al, 2002a, 2002b). PiCCO requires central venous and femoral or brachial arterial access, which arguably is not much less invasive than the PAC. The LiDCO device (LiDCO Group, London, UK) estimates cardiac output based on the peripheral arterial pressure waveform pulse power analysis after a lithium dilution calibration, and it has been used successfully in patients recovering from liver transplantation in the ICU (Costa et al, 2008). A device that estimates cardiac output based on the arterial pressure waveform without need for calibration, Flo-Trac/Vigileo (Edwards Lifesciences, Irvine, CA), did not perform well intraoperatively during OLT (Biais et al, 2008; Biancofiore et al, 2009).
Cardiac output determined by a novel suprasternal ultrasound cardiac output monitor (USCOM Pty, Coffs Harbour, Australia) correlates well with the PAC cardiac output (Wong et al, 2008; Su et al, 2008). Other USCOM measurements, such as Doppler estimation of peripheral artery pressures and left ventricular contractility, remain to be validated.
TEE has been gaining more popularity in liver transplantation (Burtenshaw & Isaac, 2006). Data from 2002 estimated use of TEE in only 21% of high-volume centers in the United States (Schuman, 2003), but a more recent survey showed that 86% of anesthesiologists in 40 large-volume U.S. centers use TEE in some or all liver transplantations (Wax et al, 2008).
The main advantages of TEE are that it provides continuous, real-time visualization of cardiac structures and dynamic function. TEE is a superior modality for estimating ventricular volume status, global LV contractility and ejection fraction, right ventricular function, segmental wall motion abnormalities, and septal motion. Doppler modalities allow for interrogation of transvalvular flows, dynamic left ventricular outflow obstruction, intracardiac shunts, and diastolic function. In addition, TEE is the only intraoperative monitor that allows an instantaneous diagnosis of pulmonary or intracardiac thrombus (Ellenberger et al, 2006; Planinsic et al, 2004) and pericardial or pleural effusion. TEE detection of a transpulmonary shunt as a positive bubble test in a patient with HPS is presented in Figure 97B.1.
Monitoring of Hemostasis
Conventional tests of hemostasis, PT and aPTT, have several important limitations (Dalmau et al, 2009; Ng et al, 2009). First, these assays utilize plasma samples devoid of platelets. The activated platelet surface, the naturally occurring medium of the in vivo coagulation, is replaced by addition of phospholipids. The contribution of platelets is thus not considered by these tests. Second, PT and PTT measure only the activity of procoagulant factors and do not take into account the anticoagulant factors. When PT is modified to include anticoagulant factors, thrombin generation in cirrhotic patients is not different from normal controls (Tripodi et al, 2005). The reported higher intralaboratory variability of INR than of PT may be of more relevance for MELD score determination than for acute intraoperative management (Lisman & Leebeek, 2007).
An important shortcoming of the conventional PT and PTT is a long turnaround time, typically about 45 minutes—suboptimal for an ongoing transplant surgery. Point-of-care (POC) assays require only a minimal delay between blood sampling and attaining the results of the test. POC assays of PT and PPT are quick (5 to 15 minutes), cartridge-type, whole-blood tests offered by several manufacturers. An acceptable agreement of such assays with corresponding plasma-based tests has been reported in OLT (Herbstreit et al, 2010), suggesting that the POC tests can effectively replace the slower, conventional testing.
TEG and ROTEM are POC assays that measure viscoelastic properties of whole-blood clot formation and lysis. Both technologies have been used to drive transfusion algorithms (Kang et al, 1985; Coakley et al, 2006). TEG measures time to onset of clot, rate of clot formation, clot tensile strength, and rate of clot lysis (Fig. 97B.2). Treatment of samples with an intrinsic pathway activator, kaolin, shortens clot formation, whereas addition of heparinase dissects the effects of exogenous heparin from intrinsic heparinoids. TEG results are available more rapidly than the conventional laboratory tests. In our institution, progress of TEG clot formation and lysis is displayed on a dedicated operating room monitor and is available to the anesthesiologist in real time.
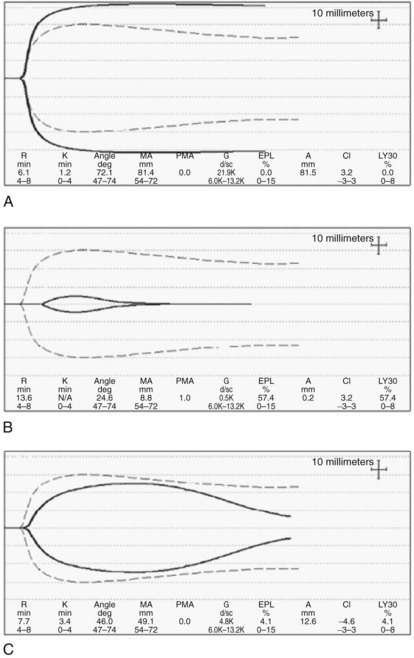
FIGURE 97B.2 Intraoperative thromboelastogram (TEG) recordings from three orthotopic liver transplantation patients with different hemostatic derangements. In each panel, a “normal” TEG trace, generated by the instrument, is presented with an interrupted line. A, Hypercoagulable state in a patient with primary biliary cirrhosis. B,