Chapter 417 Laboratory Evaluation
417.1 Radiologic Assessment
In the posteroanterior view, the left border of the cardiac shadow consists of three convex shadows produced, from above downward, by the aortic knob, the main and left pulmonary arteries, and the left ventricle (Fig. 417-1). In cases of moderate to marked left atrial enlargement, the atrium may project between the pulmonary artery and the left ventricle. The outflow tract of the right ventricle does not contribute to the shadows formed by the left border of the heart. The aortic knob is not as easily seen in infants and children as in adults. The side of the aortic arch (left or right) can often be inferred as being opposite the side of the midline from which the air-filled trachea is visualized. This observation is important because a right-sided aortic arch is often present in cyanotic congenital heart disease, particularly in tetralogy of Fallot. Three structures contribute to the right border of the cardiac silhouette. In the view from above, they are the superior vena cava, the ascending aorta, and the right atrium.
417.2 Electrocardiography
Developmental Changes
The marked changes that occur in cardiac physiology and chamber dominance during the perinatal transition (Chapter 415) are reflected in the evolution of the ECG during the neonatal period. Because vascular resistance in the pulmonary and systemic circulations is nearly equal in a term fetus, the intrauterine work of the heart results in an equal mass of both the right and left ventricles. After birth, systemic vascular resistance rises when the placental circulation is eliminated, and pulmonary vascular resistance falls when the lungs expand. These changes are reflected in the ECG as the right ventricular wall begins to thin.
During the 1st days of life, right axis deviation, large R waves, and upright T waves in the right precordial leads (V3R or V4R and V1) are the norm (Fig. 417-2). As pulmonary vascular resistance decreases in the 1st few days after birth, the right precordial T waves become negative. In the great majority of instances, this change occurs within the 1st 48 hr of life. Upright T waves that persist in leads V3R, V4R, or V1 beyond 1 wk of life are an abnormal finding indicating right ventricular hypertrophy or strain, even in the absence of QRS voltage criteria. The T wave in V1 should never be positive before 6 yr of age and may remain negative into adolescence. This finding represents one of the most important, yet subtle differences between pediatric and adult ECGs and is a common source of error when adult cardiologists interpret pediatric ECGs.
In a newborn, the mean QRS frontal-plane axis normally lies in the range of +110 to +180 degrees. The right-sided chest leads reveal a larger positive (R) than negative (S) wave and may do so for months because the right ventricle remains relatively thick throughout infancy. Left-sided leads (V5 and V6) also reflect right-sided dominance in the early neonatal period, when the R : S ratio in these leads may be <1. A dominant R wave in V5 and V6 reflecting left ventricular forces quickly becomes evident within the 1st few days of life (Fig. 417-3). Over the years, the QRS axis gradually shifts leftward, and the right ventricular forces slowly regress. Leads V1, V3R, and V4R display a prominent R wave until 6 mo to 8 yr of age. Most children have an R : S ratio >1 in lead V4R until they are 4 yr of age. The T waves are inverted in leads V4R, V1, V2, and V3 during infancy and may remain so into the middle of the 2nd decade of life and beyond. The processes of right ventricular thinning and left ventricular growth are best reflected in the QRS-T pattern over the right precordial leads. The diagnosis of right or left ventricular hypertrophy in a pediatric patient can be made only with an understanding of the normal developmental physiology of these chambers at various ages until adulthood is reached. As the left ventricle becomes dominant, the ECG evolves to the characteristic pattern of older children (Fig. 417-4) and adults (Fig. 417-5).
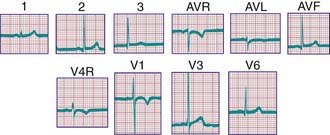
Figure 417-4 Electrocardiogram of a normal child. Note the relatively tall R waves and inversion of the T waves in V4R and V1.
The diagnosis of pathologic right ventricular hypertrophy is difficult in the 1st wk of life because physiologic right ventricular hypertrophy is a normal finding. Serial tracings are often necessary to determine whether marked right axis deviation and potentially abnormal right precordial forces or T waves, or both, will persist beyond the neonatal period (Fig. 417-6). In contrast, an adult electrocardiographic pattern (see Fig. 417-5) seen in a neonate suggests left ventricular hypertrophy. The exception is a premature infant, who may display a more “mature” ECG than a full-term infant (Fig. 417-7) as a result of lower pulmonary vascular resistance secondary to underdevelopment of the medial muscular layer of the pulmonary arterioles. Some premature infants display a pattern of generalized low voltage across the precordium.
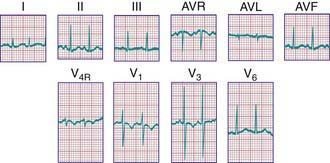
Figure 417-7 Electrocardiogram of a premature infant (weight 2 kg and age 5 wk at the time of the tracing). The cardiovascular system was clinically normal. Left ventricular dominance is manifested by R-wave progression across the chest, similar to tracings obtained from older children. Compare with the tracing from a normal full-term infant (see Fig. 417-3).
P Waves
Tall (>2.5 mm), narrow, and spiked P waves are indicative of right atrial enlargement and are seen in congenital pulmonary stenosis, Ebstein anomaly of the tricuspid valve, tricuspid atresia, and sometimes cor pulmonale. These abnormal waves are most obvious in leads II, V3R, and V1 (Fig. 417-8A). Similar waves are sometimes seen in thyrotoxicosis. Broad P waves, commonly bifid and sometimes biphasic, are indicative of left atrial enlargement (Fig. 417-8B). They are seen in some patients with large left-to-right shunts (ventricular septal defect [VSD], patent ductus arteriosus [PDA]) and with severe mitral stenosis or regurgitation. Flat P waves may be encountered in hyperkalemia.
QRS Complex
Right Ventricular Hypertrophy
For the most accurate assessment of ventricular hypertrophy, pediatric ECGs should include the right precordial lead V3R or V4R, or both. The diagnosis of right ventricular hypertrophy depends on demonstration of the following changes (see Fig. 417-6): (1) a qR pattern in the right ventricular surface leads; (2) a positive T wave in leads V3R-V4R and V1-V3 between the ages of 6 days and 6 yr; (3) a monophasic R wave in V3R, V4R, or V1; (4) an rsR′ pattern in the right precordial leads with the 2nd R wave taller than the initial one; (5) age-corrected increased voltage of the R wave in leads V3R-V4R or the S wave in leads V6-V7, or both; (6) marked right axis deviation (>120 degrees in patients beyond the newborn period); and (7) complete reversal of the normal adult precordial RS pattern. At least 2 of these changes should be present to support a diagnosis of right ventricular hypertrophy.
Abnormal ventricular loading can be characterized as either systolic (as a result of obstruction of the right ventricular outflow tract, as in pulmonic stenosis) or diastolic (as a result of increased volume load, as in atrial septal defects [ASDs]). These two types of abnormal loads result in distinct electrocardiographic patterns. The systolic overload pattern is characterized by tall, pure R waves in the right precordial leads. In older children, the T waves in these leads are initially upright and later become inverted. In infants and children <6 yr, the T waves in V3R-V4R and V1 are abnormally upright. The diastolic overload pattern (typically seen in patients with ASDs) is characterized by an rsR′ pattern (Fig. 417-9) and a slightly increased QRS duration (minor right ventricular conduction delay). Patients with mild to moderate pulmonary stenosis may also exhibit an rsR′ pattern in the right precordial leads.
Left Ventricular Hypertrophy
The following features indicate the presence of left ventricular hypertrophy (Fig. 417-10): (1) depression of the ST segments and inversion of the T waves in the left precordial leads (V5, V6, and V7), known as a left ventricular strain pattern—these findings suggest the presence of a severe lesion; (2) a deep Q wave in the left precordial leads; and (3) increased voltage of the S wave in V3R and V1 or the R wave in V6-V7, or both. It is important to emphasize that evaluation of left ventricular hypertrophy should not be based on voltage criteria alone. The concepts of systolic and diastolic overload, though not always consistent, are also useful in evaluating left ventricular enlargement. Severe systolic overload of the left ventricle is suggested by straightening of the ST segments and inverted T waves over the left precordial leads; diastolic overload may result in tall R waves, a large Q wave, and normal T waves over the left precordium. Finally, an infant with an ECG that would be considered “normal” for an older child may, in fact, have left ventricular hypertrophy.
Bundle Branch Block
A complete right bundle branch block may be congenital or may be acquired after surgery for congenital heart disease, especially when a right ventriculotomy has been performed, as in repair of the tetralogy of Fallot. Congenital left bundle branch block is rare; this pattern is occasionally seen with cardiomyopathy. A bundle branch block pattern may be indicative of a bypass tract associated with one of the pre-excitation syndromes (Chapter 429).
P-R and Q-T Intervals
The duration of the Q-T interval varies with the cardiac rate; a corrected Q-T interval (Q-Tc) can be calculated by dividing the measured Q-T interval by the square root of the preceding R-R interval. A normal Q-Tc should be <0.45. It is often lengthened with hypokalemia and hypocalcemia; in the former instance, a U wave may be noted at the end of the T wave (Fig. 417-11). There are a number of medications that can also lengthen the Q-T interval. A congenitally prolonged Q-T interval (Fig. 417-12) may also be seen in children with one of the long Q-T syndromes. These patients are at high risk for ventricular arrhythmias, including a form of ventricular tachycardia known as torsades de pointes, and sudden death (Chapter 429.5).
ST Segment and T-wave Abnormalities
T-wave inversion may occur in myocarditis and pericarditis, or it may be a sign of either right or left ventricular hypertrophy and strain. Hypothyroidism may produce flat or inverted T waves in association with generalized low voltage. In hyperkalemia, the T waves are commonly of high voltage and are tent-shaped (Fig. 417-13).