Chapter 1 Introduction to the Immune System
• The immune system has evolved to protect us from pathogens. Intracellular pathogens infect individual cells (e.g. viruses), whereas extracellular pathogens divide outside cells in blood, tissues or the body cavities (e.g. many bacteria and parasites). These two kinds of pathogens require fundamentally different immune responses.
• Phagocytes and lymphocytes are key mediators of immunity. Phagocytes internalize pathogens and degrade them. Lymphocytes (B and T cells) have receptors that recognize specific molecular components of pathogens and have specialized functions. B cells make antibodies (effective against extracellular pathogens), cytotoxic T lymphocytes (CTLs) kill virally infected cells, and helper T cells coordinate the immune response by direct cell–cell interactions and the release of cytokines.
• Specificity and memory are two essential features of adaptive immune responses. As a result, the adaptive arm of the immune system (B and T lymphocytes) mounts a more effective response on second and subsequent encounters with a particular antigen. Non-adaptive (innate) immune responses (mediated, for example, by complement, phagocytes, and natural killer cells) do not alter on repeated exposure to an infectious agent.
• Antigens are molecules that are recognized by receptors on lymphocytes. B cells usually recognize intact antigen molecules, whereas T cells recognize antigen fragments displayed on the surface of the body’s own cells.
• An immune response occurs in two phases – antigen recognition and antigen eradication. In the first phase clonal selection involves recognition of antigen by particular clones of lymphocytes, leading to clonal expansion of specific clones of T and B cells and differentiation to effector and memory cells. In the effector phase, these lymphocytes coordinate an immune response, which eliminates the source of the antigen.
• Vaccination depends on the specificity and memory of adaptive immunity. Vaccination is based on the key elements of adaptive immunity, namely specificity and memory. Memory cells allow the immune system to mount a much stronger and more rapid response on a second encounter with antigen.
• Inflammation is a response to tissue damage. It allows antibodies, complement system molecules, and leukocytes to enter the tissue at the site of infection, resulting in phagocytosis and destruction of the pathogens. Lymphocytes are also required to recognize and destroy infected cells in the tissues.
• The immune system may fail (immunopathology). This can be a result of immunodeficiency, hypersensitivity, or dysregulation leading to autoimmune diseases.
• Normal immune reactions can be inconvenient in modern medicine, for example blood transfusion reactions and graft rejection.
• illustrates how the components of the immune system fit together to allow students to grasp the ‘big picture’ before delving into the material in more depth in subsequent chapters;
• introduces the basic elements of the immune system and of immune responses, which are mediated principally by white blood cells or leukocytes (from the Greek for ‘white cell’) and are detailed in Chapters 2–12.
Cells and soluble mediators of the immune system
Cells of the immune system
Immune responses are mediated by a variety of cells and the soluble molecules that these cells secrete (Fig. 1.1). Although the leukocytes are central to all immune responses, other cells in the tissues also participate, by signaling to the lymphocytes and responding to the cytokines (soluble intercellular signaling molecules) released by T cells and macrophages.
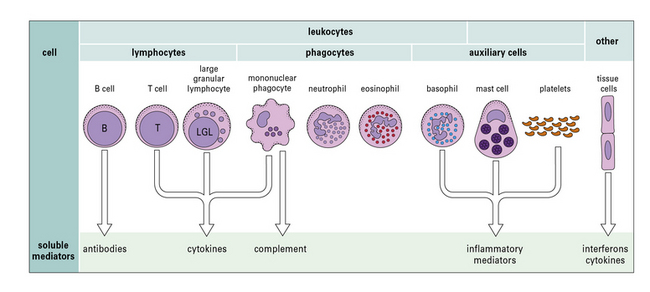
Fig. 1.1 Components of the immune system
The principal cells of the immune system and the mediators they produce are shown. Neutrophils, eosinophils, and basophils are collectively known as polymorphonuclear granulocytes (see Chapter 2). Cytotoxic cells include cytotoxic T lymphocytes (CTLs), natural killer (NK) cells (large granular lymphocytes [LGLs]), and eosinophils. Complement is made primarily by the liver, though there is some synthesis by mononuclear phagocytes. Note that each cell produces and secretes only a particular set of cytokines or inflammatory mediators.
Phagocytes internalize antigens and pathogens, and break them down
The most important long-lived phagocytic cells belong to the mononuclear phagocyte lineage. These cells are all derived from bone marrow stem cells, and their function is to engulf particles, including infectious agents, internalize them and destroy them. To do so, mononuclear phagocytes have surface receptors that allow them to recognize and bind to a wide variety of microbial macromolecules. They can then internalize and kill the micro-organism (Fig. 1.2). The process of phagocytosis describes the internalization (endocytosis) of large particles or microbes. The primitive responses of phagocytes are highly effective, and people with genetic defects in phagocytic cells often succumb to infections in infancy.
To intercept pathogens, mononuclear phagocytes are strategically placed where they will encounter them. For example, the Kupffer cells of the liver line the sinusoids along which blood flows, while the synovial A cells line the synovial cavity (Fig. 1.3).
B cells and T cells are responsible for the specific recognition of antigens
These two classes of lymphocytes carry out very different protective functions:
• B cells are responsible for the production of antibodies that act against extracellular pathogens
• T cells are mainly concerned with cellular immune responses to intracellular pathogens, such as viruses. They also regulate the responses of B cells and the overall immune response.
There are several different types of T cell, and they have a variety of functions (Fig 1.4):
• one group interacts with mononuclear phagocytes and helps them destroy intracellular pathogens – these are called type 1 helper T cells or TH1 cells;
• another group interacts with B cells and helps them to divide, differentiate, and make antibody – these are the type 2 helper T cells or TH2 cells;
• a third group of T cells is responsible for the destruction of host cells that have become infected by viruses or other intracellular pathogens – this kind of action is called cytotoxicity and these T cells are therefore called cytotoxic T lymphocytes (CTLs or TC cells).
Soluble mediators of immunity
Complement proteins mediate phagocytosis, control inflammation and interact with antibodies in immune defense
The complement system, a key component of innate immunity, is a group of about 20 serum proteins whose overall function is the control of inflammation (Fig. 1.5). The components interact with each other, and with other elements of the immune system. For example:
• a number of microorganisms spontaneously activate the complement system, via the so-called ‘alternative pathway’, which is an innate immune defense – this results in the microorganism being opsonized (i.e. coated by complement molecules, leading to its uptake by phagocytes);
• the complement system can also be activated by antibodies or by mannose binding lectin bound to the pathogen surface via the ‘classical pathway’.
• opsonization of microorganisms for uptake by phagocytes and eventual intracellular killing;
• attraction of phagocytes to sites of infection (chemotaxis);
• increased blood flow to the site of activation and increased permeability of capillaries to plasma molecules;
• damage to plasma membranes on cells, Gram-negative bacteria, enveloped viruses, or other organisms that have caused complement activation. This can result in lysis of the cell or virus and so reduce the infection;
Cytokines signal between lymphocytes, phagocytes and other cells of the body
• one group of interferons (IFNα and IFNβ or type-1 interferons) is produced by cells that have become infected by a virus;
IFNs induce a state of antiviral resistance in uninfected cells (Fig. 1.6). They are produced very early in infection and are important in delaying the spread of a virus until the adaptive immune response has developed.
• TH1 cells release one set of cytokines, which promote TH1 cell interactions with mononuclear phagocytes;
• TH2 cells release a different set of cytokines, which activate B cells.
Some cytokines may be produced by all T cells, and some just by a specific subset.
Immune responses to pathogens
Effective immune responses vary depending on the pathogen
The exterior defenses of the body (Fig. 1.7) present an effective barrier to most organisms. Very few infectious agents can penetrate intact skin. In contrast, many infectious agents gain access to the body across the epithelia of the gastrointestinal or urogenital tracts; others, such as the virus responsible for the common cold, infect the respiratory epithelium of nasopharynx and lung; a small number of infectious agents infect the body only if they enter the blood directly (e.g. malaria and sleeping sickness).
Once inside the body, the site of the infection and the nature of the pathogen largely determine which type of immune response will be induced – most importantly (Fig. 1.8) whether the pathogen is:
• an intracellular pathogen (i.e. invades the host cells to divide and reproduce); or
• an extracellular pathogen (i.e. does not invade the host cells).
Many bacteria and larger parasites live in tissues, body fluids, or other extracellular spaces, and are susceptible to the multitude of immune defenses, such as antibodies (see Chapter 3) and complement (see Chapter 4), that are present in these areas. Because these components are present in the tissue fluids of the body (the ‘humors’ of ancient medicine), they have been classically referred to as humoral immunity.
Intracellular pathogens cannot, however, wholly evade the extracellular defenses (see Fig. 1.8) because they must reach their host cells by moving through the blood and tissue fluids. As a result they are susceptible to humoral immunity during this portion of their life cycle.
Innate immune responses are the same on each encounter with antigen
Innate immune responses (see Chapters 6 and 7) can be thought of as simple though remarkably sophisticated systems present in all animals that are the first line of defense against pathogens and allow a rapid response to invasion.
Innate immune response systems range from external barriers (skin, mucous membranes, cilia, secretions, and tissue fluids containing antimicrobial agents; see Fig. 1.7) to sophisticated receptors capable of recognizing broad classes of pathogenic organisms, for example:
• innate immune receptors on certain leukocytes recognize pathogen-associated molecular patterns (PAMPs), which are common to many foreign invaders and are not normally present in the host (e.g. constituents of bacterial cell walls);
• components of the complement system can be specifically activated by bacterial surface molecules.
The innate defenses are closely interlinked with adaptive responses.
Adaptive immune responses display specificity and memory
In contrast to the innate immune response, which recognizes common molecular patterns (PAMPs), the adaptive immune system takes a highly discriminatory approach, with a very large repertoire of specific antigen receptors that can recognize virtually any component of a foreign invader (see Chapters 3 and 5). This use of highly specific antigen receptor molecules provides the following advantages:
• pathogens that lack stereotypical patterns (which might avoid recognition by the innate immune system) can be recognized;
• responses can be highly specific for a given pathogen;
• the specificity of the response allows the generation of immunological memory – related to its use of highly individual antigen receptors, the adaptive immune system has the capacity to ‘remember’ a pathogen.
Specific immunity can, very often, be induced by artificial means, allowing the development of vaccines (see Chapter 18).
Antigen recognition
Antigens are not just components of foreign substances, such as pathogens. A large variety of ‘self’ molecules can serve as antigens as well, provoking autoimmune responses that can be highly damaging, and even lethal (see Chapter 20).
Antibody specifically binds to antigen
Soluble antibodies are a group of serum molecules closely related to and derived from the antigen receptors on B cells. All antibodies have the same basic Y-shaped structure, with two regions (variable regions) at the tips of the Y that bind to antigen. The stem of the Y is referred to as the constant region and is not involved in antigen binding (see Chapter 3).
The two variable regions contain identical antigen-binding sites that, in general, are specific for only one type of antigen. The amino acid sequences of the variable regions of different antibodies, however, vary greatly between different antibodies. The antibody molecules in the body therefore provide an extremely large repertoire of antigen-binding sites. The way in which this great diversity of antibody variable regions is generated is explained in Chapter 3.
Each antibody binds to a restricted part of the antigen called an epitope
Pathogens typically have many different antigens on their surface. Each antibody binds to an epitope, which is a restricted part of the antigen. A particular antigen can have several different epitopes or repeated epitopes (Fig. 1.9). Antibodies are specific for the epitopes rather than the whole antigen molecule.
Fc regions of antibodies act as adapters to link phagocytes to pathogens
The constant region of the antibody (the Fc region) can bind to Fc receptors on phagocytes, so acting as an adapter between the phagocyte and the pathogen (Fig. 1.10). Consequently, if antibody binds to a pathogen, it can link to a phagocyte and promote phagocytosis. The process in which specific binding of an antibody activates an innate immune defense (phagocytosis) is an important example of collaboration between the innate and adaptive immune responses.
Binding and phagocytosis are most effective when more than one type of adapter molecule (opsonin) is present (Fig. 1.11). Note that antibody can act as an adapter in many other circumstances, not just phagocytosis.
Peptides from intracellular pathogens are displayed on the surface of infected cells
For example, a cell infected with a virus will present fragments of viral proteins (peptides) on its surface that are recognizable by T cells. The antigenic peptides are transported to the cell surface and presented to the T cells by MHC molecules (a group of molecules encoded with the Major Histocompatibility Complex, see Chapter 5). T cells use their antigen-specific receptors (T cell receptors – TCRs) to recognize the antigenic peptide–MHC molecule complex (Fig. 1.12).
APCs not only display antigenic peptide–MHC complexes on their surface, but also express co-stimulatory molecules that are essential for initiating immune responses (see Chapter 8). Co-stimulatory signals are upregulated by the presence of pathogens, which can be detected by the engagement of innate immune receptors that recognize PAMPs.
• in the earliest stages of infection, innate responses predominate;
• later the lymphocytes start to generate adaptive immune responses;
• after recovery, immunological memory remains within the population of lymphocytes, which can then mount a more effective and rapid response if there is a reinfection with the same pathogen at a later date.
Antigen activates specific clones of lymphocytes
How then is an adequate immune response to an infectious agent generated? The answer is that, when an antigen binds to the few lymphocytes that can recognize it, they are induced to proliferate rapidly. Within a few days there is a sufficient number to mount an adequate immune response. In other words, the antigen selects and activates the specific clones to which it binds (Fig. 1.13), a process called clonal selection. This operates for both B cells and T cells.
How can the immune system ‘know’ which specific antibodies will be needed during an individual’s lifetime? It does not know. The immune system generates antibodies (and T cell receptors) that can recognize an enormous range of antigens even before it encounters them. Many of these specificities, which are generated more or less at random (see Chapters 3 and 5), will never be called upon to protect the individual against infection.
Antigen elimination
Antigen elimination involves effector systems
Phagocytosis is promoted by opsonins
More often antibody activates complement or acts as an opsonin to promote ingestion by phagocytes. Phagocytes that have bound to an opsonized microbe, engulf it by extending pseudopodia around it. These fuse and the microorganism is internalized (endocytosed) in a phagosome. Granules and lysosomes fuse with the phagosome, pouring enzymes into the resulting phagolysosome, to digest the contents (Fig. 1.14).
Termination of immune responses limits damage to host tissues
To clear the offending pathogen immune responses are often massive, with:
These responses, if left unchecked, can also damage host tissues.
Antigen elimination can be a slow process, however, so the immune system also employs a variety of active mechanisms to downregulate responses, as discussed in Chapter 11.
Immune responses to extracellular and intracellular pathogens differ
In dealing with intracellular pathogens, the immune system has two options:
• T cells can destroy the infected cell (i.e. cytotoxicity); or
• T cells can activate the infected cell to deal with the pathogen itself (e.g. helper T cells release cytokines, which activate macrophages to destroy the organisms they have internalized).
Consequently, antibody is important in limiting the spread of infection and preventing reinfection with the same virus, while CTLs are essential to deal with infected cells (Fig. 1.15). These factors play an important part in the development of effective vaccines.
Vaccination
The study of immunology has had its most successful application in vaccination (see Chapter 18), which is based on the key elements of adaptive immunity, namely specificity and memory. Memory cells allow the immune system to mount a much stronger response on a second encounter with antigen. Compared with the primary response, the secondary response is:
Take, for example, vaccination against tetanus. The tetanus bacterium produces a toxin that acts on receptors to cause tetanic contractions of muscle. The toxin can be modified by formalin treatment so that it retains its epitopes, but loses its toxicity. The resulting molecule (known as a toxoid) is used as a vaccine (Fig. 1.16).
Inflammation
• increased blood supply to the infected area;
• increased capillary permeability due to retraction of the endothelial cells lining the vessels, permitting larger molecules than usual to escape from the capillaries;
• migration of leukocytes out of the venules into the surrounding tissues – in the earliest stages of inflammation, neutrophils are particularly prevalent, but in later stages monocytes and lymphocytes also migrate towards the site of infection or damage.
Leukocytes enter inflamed tissue by crossing venular endothelium
The process of leukocyte migration is controlled by chemokines (a particular class of cytokines) on the surface of venular endothelium in inflamed tissues. Chemokines activate the circulating leukocytes causing them to bind to the endothelium and initiate migration across the endothelium (Fig. 1.17).
A particularly active chemotactic molecule is C5a, which is a fragment of one of the complement components (Fig. 1.18) that attracts both neutrophils and monocytes. When purified C5a is applied to the base of a blister in vivo, neutrophils can be seen sticking to the endothelium of nearby venules shortly afterwards. The cells then squeeze between the endothelial cells and move through the basement membrane of the microvessels to reach the tissues. This process is described more fully in Chapter 6.
Immunopathology
In essence the immune system can fail in one of three ways (Fig. 1.19), resulting in autoimmunity, immunodeficiency, or hypersensitivity.
Inappropriate reaction to self antigens – autoimmunity
Normally the immune system recognizes all foreign antigens and reacts against them, while recognizing the body’s own tissues as ‘self’ and making no reaction against them. The mechanisms by which this discrimination between ‘self’ and ‘non-self’ is established are described in Chapter 19.
When the immune system reacts against ‘self’ components, the result is an autoimmune disease (see Chapter 20), for example rheumatoid arthritis or pernicious anemia.
Overactive immune response – hypersensitivity
Sometimes immune reactions are out of all proportion to the damage that may be caused by a pathogen. The immune system may also mount a reaction to a harmless antigen, such as a food molecule. Such immune reactions (hypersensitivity) may cause more damage than the pathogen or antigen (see Chapters 23–26). For example, molecules on the surface of pollen grains are recognized as antigens by particular individuals, leading to the symptoms of hay fever or asthma.
Normal but inconvenient immune reactions
Critical thinking: Specificity and memory in vaccination (see p. 433 for explanations)
Schedules for vaccination against tetanus and influenza A
1. Why is it necessary to vaccinate against tetanus only every 10 years, though antibodies against the toxoid disappear from the circulation within a year?
2. Why is the vaccine against tetanus always effective, whereas the vaccine against influenza protects on some occasions but not others?
3. Why is tetanus recommended for everyone and influenza for only a restricted group of ‘at-risk’ individuals, even though influenza is a much more common disease than tetanus?