Chapter 13 Intervertebral Disc Process of Degeneration
Physiology and Pathophysiology
In the United States alone, nearly 5.7 million people are diagnosed with intervertebral disc disorders each year.1,2 These disorders are responsible for widespread disability and account for tremendous costs associated with their treatment and loss of productivity. The intervertebral disc is a unique multifunctional structure. It provides a major stabilizing effect for the spine and allows for the dissipation of axial load, and yet is still flexible enough to confer significant mobility. Despite these essential roles in spinal mechanics, the disc also begins to show signs of biochemical and structural change almost immediately after birth, and signs of degeneration are present at an extremely young age.3–5 Unfortunately, these degenerative changes are often progressive, rendering the disc biochemically and biomechanically incapable of absorbing the forces necessary for normal physiologic spinal function and potentially leading to rapid degeneration of the spine.
Understanding the anatomy and function of the normal disc serves as the framework for comprehension of the complex processes of aging and the pathologic degeneration that afflict the disc. One of the most significant challenges to a complete understanding of disc disease is the lack of consistency with which radiographically detectable signs of disc degeneration translate to clinical symptomatology.6 As such, there is abundant evidence to suggest that aberrant disc morphology does not necessarily equate with back pain.6,7 This poses a significant challenge to researchers in this field because individual variation in disc degeneration makes it difficult to distinguish between age-related alterations and pathologic premature degenerative changes.5,8 In the future, the ability to make this distinction will lead to improved clarity regarding the complex process of disc degeneration.
Normal Intervertebral Disc
The intervertebral disc is composed of water, proteoglycan, collagen, and a small amount of noncollagenous proteins. There are three major regions to the intervertebral disc with differing structural compositions: the nucleus pulposus, anulus fibrosus, and cartilaginous end plates. Each has different functions. Of significant note, the intervertebral disc is one of the most hypocellular structures in the body9 (Fig. 13-1).
Anulus Fibrosus
The anulus fibrosus is the outer circumferential portion of the disc and is often subdivided into the inner and outer anulus. The outer anulus is a collection of highly organized, densely packed collagen fibrils, creating a lamellar structure of up to 25 lamellae in the mature disc.9,10 These collagen fibrils insert into the adjacent vertebral end plates and join them together to stabilize the spine. In addition to its predominantly collagenous structure, a small amount of elastin is present in the anulus, which helps it to restore and recoil after stretching in response to compression and tensile forces.9,11 The strong anulus provides the ability to absorb significant hoop stresses and maintain stiffness in the presence of the tensile forces induced by bending and twisting of the spine.12,13 The importance of this ability to resist hoop stress has been shown in large animal studies, which demonstrated that even a partial-thickness laceration into the anulus rapidly produced advanced disc degeneration.12,14 The outer anulus is populated with a low density of fibroblast-like cells.15 It is the only tissue in the mature disc that remains innervated and is capable of producing pain when exposed to noxious stimuli.16–19
The inner anulus appears to serve as a transition zone to the vastly different biochemical composition of the nucleus. It is less dense and lacks the organized lamellar structure of the outer anulus. It is populated with rounder, chondrocyte-like cells, and the concentration of proteoglycans and water content is increased.20
Nucleus Pulposus
The nucleus pulposus, the inner, less-organized region of the disc, occupies the middle and posterior thirds of the intervertebral disc.21 It consists of a rich proteoglycan matrix with a lesser amount of disorganized collagen than in the anulus.20,22 There is a significantly higher content of water in the nucleus. The large amount of proteoglycan and water present in the nucleus make it ideal for developing the “swelling pressures” necessary to resist the compressive forces of the spine. At birth, the nucleus is populated almost exclusively with notochordal cells. These cells rapidly begin to necrose shortly after birth and by the age of 4 to 10 years they are completely replaced by rounder, chondrocyte-like cells of mesenchymal origin9 (Table 13-1).
Component | Anulus Fibrosus | Nucleus Pulposus |
---|---|---|
Collagen (mainly types I and II; others present include types III, V, VI, IX, XI, XII, and XIV) | 70% of dry weight 80% type I collagen |
20% of dry weight 80% type II collagen |
Proteoglycan | 25% of dry weight | 50%–70% of dry weight |
Water | Lowest content | Highest content |
Cartilaginous End Plate
The cartilaginous end plate is a hyaline cartilage layer separating the disc from the adjacent bony vertebral end plate. It is usually less than 1 mm thick in the mature disc and lies immediately adjacent to the perforated bony end plate. It is loosely cemented to the underlying bony end plate by a thin layer of calcium that is absent near the perforated areas of the bony plate, which allows for nutrient diffusion from the vertebra to the disc.21
Matrix of the Intervertebral Disc
The matrix content of the intervertebral disc is critical to disc function. The uniquely hydrophilic nature of proteoglycans provides the swelling pressure required to increase the intradiscal water content necessary to resist compressive strains on the spine.9,23,24 The matrix composition changes with age, and the quantitative loss and qualitative deterioration of the proteoglycans is thought to represent the single largest biochemical contribution to disc degeneration.20,25
Proteoglycans are highly negatively charged molecules consisting of a core protein and chains of glycosaminoglycans radiating from the core. The chains are composed mainly of keratin and chondroitin sulfate. Multiple proteoglycans then are linked to hyaluronic acid to form large proteoglycan aggregates capable of producing even larger swelling pressures. The aggregates are held together by type II collagen and are cross-linked by type IX collagen.23,26
Aggrecan is the most abundant proteoglycan in the disc.20 It is responsible for 70% of the dry weight of the nucleus pulposus and 25% of the anulus fibrosus. Other proteoglycans present at lower levels include decorin, biglycan, fibromodulin, lumican, versican, and perlican.20,27
Nutrition of the Intervertebral Disc
The intervertebral disc is the largest avascular tissue in the human body. Nutritional support for cell survival, matrix production, and disc homeostasis occurs through diffusion of nutrients through the vertebral end plates.28–30 In the newborn and young child, small blood vessels penetrate the cartilaginous end plates and enter the disc anulus and nucleus.21 These vessels rapidly become less efficient, yet remain partially functional until about 8 years of age. They then become completely nonfunctional over the next two decades of life.5,21,28,31,32
The vertebral body is supplied by an arterial network that drains into a subchondral venous plexus within the bone.28,33 Capillaries from this region penetrate into the subchondral plate and terminate in loops at the bony end plate–cartilaginous end plate junction.28,34,35 Through these capillaries, the nutrients necessary for disc metabolism are delivered and diffuse through the cartilaginous end plates to reach the center of the disc.28 The end plate acts as a semipermeable membrane, letting smaller neutral charges (water, amino acids, oxygen) through more easily than larger, positively charged molecules28,36–38 (Fig. 13-2).
Modulation and Regulation of Disc Matrix
Anabolic Influences
The intervertebral disc matrix is in a constant state of dynamic flux. Anabolic and catabolic influences on the disc matrix continuously interact with the disc to alter the physical composition of the matrix.23 Metalloproteinases (MMPs) modulate the matrix composition by breaking down aged proteoglycans under catabolic influences while newly synthesized matrix takes the place of the degraded proteoglycans.23,39 A very complex, multifactorial, poorly understood interaction of mechanical and biochemical factors defines whether a disc is capable of remodeling in response to loading conditions in order to maintain disc homeostasis.1 If the disc is unable to remodel and maintain a balance of anabolic and catabolic influences, then damage accumulates in a process that may eventually self-perpetuate into a state of continued degeneration.
The key anabolic modulating growth factors work by stimulating the chondrocyte-like cells to produce matrix while inhibiting the MMPs present in the disc.1 In addition to various growth factors, tissue inhibitors of MMPs (TIMPs) are present to suppress the activation of the MMPs23,40 (Table 13-2).
TABLE 13-2 Key Anabolic Modulators (Growth Factors) of the Intervertebral Disc
Growth Factor | Role |
---|---|
Insulin-like growth factor-1 (IGF-1) | Stimulates proteoglycan synthesis Reduces cellular apoptosis |
Epidermal growth factor (EGF) | Stimulates matrix synthesis Cellular proliferation |
Basic fibroblast growth factor (bFGF) | Stimulates proteoglycan synthesis Cellular proliferation |
Platelet-derived growth factor (PDGF) | Decreases cellular apoptosis |
Transforming growth factor beta (TGF-β) | Greatly increased proteoglycan synthesis Cellular proliferation |
Bone morphogenetic protein-2 (BMP-2) | Increased proteoglycan synthesis Cellular proliferation Increased mRNA for type II collagen, aggrecan, sox9, and osteocalcin Up-regulates gene expression of BMP-6, BMP-7, and TGF-β |
Bone morphogenetic protein-7 (BMP-7; osteogenic protein-1 [OP-1]) | Increased proteoglycan synthesis Increased collagen synthesis Cellular proliferation |
Link protein N-terminal peptide | In concentrations of 10–100 μg/mL stimulates matrix assembly |
From Masuda K, Oegema TJ, An H: Growth factors and treatment of intervertebral disc degeneration. Spine (Phila Pa 1976) 29:2757, 2004.
Catabolic Influences
Catabolic modulation in the disc occurs mainly through cytokine-induced and metalloproteinase degradation of matrix components.23 MMPs 1, 2, 3, 8, 9, and 13 are all present in the disc and serve to degrade collagen, aggrecan, versican, link protein, and the other proteoglycans.9,41–45 The aggrecanases ADAM-TS-4 and ADAM-TS-5, from the ADAM (a disintegrin and metalloproteinase) protein family, are also present in the disc and mainly function to degrade aggrecan and versican.9,46 These MMPs and aggrecanases allow for the large proteoglycans to be degraded into smaller components that may be more easily leached from the intervertebral disc by diffusion.20 In a pathologic catabolic state when the MMPs are working with little opposing matrix production, a significant net loss of proteoglycan and water content occurs, rendering the disc incapable of producing the swelling pressure necessary to resist compressive forces on the disc.20 Overproduction of MMPs can thus lead to increased forces on other spinal structures and contribute to disc and overall spinal degeneration.
In addition to the catabolic effect of MMPs, growing evidence suggests that inflammatory cytokines may have a significant catabolic influence on the disc.23,47,48 Animal studies have indicated that in the presence of injury to the intervertebral disc there are increased levels of interleukin (IL)-1, tumor necrosis factor alpha (TNF-α), and IL-8 near the injured regions of the disc.49 These cytokines have negative catabolic effects on the disc and also stimulate the disc cells to produce chemokines, which are responsible for macrophage attraction and stimulation, especially in the presence of disc herniation.48,50 Macrophages in the disc usually reflect an attempt to break up or remove herniated disc fragments, but their presence also leads to further inflammation and cytokine release, which may prove damaging to the disc.23,51 These inflammatory substances are also responsible for the activation of multiple cascades within the disc cell nuclei that lead to the production of metalloproteinases, which in turn contribute to matrix breakdown.48,52 In addition, these inflammatory cytokines are thought to contribute to the pain-producing effects of disc degeneration48,49,53,54 (Table 13-3).
TABLE 13-3 Key Catabolic Modulators of the Intervertebral Disc
Catabolic Modulators | Actions |
---|---|
Interleukin-1 (IL-1) | Inhibits synthesis of matrix Promotes metalloproteinase production Stimulates nitric oxide synthetase to produce nitric oxide Enhances caseinase activity Triggers the translocation of transcription factors to cell nuclei that target genes that include collagenases and COX-2 |
Tumor necrosis factor alpha (TNF-α) | Inhibits synthesis of matrix Promotes metalloproteinase production Stimulates nitric oxide synthetase to produce nitric oxide Triggers the translocation of transcription factors to cell nuclei that target genes that include collagenases and COX-2 |
Nitric oxide | Produces free radicals that damage cell membranes and induce degenerative pathways Inhibits tissue inhibitors of metalloproteinases (TIMPs) |
Interleukin-6 and interleukin-9 (IL-6, IL-9) fibronectin superoxide | Proinflammatory and pro-nociceptive effect stimulates proteoglycan degradation Degrades hyaluronic acid, causing aggregate breakdown |
COX-2, cyclooxygenase-2.
Data from references 1, 9, 48; and Anderson DG, Tannoury C: Molecular pathogenic factors in symptomatic disc degeneration. Spine J 5(6 Suppl):260S, 2005.
Degeneration
It is very difficult to differentiate the normal degenerative changes of an aging disc from those of pathologic disc degeneration.5,8 It is also crucial to understand that even pathologic disc degeneration is not always accompanied by back pain, and therefore degeneration as discussed here does not necessarily translate to clinical symptomatology. The biochemical and cellular structure of the intervertebral disc begins changing almost immediately after birth, and the process progresses through life.3–5 It is useful to divide disc changes into the following two categories:
Gross and Histologic Changes
The hallmark histologic finding in intervertebral disc degeneration is the progressively diminishing vascular supply, which begins shortly after birth and leads to tissue breakdown as early as the second decade of life.5 Changes in the nucleus pulposus also begin immediately after birth. Within the first two decades of life the nucleus begins to undergo mucoid degeneration, increasing cleft formation, and granular changes consistent with granulation tissue formation at sites of disc injury. These clefts and granular changes eventually lead to frank tears that communicate with anular rim lesions.5,21
In the anulus, peripheral rim lesions appear late in the second decade of life and eventually progress to frank tears that communicate with the nuclear clefts. The end plate also shows degenerative changes that begin with the loss of the end plate vessels early in childhood. After the loss of these vessels, cartilage disorganization increases and cracks in the cartilaginous end plate begin to appear. Microfractures in the adjacent subchondral bone follow and new subchondral bone formation and eventual sclerosis of the plate occur. All of these changes eventually lead to a “burned-out” state,5 apparent in the seventh and later decades of life. At this time, all components of the disc begin to resemble scar tissue and there is no longer a distinction between the different regions of the disc5,21 (Table 13-4 and Figs. 13-3 to 13-6).
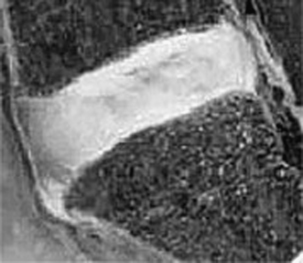
FIGURE 13-3 Sagittal section through a normal intervertebral disc.
(From Thalgott J, Albert T, Vaccaro A, et al: A new classification system for degenerative disc disease of the lumbar spine based on magnetic resonance imaging, provocative discography, plain radiographs and anatomic considerations. Spine J 4[6 Suppl]:167S, 2004.)
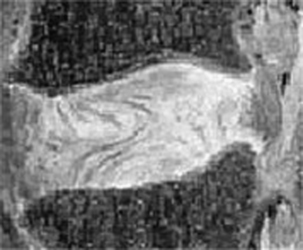
FIGURE 13-4 Sagittal section through a disc with mild degeneration.
(From Thalgott J, Albert T, Vaccaro A, et al: A new classification system for degenerative disc disease of the lumbar spine based on magnetic resonance imaging, provocative discography, plain radiographs and anatomic considerations. Spine J 4[6 Suppl]:167S, 2004.)
The cellular content of the disc also changes over time. The earliest changes occur shortly after birth and include replacement of the rapidly decaying notochordal cells with mesenchymal chondrocyte-like cells.9 Cell density in both the nucleus and the anulus also rapidly declines after birth. This occurs as the matrix grows along with the expanding volume of the intervertebral disc. The cell density in the nucleus pulposus shortly after birth is approximately 4 × 106 cells/cm3 and in the anulus fibrosus, 9 × 106 cells/cm3. This is significantly lower then the cellular density of even relatively acellular hyaline cartilage,9 which has about 1.4 × 107 cells/cm3. As the disc continues to age, cell death accelerates and large numbers of decaying cells can be found in clusters. By adulthood, more than 50% of the disc’s cells are necrotic55 (Fig. 13-7 and Table 13-5).

FIGURE 13-7 Midsagittal view on T2 density-weighted MRI of discs graded according to a modified Pfirrmann classification (see Table 13-5).
(From Hangai M, Kaneoka K, Kuno S, et al: Factors associated with lumbar intervertebral disc degeneration in the elderly. Spine J 8:732, 2008.)
TABLE 13-5 Modified Pfirrmann Classification as Evaluated on Midsagittal T2-Density-Weighted MRI
Grade | Signal Intensity of Nucleus Pulposus | Distinction between Nucleus and Anulus |
I | Hyperintense or isointense to CSF (bright white) and homogeneous | Clear |
II | Hyperintense of isointense to CSF (white) and inhomogeneous | Clear |
III | Intermediate to CSF (light gray) and inhomogeneous | |
IV | Hypointense to CSF (dark gray) and inhomogeneous | |
V | Low intense to CSF (black) and inhomogeneous |
CSF, cerebrospinal fluid.
Adapted from Hanagai M, Kaneoka K, Kuno S, et al: Factors associated with lumbar intervertebral disc degeneration in the elderly. Spine J 8:732, 2008.
Biochemical Changes
The most significant biochemical change in the degenerating disc is the net loss of proteoglycan.20,25 In addition to this quantitative loss of proteoglycan, the quality of the remaining proteoglycan is compromised.9,27,56 With the loss of large quantities of aggregated proteoglycan, the disc’s ability to create the swelling pressure necessary to absorb the forces imposed on it decreases. These changes also begin shortly after birth, when the rich proteoglycan matrix composition is altered. At birth, chondroitin sulfate is the predominant glycosaminoglycan in the disc, but as the blood supply is progressively lost and the disc volume increases, the disc contents become increasingly anaerobic. Because of the lack of oxygen, chondroitin sulfate is progressively replaced by keratan sulfate chains, which are created in the absence of oxygen.9,57–59
In addition to the changing glycosaminoglycan composition in the disc, the overall structure of the large proteoglycans changes. In the young disc, aggrecan is the predominant proteoglycan. Multiple aggrecan molecules bind to hyaluronan to form an even larger structure called an aggregate. In the fetal and newborn disc, aggregating proteoglycans predominate and are extremely effective in creating large swelling pressures.9,60,61 The amount of aggregating proteoglycan is rapidly diminished with age by proteolytic degradation of the large aggregates. By 6 months of age, only 50% of the anular and 30% of the nuclear proteoglycan content is in aggregated form. In the mature adult disc, only 10% of the proteoglycan is aggregated and these aggregates are smaller and have a higher keratan sulfate composition compared with their younger equivalents.27,62 These degenerative features of disc proteoglycans may make them less effective in creating the swelling pressure necessary to resist compressive forces9 (Fig. 13-8).
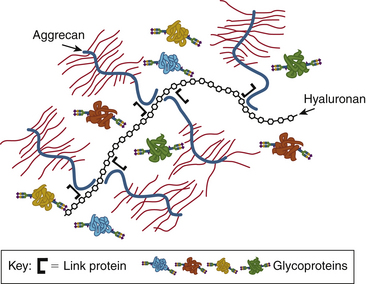
FIGURE 13-8 The large aggrecan molecule forms an aggregate with hyaluronan and link protein.
(From Esko JD, Kimata K, Lindahl U: Proteoglycans and sulfated glycosaminoglycans. In Varki A, Cummings RD, Esko JD, et al., editors: Essentials of glycobiology, ed 2, Cold Spring Harbor, NY, 2009, Cold Spring Harbor Laboratory Press. Online text version available at www.ncbi.nlm.nih.gov/books/NBK1900/.)
The collagen content of the disc is not immune to change, also exhibiting signs of degeneration. The disc collagen demonstrates evidence of proteolytic collagenase action, which accumulates and eventually contributes to the weakening mechanical properties of the disc.9,57,63 It is significant that the triple helix structure of disc collagen is more denatured and damaged than that of the collagen in articular cartilage, which may indicate less effective repair mechanisms in the disc.57 In addition, the collagen present in the disc appears to be nonenzymatically cross-linked by advanced glycation end products (AGEs).9,64–66
AGEs are protein modifiers formed through the Maillard reaction when an amino acid and a reducing sugar react.66 With age, AGEs accumulate in various tissues in the body such as the skin, myocardial cells, renal cells, chondrocytes, and intervertebral discs.66–70 AGEs originally were given substantial attention because of their increased presence in diabetes.71 After research elucidated the presence and negative effect of AGEs on articular cartilage, their presence and potential role in intervertebral disc degeneration were sought.66,72,73 in vitro and animal studies suggest that AGEs play a role in disc degeneration. Their increased concentration in diabetes may contribute to the increased relative risk for disc herniation seen in at least one study of patients with diabetes.74
AGEs likely influence disc degeneration through negative biologic and biomechanical contributions. Biomechanical studies have demonstrated that the accumulation of AGEs leads to alterations in the disc’s physical properties, the most significant of which is increased mechanical stiffness.75 This increased stiffness of the disc also likely leads to a decreased ability to produce the swelling pressure necessary for normal disc function.9,69 Biologic in vitro studies have also demonstrated that the accumulation of AGEs in intervertebral disc cells leads to a down-regulation in the production of aggrecan.66 In addition, a recent bovine study documented the increased expression of the receptor for AGEs (RAGE) in intervertebral discs in the presence of an inflammatory state with increased IL-1β. All of these studies noted a decrease in aggrecan production, which may at least partially explain the increased disc degeneration seen in the presence of chronic inflammation.48,76
Disc Material Properties and Disc Degeneration
The gross structural and biochemical changes associated with early degeneration lead to changes in the intervertebral disc’s mechanical properties, which make it less capable of performing its necessary functions.77 Cadaveric studies conducted on discs of various ages and degrees of degeneration have demonstrated an increased shear modulus in aged and degenerated discs, indicating a decreased ability of the disc to absorb energy.77–79 In essence, the discs become increasingly stiff and appear to undergo a transition from “fluid-like” behavior to “solid-like” behavior with increasing degeneration.80 Alterations in disc viscoelasticity have also been seen, including both increased creep and creep rate. In addition to these changes, which more drastically affect the nucleus, alterations in the anulus result in a decreased Poisson’s ratio as well as decreased radial permeability, which transforms the anisotropic hydraulic permeability of the normal disc to a more isotropic permeability in degenerated discs81 (Box 13-1).