Chapter 11 Interventional Ultrasound
General Principles of Ultrasound-Guided Interventions
Human anatomy is typically more complicated than the remnants of gross anatomy class that most nonsurgical physicians carry around in their heads. Between the surface and the target structures of nerve or muscle, there are not only multiple types of tissues with different ultrasonographic signatures, but also a variety of interposed blood vessels, nerves, and lymphatic structures, some of which are apparent by ultrasound. Furthermore, anatomic relationships vary with patient size, positioning, and bone structure and anatomic variants are common. To that end, the first phase of all ultrasound-guided interventions is preprocedural examination of the area of interest. This allows the clinician to visualize the target tissue and analyze surrounding structures in three dimensions. The diligent examiner uses this time to address three critical questions for needle-based interventions: (1) is the target well delineated; (2) are there anatomic variants or pathologic changes present that might complicate the procedure; and (3) what is the optimal pathway for a needle to reach the target with the least risks of complications and discomfort for the patient? These questions warrant further elaboration.
Visualization of the target implies that the examiner can fully image the site of eventual needle placement and the pathway leading up to it. Furthermore, it implies that the needle should be visible during its trajectory, if not throughout its entire passage, at least in areas where the needle is in proximity to other vital structures. If the probe is positioned to obtain a cross-sectional image of an inserted needle, the needle appears as only a single point, and the technique cannot distinguish the tip of the needle from its shaft, except at the point where the needle first appears while advancing it (or disappears while retracting it). The probability of error using this method can be reduced somewhat by needle or probe movement back and forth, observation of local tissue movement by such maneuvers, or the injection of a small test dose of material that might be apparent by ultrasound. More information about the tip of the needle is available by in-plane or an image in the sagittal plane of the needle in which the needle is advanced in-plane with the long axis of the ultrasound transducer (Fig. 11.1 and Videos 11.1, 11.2, and 11.3).

Fig. 11.1 Images of electromyography (EMG) needles in a convenient phantom, an uncooked pork loin. A and B are in-plane views of the needle, with the probe parallel to the direction of the needle. A, A Teflon-coated EMG injection needle, with an uninsulated beveled tip; this characteristic is captured better on this image than on the direct photographs of the needles in Figure 11.2. B, An 18-gauge hypodermic needle with a ring-down–type artifact (this image results from the sound waves reverberating once in the hypodermic compartment before being transmitted back to the transducer). C, Six different needle types in cross section with an out-of-plane view, probe perpendicular to the needle. These are an EMG injection needle, a monopolar EMG needle, a concentric EMG needle, an 18-gauge needle, an 18-gauge needle that has been sandpapered slightly, and the needle from an insulin syringe. Note that the size of the footprint in this image is relatively independent of needle thickness. D, 18-gauge needles show different ring-down artifacts because they are inserted at slightly different angles to the plane of the surface of the phantom.
The presence of pathologic changes and anatomic variants should be apparent on the screening examination. Of course, an informed user should have a heightened suspicion of pathology based on preprocedural clinical assessment of the patient, and should be sufficiently educated on possible anatomic variants so as to be able to deal with the majority of them. This requires specific acquired knowledge of the target area and its surroundings. For example, injectors of the median nerve at the wrist should be aware of common variants such as bifid median nerves and persistent median arteries (see Chapter 5). For those using ultrasound to guide needle biopsy of muscle, an awareness of myopathies associated with particularly echogenic tissue changes (such as inclusion body myopathy) is helpful in that these changes can cause difficulty precisely locating the needle for biopsy.
In evaluating the optimal pathway for passing the needle to the target, the clinician may benefit from having experience with more than one approach to the structure of interest. Anomalous blood vessels, anatomic variants, or scar tissue may present problems amenable to selection of an alternate pathway. The clinician should also be aware of other imaging modalities (computed tomography [CT] or fluoroscopy) that might be available for guiding needle placement should ultrasound prove inadequate, and be ready to refer the patient for such procedures or consider referral for interventions when appropriate. Also compatible with concurrent ultrasound imaging is the use of EMG guidance. EMG guidance needles are monopolar hypodermic needles that are covered with a thin electrical insulator (e.g., Teflon) up to the bare tip, which serves as a recording electrode (Fig. 11.2). As this needle tip is passed through muscle, it generates an injury potential that creates a distinct high-pitched audible sound known as insertional rip on an EMG instrument. This type of needle monitoring is a useful adjunct when injecting tissue near muscles, because insertional activity is unique to muscle and its presence on entering, and absence when leaving muscle provides valuable localizing information about the needle tip. The needle tip can also be used for stimulation, which can help with localization.

Fig. 11.2 Images of the hypodermic needles used in Figure 11.1. A, Left to right: 30-gauge needle, 10-mm length; insulin syringe, 15-mm length; 18-gauge needle, 35-cm length; Teflon-coated electromyography (EMG) injection needle, 35-mm length; and 21-gauge needle, 45-mm length. B, The EMG injection needle (Myoject), compared with a Teca monopolar EMG needle, with a shaft coated to its tip, and a TECA concentric EMG needle, with a bare shaft. All three needles are 35 mm in length. Note the slightly greater thickness of the injection needle. C and D, Zoom images of the tip of the Teflon-coated injection EMG injection needle (Myoject) and monopolar EMG needle shown in B.
Ultrasound Guidance for Steroid Injections for Carpal Tunnel Syndrome
Steroid injections into the carpal tunnel are helpful for treating symptoms of the disorder. In some series a significant percentage of treated patients achieve meaningful results that last for up to 7 years or longer.1 However, like most interventional procedures, it is difficult to acquire an acceptable evidence base because of inconsistent variations in technique, drug dose, concentration, and combinations of drug with local anesthetics, and lack of a standard, in many published studies, to guarantee proper location of the injection. Further complicating the process is the absence of a strong financial interest from pharmaceutical companies to fund such research, because the cost of the drugs used is far too little to justify funding expensive clinical trials. The advent of widely available high-resolution ultrasound helps address the concern about accurate injection placement and makes the technique more available to those with a good working knowledge of the anatomy and familiarity with ultrasound technique. More definitive studies will be welcome.
Because it is likely that many neuromuscular medicine physicians will see and diagnose carpal tunnel syndrome (CTS) in a laboratory setting, it is appropriate to discuss CTS injection techniques in detail. Standard, blind techniques for injecting the carpal tunnel involve identifying either the flexor carpi radialis tendon or palmaris longus tendon at the wrist and inserting a needle adjacent to the tendon and advancing the needle at a 30- to 45-degree angle to the skin toward the median nerve. The injection is made just proximal to the distal wrist crease, and the angle of approach is determined by anticipated location of the median nerve under the flexor retinaculum. Some techniques involve starting 1 cm proximal to the distal wrist crease, others start closer and adjust the needle angle accordingly.2–4 Injuries from blind techniques for steroid injections do occur but are rare,1 perhaps less than 0.1%, a figure much lower than that caused by surgery;1,5 relief begins in just a few days for most patients.2
Ultrasound guidance can be used to monitor the procedure, insure proper localization of the injectate, and avoid intraneural injection. Two approaches are commonly used, although there are probably a number of variations that could be considered as well. The nerve can be approached in a fashion similar to the blind techniques. The proximal edge of the transducer can be positioned over the nerve at the distal wrist crease in a sagittal plane. This frees up the area just proximal to the distal wrist crease. Using the tendons as surface landmarks that correspond to their visual location and relationship to the median nerve, a site 1-cm proximal to the distal wrist crease can be selected, the needle placed just ulnar to the palmaris longus tendon, and then advanced at an angle toward the nerve (Fig. 11.3 and Video 11.4).6 With ultrasound the angle of approach does not have to be arbitrary, but can be mapped out visually by rotating the probe slightly toward the advancing needle, where it can be followed to a position just lateral or superficial to the nerve where the injection can be given. The median nerve depth is shallow at this location.
Another approach using ultrasound has been described (Fig. 11.4).7 In this approach the transducer is placed at the wrist over the distal wrist acquiring a standard cross-sectional image of the median nerve at this level. The needle is inserted at the ulnar edge of the distal wrist crease, and advanced underneath the transducer at a shallow depth. It is then guided to pass over the ulnar nerve and artery, enters the carpal tunnel, and approximates the ulnar edge of the median nerve. The authors recommend injecting superiorly first, to slightly separate the nerve from the ceiling of the tunnel formed by the transverse carpal ligament.7 The needle can then be withdrawn slightly and re-advanced to a deeper level, ulnar to the nerve, and additional material injected to surround the nerve, from below, with the injectate. This technique has the advantage of keeping the nerve, tendons, ulnar nerve, ulnar artery, and needle tip in sight at all times.
A variety of parenteral steroid preparations and local anesthetics can be used for intralesional therapy of CTS. The author prefers to use a mixture of 1 mL of 1% lidocaine and 1 mL of triamcinolone acetonide 40 mg/mL. Others use 1 mL of 1% lidocaine mixed with 1 mL of methylprednisolone (40 mg).7 Because the mechanism of action is not yet understood, it is difficult to predict how to best determine optimal dose, concentration, and volume of either the steroid compound, or the local anesthetic. The use of ultrasound guidance should enhance the comfort level of physicians performing this technique and possibly lead to the type of quality clinical studies needed to advance the field.
Ultrasound Guidance for Botulinum Toxin Injections
The Physiology and Pharmacology of Botulinum Toxin
The mechanism of action of the botulinum toxins is that they bind to and disable the interior membrane proteins essential for neurotransmitter release in the nerve terminals at the neuromuscular junction.8–10 The explanation for their prolonged duration of effect is still not well understood, a phenomenon all the more remarkable in view of the minuscule doses used in patients that are on the order of nanograms of active toxin. Perhaps the nerve terminal environment, where the toxin is retained, is relatively free of enzymatic processes that degrade the active toxin.
The human extensor digitorum brevis, a largely superfluous muscle for shoe-wearing bipeds, is an ideal muscle for studying the clinical effects of botulinum toxins.11 Injection of 5 units of Botox in this muscle has a profound but slightly delayed effect on muscle function as measured by motor responses evoked by supramaximal stimulation of the peroneal nerve (compound muscle action potentials) or by the mean rectified voltage of surface EMG recorded during maximal voluntary contraction of the extensor digitorum brevis as shown in Figures 11.5 to 11.7. At 100 days, a time when many patients undergo repeat injection for dystonia, the muscle is far from full recovery. In many ways this should not be unexpected. Patients with postoperative surgical pain do not wait for the effects of a single dose of morphine to wear off before asking for the next, so it makes sense that the beneficial effects of the drug should still be present at a time when repeat therapy is requested. Interviews of individuals who recover from accidental botulinum toxin poisoning indicate that they do not experience complete recovery from it for 1 to 2 years.12
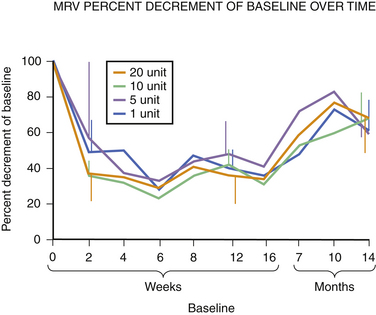
Fig. 11.6 This figure is from the same subjects as in Figure 11.5 and represents the data from full voluntary activation of the extensor digitorum brevis while recording the mean rectified voltage of a 200 ms epoch of data. Again, full recovery has not occurred, this time in any subject, at 14 months. The disparity between the compound muscle action potentials data and the mean rectified voltage data raises the possibility that there may be a central effect of botulinum toxin related to voluntary recruitment; however, the n is too small for definitive conclusions. Claims of longer duration of one botulinum toxin product versus another based on subjective estimates of perceived duration of clinical benefit may need to be tempered in light of more tangible evidence available from quantitative studies of muscle.
Experience in this author’s own laboratory indicates that this may be the case. Following extensor digitorum brevis injections of 1, 5, 10, or 20 units of botulinum toxin, it was found that at 10 months all injected muscles averaged only 70% recovery; even at 14 months, those injected with 5, 10, or 20 units of botulinum toxin were still only 70% of baseline. Only those injected with 1 unit were completely recovered at that point (see Fig. 11.5).
In addition to measurements of electrical activity, the effects of botulinum toxin can also be assessed by loss of muscle bulk. Hamjian and Walker11 showed that Botox injections into the extensor digitorum brevis caused a significant and measurable loss of thickness of this muscle by ultrasound imaging that peaked at 42 days after injections of botulinum toxin. This observation has two substantive implications. The first relates to the fact that muscle hypertrophy contributes to the severity of the symptoms of dystonia. Prior to the advent of botulinum toxin in patients whose symptoms went untreated for years it was common to find that patients complained not only of visible hypertrophy of the sternocleidomastoid muscle (the only muscle with sufficiently distinct contours to be so described), but also of enlargement of their neck size. Women noted that they needed to add links to their necklaces, and men noted that their collar size increased. One of the consequences of constant pulling of the neck muscles was that they became increasingly stronger with the exercise afforded by the involuntary muscle contractions, adding to the disability component of the disorder. Botulinum toxin reverses the hypertrophy, and in fact with repeated injections results in palpable and visible atrophy.13 Anecdotally, some have suggested that not all botulinum toxins cause the same degree of atrophy following injection, speculation that has yet to be validated by clinical data. Ultrasound would be a tool that could easily address this question if clinical observation warranted it. The other implication of this observation is that ultrasound would likely be an effective tool for measuring the efficacy of drugs that reversed atrophy, an observation of potential relevance for disorders such as neuropathy or motor neuron disease where atrophy is a fundamental manifestation of illness.
Guiding Botulinum Toxin Injections with Ultrasound
The pioneers of botulinum toxin therapy in the 1980s for neurologic indications in the United States used palpation and surface landmarks for guiding toxin injections. Subsequently, EMG guidance evolved, using Teflon-coated hypodermic recording needles that were insulated with only a bare tip exposed. Blind injections were generally effective, particularly if administered by experienced clinicians, but subsequent studies showed that EMG needle guidance enhanced the effects of such injections with fewer side effects.14