CHAPTER 101 Instability: Clinical Manifestations and Assessment
INTRODUCTION
Lumbar segmental instability is an important but often unrecognized cause of chronic low back pain (LBP). It has been a controversial and poorly understood topic, primarily because of the varying definitions and usage among the several disciplines involved in the treatment of spinal disorders.1
Traditionally, one of the most obvious manifestations of lumbar instability has been the radiographic diagnosis of spondylolisthesis,2,3 with increased segmental motion reported in patients with chronic LBP.4–7 However, lumbar segmental instability (‘microinstability’) also has been cited as a cause of chronic LBP in patients without an injury to the osseous spine,8 with a number of studies reporting increased and abnormal intersegmental motion in these patients.9–11
DEFINITION OF SPINAL INSTABILITY
Numerous biomechanical and clinical definitions have been developed to describe spinal instability; however, most, including the one adopted by the American Academy of Orthopaedic Surgeons, imply excessive motion beyond normal constraints.12–16 Despite a multitude of biomechanical, radiographic, and clinical studies, ‘normal’ motion of the lumbar vertebral segments continues to be a topic of debate because of the lack of standardized measurements in normal subjects.17
Correlating clinical instability and radiographic instability also has been difficult because of the overlap of symptomatic and asymptomatic motion patterns. Additionally, conventional radiography often is insensitive and unreliable in detecting abnormal or excessive intersegmental motion.18,19 Conceptually, therefore, as with other spinal conditions, radiographic abnormalities (more specifically, abnormal motion of a single motion segment) are considered significant only if they confirm the clinical finding (of lumbar segmental instability) at the corresponding symptomatic level.14
As the pathomechanics of the lumbar spine have become better understood, definitions of spinal instability have been developed that incorporate both the mechanical aspect and the clinical consequence. In 1992, Panjabi defined spinal instability as a region of laxity around the neutral position of a spinal segment, called the neutral zone, and more precisely as a decrease in the capacity of the stabilizing systems of the spine to maintain intervertebral neutral zones within physiological limits within which there is no major deformity, no neurological deficit, and no incapacitating pain.20 This definition is useful because it describes the quality of motion throughout the range of motion rather than relying solely on the total range of motion values for diagnosis.21
THE NEUTRAL ZONE CONCEPT AND THE SPINAL STABILIZING SYSTEM
The neutral zone concept of segmental instability is based on the observation that the load-displacement curve of the spine is nonlinear (i.e. the ratio of the load applied to the displacement produced is not consistent), with minimal resistance to intervertebral motion occurring within the neutral zone and increased resistance to intervertebral motion occurring at the end-ranges of spinal motion.22 This concept has been described as a ‘ball in a bowl’ (Fig. 101.1). The ball rests in a bowl created by flipping the extension part of the load-displacement curve around the displacement axis. The ball moves easily within the base of the bowl (the neutral zone), but requires greater effort to move in the steeper part of the bowl (the end-ranges of motion). The shape of the bowl is analogous to spinal stability: a deeper bowl, such as a wine glass, represents a more stable spine, while a shallower bowl, such as a soup plate, represents a less stable spine (Fig. 101.2).22 The total range of motion of a spinal motion segment, therefore, can be divided into a neutral zone and an elastic zone. The neutral zone is the initial spinal motion which is produced against minimal internal resistance, whereas the elastic zone is motion nearer to the end-range of movement that is produced against significant internal resistance.23 In order for the stabilizing system of the spine to be effective, it must limit the excursion of spinal motion within a segment and maintain the proper ratio of neutral to elastic motion.23
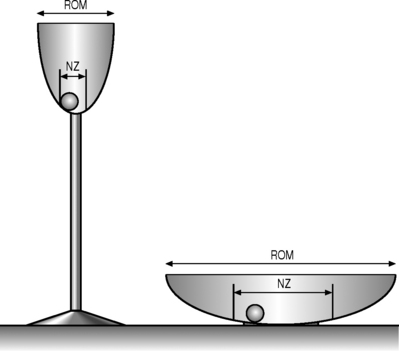
Fig. 101.2 Representation of different spinal stabilities. Using the analogy of a ball in a bowl to represent the load-displacement curve of the spine (Fig. 101.1), a deep champagne glass and a shallow soup plate represent a more and a less stable spine, respectively.
(Adapted from Panjabi MM. Clinical spinal stability and low back pain. J Electromyogr Kinesiol 2003; 13:371–379.)
Biomechanical studies of the stabilizing system of the spine have provided insight into the roles of the various components providing spinal stability. The stabilizing system of the spine is composed of three subsystems: (1) the spinal column or passive subsystem, (2) the spinal muscles or active subsystem, and (3) the neural control unit.20,22 Because, under normal conditions, the three subsystems work in harmony to provide mechanical stability, their functions are interrelated, and decreased function of one subsystem may result in increased demands on the others to maintain stability.24,25 The various components of the spinal column generate transducer information about the mechanical status of the spine, such as position, load, and motion of each vertebra, and the neural control unit computes the needed stability and generates the appropriate muscle pattern (Fig. 101.3).23 A comprehensive knowledge of the three subsystems of the lumbar spine is essential in understanding spinal stabilization and clinically evaluating patients with LBP.
The passive subsystem
The passive subsystem consists primarily of the vertebral bodies, zygapophyseal joints and joint capsules, spinal ligaments, and passive tension from the musculotendinous units.20 The passive subsystem plays its most important stabilizing role in the elastic zone of the spinal motion (i.e. near the end-ranges of motion).25 Serial sectioning26,27 and mathematical modeling25,28 have been used to investigate the relative contributions of structures to segmental stability. Spinal flexion is stabilized primarily by the posterior ligaments of the spine (interspinous and supraspinous ligaments), the zygapophyseal joints and joint capsules, and the intervertebral discs,28,29 while end-range extension is stabilized primarily by the anterior longitudinal ligament, the anterior aspect of the anulus fibrosus, and the zygapophyseal joints.25,27 Rotational movements of the lumbar spine are stabilized mostly by the intervertebral discs and the zygapophyseal joints.30 Although not studied extensively, the intertransverse ligaments appear to play an important role in segmental stability during side-bending movement.26
Because afferent nerve fibers capable of conveying proprioceptive information are present in most of the structures of the passive subsystem, including the intervertebral discs, the zygapophyseal joint capsules, and the interspinous and supraspinous ligaments,24,31 these structures may function as force transducers in the neutral zone, sensing changes in position and providing feedback to the neural control subsystem.20,26,31 Injury to the passive subsystem, such as intervertebral disc degeneration or disruption of the posterior ligaments of the spine, may increase the size of the neutral zone, increasing the demands on the active and neural control subsystems to avoid the development of segmental instability.20,22,32
The active subsystem
The active subsystem of the spinal stabilizing system consists of the spinal muscles and tendons. The active and neural control subsystems are primarily responsible for spinal stability in the neutral zone, where passive resistance to movement is minimal.20,27 Without musculotendinous support, the lumbar spine is highly unstable at very low applied loads.33 The relative contributions of different muscle groups to lumbar spine stability have not been clearly delineated, despite much research.34–38
The contributions of the deeper, unisegmental muscles differ from those of the more superficial multisegmental muscles, such as the abdominal and erector spinae muscles.34 Because of their small size, their close proximity to the center of rotation for spinal movements, and their high concentration of muscle spindles,39,40 the unisegmental muscles of the lumbar spine, such as the intertransversarii and the interspinalis muscles, are believed to function primarily as force transducers, providing feedback on spinal position and movements to the neural control subsystem.20
The larger multisegmental muscles are responsible for producing and controlling movements of the lumbar spine, primarily lifting and rotational movements. The lumbar erector spinae muscle group provides most of the extensor force required for most lifting tasks,41 while rotation is produced primarily by the oblique abdominal muscles.35 Both of these muscles have few or no direct attachments to the lumbar spinal motion segments and, therefore, do not exert forces directly on individual motion segments. The multifidus muscle, which originates from the spinous process of the lumbar vertebrae and forms a series of repeating fascicles attaching to the inferior lumbar transverse process, the ilium, and the sacrum, provides segmental control. The multifidus muscle functions as a stabilizer during lifting and rotational movements of the lumbar spine.42 Although not studied extensively, the quadratus lumborum muscle is believed to be the primary active stabilizer during movements in the frontal plane.43
The role of the abdominal muscles in spinal stability has been suggested to be the generation of extensor force during lifting tasks, either by increasing intra-abdominal pressure or by creating tension in the lumbodorsal fascia.36,44 Research indicates, however, that the abdominal muscles are not capable of generating substantial extensor force through these mechanisms.37,42 The abdominal muscles are primarily flexors and rotators of the lumbar spine.45 The oblique abdominal and transverses abdominis muscles, with their more horizontal orientation, are thought to contribute to spinal stability by creating a rigid cylinder around the spine and by increasing the stiffness of the lumbar spine.38,46 Continuous activity of the transverses abdominis muscle has been demonstrated throughout flexion and extension movements of the lumbar spine.47
The neural control subsystem
The neural control subsystem receives input from structures in the passive and active subsystems that allows it to determine the specific requirements for maintaining spinal stability and to act through the spinal musculature to stabilize the spine.20,38,48 Dysfunction of the neural control system may place other spinal structures at risk for injury,20 and the risk of reinjury may be increased if proper functioning of the neural control system is not restored after injury.48
No evidence has linked poor neuromuscular control to an increased risk of initial injury to the lumbar spine, but several studies38,49–52 have shown that patients with LBP often have persistent deficits in neuromuscular control, indicating that recovery of proper function of the neural control subsystem is not automatic following an initial injury. Increased postural sway and slower reaction times have been identified in patients with LBP compared to subjects without LBP.49–51 Luoto et al.50 found in patients undergoing rehabilitation that improvements in reaction time correlated with reduced disability, indicating that neuromuscular control deficits often persist after lumbar spine injury and that reduction in these deficits correlates with improvement in functional status.
The neural control system also may help stabilize the spine in anticipation of an applied load. Hodges and Richardson38,52 reported that transverses abdominis and multifidus muscle activity consistently preceded active extremity movement in subjects without LBP, but in patients with LBP the contraction of the transverses abdominis muscles was delayed, possibly indicating deficient neural control. Further research is needed to clarify the role of neural control in patients with LBP, but these preliminary findings indicate that enhancing neural control may be an important consideration in the prevention and rehabilitation of LBP.
A HYPOTHESIS THAT RELATES MOTION TO PAIN
The relationship between abnormal intervertebral motion and low back pain is inherent in the definition of clinical spinal instability. In theory, therefore, if intervertebral motion is decreased in a patient with LBP, pain also should be decreased. This assumption is the basis for low back treatments involving surgical fusion, muscle strengthening, and muscle control training.23 Based on the hypothesis that severe LBP is caused by instability between lumbar segments, Olerud et al.53 used external fixation to produce ‘instantaneous fusion’ of selected lumbar spinal segments in 18 patients with chronic severe LBP; 17 patients experienced ‘remarkable relief’ of pain. The authors suggested that this test could be used to identify unstable segments before surgery. Panjabi et al.,54 in a biomechanical cadaver study of the cervical spine, found that after stabilization with external fixation the average range of motion decreased by 39.3%, while the neutral zone decreased by an average of 68.8%.
Using the ‘ball in a bowl’ analogy, Panjabi23 formulated a hypothesis to relate motion to pain (Fig. 101.4). He postulated that in people without spinal pain the neutral zone and range of motion are normal and the ball moves freely within the pain-free zone (Fig. 101.4A). Injury to or degenerative changes in a spinal column component result in an increase in the neutral zone and the ball moves freely over a larger distance, beyond the pain-free zone (Fig. 101.4B). The spinal stabilizing system reacts to decrease the neutral zone by activating the muscles or by adaptive stiffening of the spinal column over time (e.g. formation of osteophytes) (Fig. 101.4C). The spine also may be stabilized by surgical fusion, muscle strengthening, and retraining of the neuromuscular control system. In the analogy, the ball is now anchored and the spine is again pain free. This hypothesis is unproven and must be validated by future clinical studies.23
CLINICAL ASSESSMENT OF LUMBAR INSTABILITY
General classification and evaluation
Lumbar segmental instability can be viewed as a purely movement syndrome (Table. 101.1): microinstability (without osseous injury) in which directional patterns of motion produce an observed lack of movement control and related symptoms within the neutral zone or may be associated with other conditions (Table. 101.2),55 which are discussed in other chapters. In general, the principles derived from traumatic conditions also are applicable to infections and tumors, which produce instability by mechanical weakening of the anterior and middle columns of the spine. For the evaluation of acute spinal injuries caused by trauma, tumor, or infection, White and Panjabi developed a checklist (Table. 101.3) based on anatomy, biomechanics, and clinical observations with the goal of establishing reproducible guidelines.56 In chronic degenerative clinical instability, however, the link between objective findings, which usually are radiographic, and clinical symptoms is not clear and can be confused by coexisting degenerative conditions such as stenosis or spondylolisthesis. An ongoing challenge is to develop diagnostic tests that are both sensitive and specific enough to clearly identify segmental instability.
Table 101.1 Directional patterns in syndromes causing microinstability
Flexion pattern |
Extension pattern |
Lateral shift pattern |
Multidirectional pattern |
Table 101.2 Conditions associated with lumbar segmental instability