Chapter 23 Injuries to the Cervical Spine
• The cervical spine is mobile and highly prone to traumatic injury.
• Early immobilization of the cervical spine and its thorough evaluation with imaging allow identification of a cervical injury and minimize further injuries related to the clinical evaluation of patients.
• The clinician should always search for clinical and imaging signs of ligamentous injury.
• Injuries can be classified as bony, ligamentous, or both according to various classification schemes described in the text. The type of injury and its degree of stability dictate the intervention, which may include conservative management with external immobilization or aggressive management, including open or closed reduction, or internal stabilization and fusion.
• Spinal cord injury may present in a delayed fashion. In all cases of incomplete spinal cord injury, early reduction, when appropriate, should be considered.
The cervical spine is the most mobile portion of the spine and the most common site of spinal injuries. The relative traumatic involvement of different spinal levels is shown in Table 23.1. Nearly 12,000 to 14,000 spinal cord injuries occur each year in the United States.1–6 Cervical spine trauma accounts for almost 1.5% of all pediatric trauma admissions.7 Nearly 10,000 patients die each year as a result of an injury to the spinal cord.5,8–11
TABLE 23.1 Incidence of Adult Spine Injuries
Spinal Segment Level of Injury | Incidence |
---|---|
Cervical | 60% |
Thoracic | 8% |
Thoracolumbar | 20% |
Lumbar | 10% |
Sacral | 2% |
The most common mechanisms of injury to the cervical column are motor vehicle accidents, falls, and sports. Teens and young adults ranging in age between 15 and 30 are the most frequently injured population.2,5,12–14 Falls account for 18% to 30% of cervical spine injuries in the younger age group (<8 years) and for 11% in the older age group (>8 years). Sports injuries are more prevalent in the older group (20-28%) and uncommon in the younger group (3%).15,16 Nonaccidental trauma and penetrating injuries are also found in small numbers of very young children and adolescents, respectively.
The cause of spinal injury varies with age, and particularly with gender. Males are three to four times as likely to sustain an injury as females. Fractures are the most common injury pattern in all age groups, although ligamentous disruptions and dislocations are more prevalent in the young. Upper cervical spine injuries (C1-C4) are almost twice as common as lower cervical injuries (C5-C7).1,17–19 Subluxation injuries without fracture and spinal cord injuries without radiographic abnormality (SCIWORA) are less common in adults and are more likely to occur in younger patients.17,18,20
Neurological Injury
As many as 15% of patients who sustain a traumatic spinal injury will sustain a neurological injury as a result.5,14 Injuries to the cervical spine, in particular, result in a much higher incidence of injury to the spinal cord than injuries at any other spinal level. The incidence of spinal cord injury ranges from 2% to nearly 100% of cervical spine injuries, depending on the cervical level involved, with an incidence of 40% to 60% overall (Table 23.2).1,2,5,8–12,21,22 The incidence of spinal cord injury associated with cervical fractures is likely to be underestimated because some patients may die before reaching medical attention. This situation is particularly true of atlanto-occipital dislocations, in which 25% of patients may die as a result of respiratory arrest before evaluation.4,5,14
Injury Level | Incidence of Neurological Deficit |
---|---|
Atlanto-occipital dislocation | Up to 100% |
Atlas | 1-2% |
Axis | 10% |
C3-T1 | 6% |
Unilateral cervical facet dislocation | 60% |
Bilateral cervical facet dislocation | Up to 100% |
The level and mechanism of the spinal injury, the force involved, and the patient’s age and medical status have important influences on the extent of neurological injury following trauma. In particular, conditions such as ankylosing spondylitis or Down syndrome, which have a significant effect on the relative rigidity (or laxity) of the spinal column, may predispose affected individuals to neurological injury.5,14 Furthermore, the status of the patient after spinal injury can influence neurological injury, mainly through the deleterious effect of hypotension or hypoxia.3,5,13,14 The American Spinal Cord Injury Association (ASIA) scale23 is a clinical assessment tool that offers a detailed overview of the extent and type of spinal cord injury in an efficient and easy to apply format (Fig. 23.1).
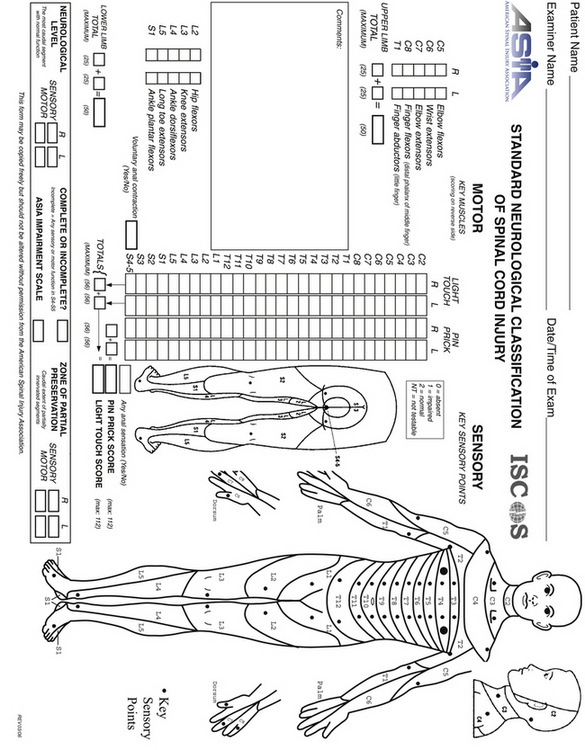
FIGURE 23.1 The American Spine Injury Association (ASIA) scale for spinal cord injury.
(Used with permission from the American Spinal Injury Association.)
General Principles
Given the significant incidence of spinal injury, clinicians must maintain a high index of suspicion for potential spinal injury in all trauma patients. All trauma patients should be immobilized until an appropriate and thorough evaluation of the spine for fractures or instability is completed. Ideally, immobilization should be initiated at the trauma scene and maintained during triage, resuscitation, and primary and secondary surveys. The primary trauma survey places special emphasis on the airway, breathing, and circulation (ABCs of resuscitation). In patients improperly immobilized, the primary survey can exacerbate existing cervical spine injuries in nearly 10% of the cases.4,5
In 2002 the Joint Section on Disorders of the Spine and Peripheral Nerves of the American Association of Neurological Surgeons (AANS) and the Congress of Neurological Surgeons (CNS) published specific evidence-based guidelines for the management of acute cervical spine and spinal cord injuries.24 We have adopted these guidelines and discuss them here.
Imaging
In a trauma situation, the evaluation for cervical injury usually begins with a cross-table lateral radiograph (Fig. 23.2A). A swimmer’s view may be added to visualize the cervicothoracic junction and the top of the T1 vertebral body (Fig. 23.2B).5,14,25 The combination of a cross-table lateral and swimmer’s view has an 85% sensitivity and a negative predictive value of 97% for fracture.25,26 An open-mouth odontoid view should be obtained to assess the C1-C2 vertebrae and articulations and is particularly valuable for assessment of the odontoid process (Fig. 23.2C). If an open-mouth view cannot be obtained for whatever reason, the pillar (oblique) view can be utilized to demonstrate the odontoid process. The anteroposterior view is often ignored by inexperienced clinicians, but it can identify injuries with a rotatory component, such as unilateral facet dislocations, that may not be readily apparent on lateral films. With the use of the anteroposterior, lateral, and open-mouth odontoid views, the sensitivity and negative predictive values of the series are 92% and 99%, respectively.25–27 Adding oblique views to the standard series does not increase the sensitivity.28
The use of computed tomography (CT) has become commonplace in the evaluation of the trauma patient and is particularly useful for evaluating the occipitocervical and the cervicothoracic junctions. However, the routine use of CT in the trauma setting is difficult to recommend as there have been no direct comparisons with plain radiographs.29,30 Areas identified as possible injury on plain radiographs should be further investigated with CT with fine sections through the suspicious area (Fig. 23.3A).14,25 Reconstructions in the sagittal and coronal planes may further help define the nature of the injury (Fig. 23.3B and C).25 Determining the level of trauma may be difficult in SCIWORA patients. In these cases, the neurological examination is critical in evaluating the appropriate level to be studied.17 The evaluation can also be conducted by the use of magnetic resonance imaging (MRI).31 Approximately 10% of patients with a cervical spine fracture have a second associated, noncontiguous vertebral column fracture; therefore, a complete radiographic assessment of the entire spinal column is warranted.5,14 The exceptions to early CT after the initial lateral radiographs have been obtained are patients with an obvious facet fracture-dislocation injury.21 These patients may benefit from early reduction of the fracture and realignment of the cervical spine as long as rostral injuries, especially injuries to the craniocervical junction, are ruled out. Three-dimensional CT can be useful in the evaluation of complex cervical spine fractures and particularly in operative planning (Fig. 23.3D).32
The debate over the most appropriate workup for cervical spine injury in an asymptomatic trauma patient has resulted in several guidelines to minimize the cost and radiation exposure to patients.33 According to the Guidelines from the AANS/CNS Joint Section, trauma patients who meet the following criteria do not require x-ray evaluation of the cervical spine: (1) normal neurological examination and a Glasgow Coma Scale (GCS) score of 15, with no delayed or inappropriate responses, (2) not intoxicated, (3) no neck pain or midline tenderness, and (4) no significant distracting injury such as long-bone fracture or visceral injury.34 Symptomatic patients who do not meet these criteria should undergo a full radiographic assessment of the cervical spine.
Alternatively, the Canadian C-spine rules can be applied as a guideline to minimize the use of radiographs in the evaluation of alert and stable trauma patients when cervical spine injury is a concern.31,35
MRI is an extremely useful tool in the assessment of patients with a cervical spine injury and may identify injuries, such as disk herniations and ligamentous injuries, not seen on plain radiograph or CT. MRI provides a longitudinal assessment of the spine and spinal cord with views in the axial, sagittal, and coronal planes (Fig. 23.4).5,10,14,25,36–38 MRI may be the only imaging modality on which an abnormality is found in patients with SCIWORA, because ligaments, soft tissues, and neural elements can be imaged with superior detail. Hematomas within the spinal canal will not be identified on plain radiograph and may even be missed on CT but are readily identified on MRI.10,37,39 Although MRI is extremely sensitive for ligamentous injury, it cannot be used alone to diagnose an “unstable” injury.35
In the case of cervical trauma but no demonstrable fracture or dislocation injury on the initial standard cervical spine x-ray and CT studies, flexion and extension lateral cervical spine views are important additions to the radiographic assessment. Dynamic flexion-extension views may determine the presence of subluxation injuries or abnormal ligamentous laxity in patients who have persistent post-traumatic neck pain.5,14 In the obtunded or unconscious patient, flexion and extension may be performed under fluoroscopy and may detect a previously unrecognized injury, although its clinical significance is uncertain.40,41 Fluoroscopy is probably indicated only in patients with high-risk injuries such as high-speed motor vehicle accidents, falls over 3 m, major associated injuries, or vehicle crashes involving a death at the scene, because low-risk injuries have only a 0.2% incidence of cervical spine injury.42 MRI can be performed in flexion and extension, a technique that may be useful in identifying positional subluxation or compression of the cervical spinal cord in select cases.25,37,38
Management
Atlanto-Occipital Dislocation
Traumatic atlanto-occipital dislocation (AOD) is associated with a high incidence of neurological morbidity and mortality.39,43–48 The mechanism and amount of force required to disrupt the atlanto-occipital junction are often fatal to victims of this type of injury. Historically, AOD injuries were deemed uncommon. More recent autopsy reports indicate that AOD accounts for 6% to 8% of all traffic fatalities. Of cervical spine injury-related deaths, 20% to 30% are the result of AOD.49–51 Given recent advances in the diagnosis and management of AOD, as many as 20% of survivors with this type of injury have a favorable functional outcome if therapy and immobilization are instituted promptly (Fig. 23.5).35,39,43–47
A classification scheme for the types of AODs describes anterior (type I), longitudinal (type II), and posterior (type III) dislocations based on the movement of the occiput with respect to the cervical spine (Fig. 23.6).
On the basis of class III evidence, The Guidelines for the Management of Acute Cervical Spine and Spinal Cord Injuries recommend applying the Harris method to a plain lateral cervical radiograph to recognize AOD. Since the publication of these guidelines, several groups have documented the use of CT as the diagnostic imaging of choice in patients suspected of having AOD.31,52–54 Based on these studies a basion-dens interval (BDI) (with 10 mm as the cutoff) and the occipital condyle-C1 interval (CCI) (>4 mm is abnormal) are the diagnostic tests of choice.53 It should be emphasized that AOD is a highly unstable injury and can be missed on any imaging modality if normal alignment is temporarily restored. MRI can be an important adjunct in the diagnosis of this condition.35
Our treatment paradigm for AOD is based on the recommendations from the largest published series of AOD patients (Table 23.3). Grade I injuries are those with “normal” BDI and CCI (Fig. 23.7) and equivocal MRI findings such as high posterior ligamentous or occipitoatlantal signal and mild to no signal change at the occipitoatlantal joint. In this population, nonoperative management with a halo or collar is adequate. Grade II injuries are defined by a minimum of one abnormal finding on CT-based criteria or grossly abnormal MRI findings in the occipitoatlantal joints, tectorial membrane, or alar or cruciate ligaments. Effective surgical stabilization of these patients requires open reduction and internal fixation (ORIF) from the occiput to the upper cervical spine.39,43–45 Fixation can be achieved with one of several types of instrumentation (Fig. 23.8) and must be supplemented by allograft or autogenic bone to provide long-term stability.
Occipital Condyle Fractures
Occipital condyle fractures (OCFs) are frequently missed on plain radiographs. With almost universal use of CT scans of the occipitocervical junction in the evaluation of trauma patients, these injuries are now being recognized with increased frequency.55 The true prevalence of OCF is unknown, but most authors have concluded that it is probably more common than typically realized.4,5 The reported incidence of OCF ranges from 4% to 19%, with a mean age of presentation of 32.4 years (range, 7 months to 88 years) and a male predilection of 2:1.56
OCFs commonly occur as isolated injuries.28,29,57 The presence of a retropharyngeal hematoma on a lateral cervical radiograph may be the only clue that serious craniovertebral insult has occurred.58–60 Based on CT-based anatomical studies, Anderson and Montesano have classified these fractures (Fig. 23.9).61 Type I fractures (axial load and comminuted fractures) and type II fractures (extension of a skull-base fracture) are usually considered stable if isolated. Thus, symptomatic treatment is indicated and may include external immobilization in a collar.62 Type III injuries (avulsion of a condylar fragment by the alar ligament) may be unstable and require rigid external immobilization in a collar or halo or even ORIF, if other injuries such as atlantoaxial instability are present.62 Patients not treated may develop lower cranial nerve palsies, which still have a significant chance of resolving with immobilization.63
The need for surgical intervention to treat OCF is rare, and conservative management of all isolated OCFs is generally supported, even in cases of brainstem compression with neurological injury. Bilateral OCF can represent a unique presentation of AOD and needs to be evaluated carefully.35
Atlas Fractures
The atlas is the first cervical vertebra (C1) and bridges the occiput with the axis, the second cervical vertebra (C2), and the rest of the cervical spine.64 The position of the atlas and its ring shape make it vulnerable to a number of different fracture patterns. Fractures of the atlas account for 3% to 13% of all cervical spinal injuries.65,66 Injuries to C1 are mostly isolated, but 40% to 44% are associated with fractures of C2.65 Owing to the wide spinal canal at the level of C1, a neurological injury in the region is rare. Atlas fractures can be observed in various patterns and multiple classification schemes exist. The one used most commonly is the classification first presented by Jefferson67 and later modified by Gehweiler and associates68 (Fig. 23.10). In this modified classification scheme, type I fractures involve only the posterior arch. Only the anterior arch is involved in type II fractures. In type III fractures the posterior arch is fractured bilaterally, and there is an associated unilateral or bilateral anterior arch fracture. The classic Jefferson fracture is a type III atlas burst fracture. In this case the lateral masses are displaced laterally. The classic Jefferson fracture is the most frequent fracture pattern of C1, and it is caused by axial loading of the atlas. Type IV fractures involve the lateral mass, and type V fractures are transverse anterior arch fractures. Congenital abnormalities of the C1 ring can be mistaken for a fracture in some cases and MRI can be used in these cases to assess the affected area for edema within the bone.
Apart from the bony fractures of C1, the integrity of the transverse atlantal ligament (TAL), which is a key factor in determining the stability of atlas fractures, should always be assessed.69 The classic rule of Spence assesses the extent of lateral mass displacement (of C1 with respect to C2) on spine radiographs to provide evidence for the appropriate treatment modality.70 The combined sum of the displacement of both lateral masses of C1 on C2 is measured on open-mouth odontoid or coronal CT. If the combined sum of the displacement is 6.9 mm or greater, it is presumed that the TAL is incompetent and the fracture is considered unstable. In the era of CT and MRI, Dickman and co-workers demonstrated that 61% of TAL ruptures could be missed by using the rule of Spence; therefore, they proposed a new classification system for TAL ruptures (Fig. 23.11).69 Disruption at the midportion of the TAL or at the insertion of the medial tubercle is considered a Dickman type I disruption, which cannot be healed by external immobilization alone. Dickman type II TAL disruption involves purely bony avulsions and can potentially be treated by external immobilization alone.
The treatment of atlas fractures relies only on level III evidence data from published series (Fig. 23.12). In general, isolated fractures can be treated with cervical immobilization alone if the transverse ligament is intact. If the transverse ligament is disrupted, both cervical immobilization alone or surgical fixation and fusion are reasonable options.64 Fractures of the anterior or posterior arch are treated with a rigid cervical orthosis. Fractures of the anterior and posterior arches (burst) with an intact TAL can be treated with a rigid cervical orthosis or halo brace. Both anterior and posterior arch fractures (burst) that are unstable (TAL disrupted) can be treated with a halo brace but usually require C1-C2 stabilization and fusion. Depending upon the extent of injury to C1, occipitocervical fixation may be indicated. Comminuted lateral mass fractures require a rigid cervical orthosis or a halo brace. In all cases, 8 to 12 weeks of immobilization are recommended. When a surgical solution is indicated, the approach is determined by the extent of C1 injury. An unstable unilateral ring or anterior C1 ring fracture requires C1-C2 fusion, while multiple ring or posterior ring fractures may require occipitocervical fusion.
Axis Fractures
Fractures of C2 account for 18% of all cervical spine traumatic injuries.4,12,14,19,21,71,72 Odontoid process fractures are the most common C2 fracture, representing approximately 60% of fractures at this level. The rates of neurological deficit and acute injury mortality associated with axis fractures are 8.5% and 2.4%, respectively.73 Autopsy studies have revealed that a significant proportion of deaths at the scene of traffic accidents are associated with fractures of the upper cervical spine; of these, axis fractures are estimated to range from 25% to 71%.50,74–76
The Anderson-D’Alonzo classification of odontoid fractures is presented in Table 23.4 and Figure 23.13.77 Type I odontoid fracture is the rarest and involves an isolated odontoid tip fracture. Type II is the most common and involves the base of the odontoid tip. The type IIA fracture is the only widely accepted modification to the Anderson-D’Alonzo nomenclature and includes comminution associated with a type II fracture; this type accounts for about 5% of odontoid fractures. Type III odontoid fractures represent one third of all odontoid injuries and involve a fracture that extends through the vertebral body of C2.4,12,14 Odontoid type I and type III fractures are usually treated with rigid external immobilization. Type III fractures have a 97% fusion rate in a halo vest.4,12,14,71,72 In contrast, odontoid type II fractures, which are more difficult to treat, are associated with a nonunion rate as high as 40% when treated with external immobilization alone.4,12,14,19,72 Type II fractures with less than 5 mm of dens displacement are good candidates for halo stabilization. However, the rate of failure associated with external immobilization alone of those with more than 5 mm of dens displacement is greater than 86%; therefore, these fractures should be addressed surgically.4,12,14,72,78 Compared with younger patients, patients older than 50 years of age have a 21-fold greater incidence of nonunion with type II odontoid fractures; thus, primary treatment with surgery should be considered.79 Type IIA odontoid fractures should also be treated with early surgery. Surgical operations include anterior odontoid screw fixation or posterior atlantoaxial fusion.14,80
TABLE 23.4 Anderson and D’Alonzo Classification of Odontoid Fractures
Type | Fracture Location |
---|---|
I | Tip of odontoid |
II | Base of odontoid |
IIA∗ | Type II with bony chips |
III | Base of odontoid into body of C2 |
∗ Hadley and associates added type IIA in 1988: Hadley MN, Browner CM, Liu SS, Sonntag VK. New subtype of acute odontoid fractures (type IIA). Neurosurgery 1988;22(1 Pt 1):67-71.
Hangman’s fractures, or bilateral fractures of the pars interarticularis (also referred to as traumatic spondylolisthesis of the axis), represent 4% of all cervical spine fractures and 20% of all axis fracture injuries.4,12,14,19,81 Hangman’s fractures are associated with a low rate of neurological injury.82 Effendi and colleagues classified hangman’s fractures into nondisplaced fractures (type I), fractures in which the anterior fragment is displaced (type II), and fractures in which the anterior fragment is in a flexed position and a C2-C3 facet dislocation (type III) is present (Fig. 23.14).83 Types I and II hangman’s fractures are well treated with external immobilization with a halo vest or rigid collar.4,12,14,84 Type III hangman’s fractures should be preferentially treated with open surgery.85–87 Levine and Edwards further modified the Effendi classification to include the degree of angulation into the grading system.88 The combination of the Effendi and Levine systems is the most popular system for grading hangman’s injuries. If there is evidence of C2-C3 subluxation and instability with any subtype of axis fracture, surgical repair should be performed because these fractures have a high rate of nonunion with nonoperative therapy.4,12
Fractures involving the body, pedicle, lateral mass, laminae, and spinous process of C2 account for nearly 20% of fractures at this level.14,71,84 Fractures of the C2 vertebral body are usually treated conservatively.89,90
Combination fractures of the atlas and axis comprise 4% of all cervical spine fractures. In general, the type of C2 fracture dictates the type of intervention in combined fracture situations. Surgical options include posterior C1-C2 fusion,91 transarticular screws,92 anterior odontoid screw fixation,93 C1-C3 posterior fusion, or occipitocervical fusion (Fig. 23.15).94,95 Anterior approaches to C1 and C2 include odontoid screw fixation (Fig. 23.16) for odontoid fractures and C2-C3 interbody fusion with anterior plating for hangman’s fractures.4,96,97
C3-T1 Injuries
The third cervical vertebra is an uncommon site for isolated injury, accounting for less than 1% of all cervical spine injuries.4 Fractures of C3 associated with C2 fractures (usually of the hangman’s variety) are slightly more common and may involve the lamina and spinous process of C3. C3 may be relatively protected from injury because it is situated between the more vulnerable C1-C2 complex and C5-C6, which is the area of greatest flexion and extension in the cervical spine.4,71
Almost 75% of all cervical spine fractures occur between C4 and T1.1,4 The most common level of cervical vertebral fracture is C5, and the most common level of subluxation injury is the C5-C6 interspace.1,4,5 Fractures of the vertebral bodies are the most common type of injury to occur in the subaxial cervical spine. In decreasing order of frequency, subluxations, facet dislocations, and laminar, pedicular, or spinous process fractures can also occur. It is possible to have a purely ligamentous injury without fracture or dislocation. Subluxation associated with vertebral body fracture results in a high incidence of spinal cord injury.5,9,11,21 Patients suffering bilateral facet dislocations have a nearly 100% incidence of neurological injury, and the incidence in those with unilateral facet dislocations is 80%.21 Of particular importance, most injuries with unilateral dislocations are root injuries, while the most common neurological injury associated with bilateral facet dislocations is a complete spinal cord injury.
In designing the optimal regimen for the management of patients with C4-T1 spinal injuries, it is important to consider the following factors: the mechanism of injury, the type of injury, the extent of the neurological deficit, and the type of material (e.g., hematoma, bone fragments, or disk material) that may be compressing the spinal cord.4,5,14,57,71,98,99 These injuries can be classified according to the system proposed by Allen and co-workers that includes distraction/flexion (including facet dislocation), compression/flexion/vertical compression, extension, and subluxation injuries.100
General management principles of spinal cord injury also apply for subaxial cervical spinal injuries. Specifically, C3-T1 fracture or subluxations should undergo early reduction and realignment as well as operative decompression of injuries with nonreducible compression of the spinal cord, especially for patients with incomplete spinal cord injuries. Patients with undisplaced vertebral body fractures or isolated posterior element fractures will usually heal with external immobilization alone. Facet dislocations that have been successfully reduced can heal with nonoperative immobilization if there is an associated facet fracture rather than a pure ligamentous injury. Patients treated with nonoperative immobilization need to be followed with serial and dynamic imaging to ensure delayed instability has not developed. If the fracture or subluxation cannot be reduced by closed manipulation, open reduction and repair are required, again more urgently if the patient has an incomplete neurological injury. Operative fixation is also indicated for patients who have failed to heal with external immobilization alone. Patients with pure ligamentous injuries should be considered for primary operative repair as the likelihood of healing without surgery is small.
The surgical approach chosen for patients with subaxial spinal injuries must account for the potential need for decompression of the spinal cord or spinal roots as well as the need for stabilization and fusion.4,5,14,57,98,99 In general, anterior pathology is approached anteriorly and posterior pathology is approached posteriorly, although there are exceptions. Posterior reconstructions may include decompression of the spinal cord and nerve roots if needed, with stabilization using a variety of wiring and screw fixation techniques that must be augmented by a bony fusion.4,57,101,102 Ventral stabilization can be performed following corpectomy or diskectomy with an interbody graft and anterior plate (Fig. 23.17).4,99
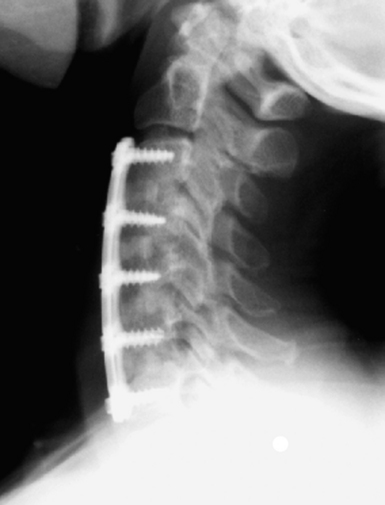
FIGURE 23.17 Lateral postoperative radiograph demonstrating corpectomy and fusion of the cervical spine.
(Used with permission from Barrow Neurological Institute.)
Almost one third of patients with facet-dislocation injuries fail initial closed reduction.21 One third of patients will fail to maintain alignment with external immobilization alone, with pure ligamentous disruption being a predictor of failure.21,103 Anterior and posterior stabilization procedures have a high chance of successful fusion; only 2% to 5% of cases are associated with postoperative instability.104–108 Indications for surgical therapy include nonreducible spinal cord compression with deficit, ligamentous injury with facet instability, kyphosis 15 degrees or greater, vertebral body compression of 40% or more, and subluxation of 20% or more.109
Overall, cervical vertebral compression injuries have a 5% incidence of instability when treated with external immobilization alone.104,110 The incidence of fractures of the extension type associated with instability may be as high as 24%, and that of subluxation-type fractures may be even higher.
Whiplash Injury
The cervical spine is the most mobile segment of the human spine. This property makes it quite vulnerable to injury caused by high- or low-energy forces. Any traumatic injury to the soft tissues of the cervical spine resulting from hyperextension, hyperflexion or rotation of the neck without associated fracture, dislocation, or intervertebral disk herniation is defined as “a whiplash injury.”111 The structures vulnerable to this type of injury are the cervical musculature, ligaments, intervertebral disks, facet joints, and branches of the cervical spinal nerves such as the occipital nerve. Of all traffic accidents 90% occur at speeds less than 14 mph with whiplash injury to the cervical spine being especially common in injuries involving a rear-end collision.112 Interestingly, the clinical picture can be acute with symptoms clearly related to cervical spine injury or can show an insidious, delayed course presenting with cognitive defects, chronic headaches (4.6% of whiplash patients), and even lower back pain.112,113
The severity of whiplash injuries can be approached though the simple clinical grading system proposed by the Quebec Task Force.114 Type I whiplash injuries involve neck pain, stiffness, or tenderness with no clinical signs of cervical spine injury. To the previous group, type II injuries add signs of decreased range of motion (ROM) of the cervical spine with point tenderness. Type III injuries manifest with neck complaints (as in type I injuries), decreased ROM, point tenderness, and neurological signs such as decreased or absent deep tendon reflexes, muscle weakness, and sensory deficits.
The treatment of whiplash injury and associated disorders involves a multimodal approach because many of these patients demonstrate signs of depression or deficits in their ability to work in conjunction with physical signs and symptoms.112,114,115
Cervical Spinal Cord Injuries
The time frame for intervention is critical and the level of care needed is demanding. All acute spinal cord injury patients should be admitted to the intensive care unit. Spinal cord perfusion is precious and must be maintained in the acute setting to preserve function in areas at risk for secondary injuries. Consequently, the mean arterial blood pressure should be maintained at 85 to 90 mm Hg or higher for the first week after injury. Any drop of pressure below that limit should be corrected rapidly with pressors (such as dopamine) or fluids to avoid compromising spinal cord blood flow. Signs of a complete spinal cord injury associated with neurogenic shock indicate a poor prognosis as far as recovery of neurological function is concerned.21 Maintaining oxygenation and meticulous monitoring with clinical evaluations and neuroimaging studies are also critical. The use of high-dose steroids in the setting of spinal cord injury previously was considered to be the standard; however, the utility of the protocol has recently been subjected to serious criticism.116–118 Currently, high-dose steroids are an option (level III evidence) in the management of spinal cord injury and should be given within 8 hours of injury if at all. Many centers have abandoned the use of corticosteroids as a thoughtful review of the literature has seriously questioned their efficacy. Level I evidence supports deep venous thrombosis preventive strategies in patients with a spinal cord injury and should be followed meticulously. This approach includes stockings, compression devices, and anticoagulation.
Some authors have stated that the neurological outcome of a cervical spinal cord injury may be optimized by initial attempts at closed reduction followed by the institution of rigid external immobilization.14,21 Early closed reduction of these injuries with cranial-cervical or manual traction offers the possibility of recovery of neurological function in select patients, particularly those with incomplete neurological injuries.21 There is an 80% success rate with these maneuvers, a 1% chance of permanent neurological injury, and a 2% to 4% chance of transient neurological change.119 Only two well-documented cases have ascribed neurological injury to a herniated disk during reduction maneuvers.120,121 The presence of a herniated disk, which may be found in 46% of cases on prereduction MRIs, may reflect a pseudodisk and does not predict neurological outcome.121,122 The overwhelming majority of compression in the case of bilateral locked facets is due to bone. Thus, obtaining a prereduction MRI does not increase the safety of the procedure and may delay therapy.119
The timing of cervical spinal cord decompression appears to be important with respect to subsequent recovery of neurological function in certain patients, because of focal compressive injury. These patients may benefit from early surgery and stabilization, although no randomized controlled trials have confirmed this possibility.123–125 A recent meta-analysis of the timing of decompressive surgery after traumatic spinal cord injury indicated that early surgical intervention should be considered for every patient with compressive injury within 8 to 24 hours of an acute spinal cord injury.126 Evidence is also available on the safety of early surgery (<72 hours) in patients with spinal cord injury after hemodynamic optimization. Urgent reduction of bilateral locked facets in a patient with incomplete tetraplegia is also indicated.126,127
A small subset of adult patients will have signs of a spinal cord injury but no fracture or subluxation demonstrable on radiograph or CT. This scenario, which is much more common in children than in adults, is referred to as SCIWORA (spinal cord injuries without radiographic abnormality).128 Presentation may be delayed and caution is needed in managing children who initially present with mild neurological dysfunction, even if they may be neurologically normal when examined. Children initially cleared of spinal column injury who have subsequently returned with signs of spinal cord injury have been reported.18,20,128,129 These patients, as in all patients with spinal cord injury, must be properly immobilized and evaluated with CT, MRI, and dynamic x-rays. Some evidence suggests that somatosensory evoked potentials are useful in identifying the extent of injury and in predicting the expected neurological recovery.17,18,20 Poor prognostic indicators include a complete neurological injury and age less than 4 years.17,20
Evidence also suggests that MRI may be able to predict outcome, with a normal scan being predictive of an excellent neurological outcome.130 Based on MRI data five subgroups of patients with SCIWORA can be identified: complete transection, major hemorrhage, minor hemorrhage, edema only, and normal. Appropriate therapy includes rigid immobilization in a collar, restriction from activities that may result in further injury, and follow-up examination that should include flexion and extension views. Current recommendations are for 12 weeks of rigid immobilization followed by another 12 weeks of restricted activity, although achieving these goals in children can be challenging.131 Several investigators have described delayed SCIWORA injuries and recurrent spinal cord injuries among patients who presented with SCIWORA injuries but recovered and were cleared of vertebral column injury on their initial hospital evaluation.
Anderson L.D., D’Alonzo R.T. Fractures of the odontoid process of the axis. J Bone Joint Surg Am. 1974;56(8):1663-1674.
Dickman C.A., Mamourian A., Sonntag V.K., Drayer B.P. Magnetic resonance imaging of the transverse atlantal ligament for the evaluation of atlantoaxial instability. J Neurosurg. 1991;75(2):221-227.
Effendi B., Roy D., Cornish B., et al. Fractures of the ring of the axis. A classification based on the analysis of 131 cases. J Bone Joint Surg Br. 1981;63-B(3):319-327.
Gehweiler J.A.Jr., Clark W.M., Schaaf R.E., et al. Cervical spine trauma: the common combined conditions. Radiology. 1979;130(1):77-86.
Sonntag V., Hadley M. Management of upper cervical spinal instability. In: Wilkins R., editor. Neurosurgery Update. New York: McGraw Hill; 1991:222-223.
Please go to expertconsult.com to view the complete list of references.
1. Bohlman H.H. Acute fractures and dislocations of the cervical spine. An analysis of three hundred hospitalized patients and review of the literature. J Bone Joint Surg Am. 1979;61(8):1119-1142.
2. Bohlman H., Boada E. Fractures and dislocations of the lower cervical spine. In: Cervical Spine Research Society. The Cervical Spine. Philadelphia: Lippincott; 1983:232-267.
3. Heiden J.S., Weiss M.H., Rosenberg A.W., et al. Management of cervical spinal cord trauma in Southern California. J Neurosurg. 1975;43(6):732-736.
4. Sonntag V.K., Hadley M. Management of nonodontoid upper cervical spine injuries. In: Cooper P., editor. Management of Posttraumatic Spinal Instability: Neurosurgical Topics. Rolling Meadows, IL: AANS Publications; 1990:99-109.
5. Weiss J. Mid- and lower cervical spine injuries. In: Wilkins R., editor. Neurosurgery. New York: McGraw Hill; 1985:1708-1715.
6. Clinical assessment after acute cervical spinal cord injury. Neurosurgery. 2002;50(Suppl 3):S21-S29.
7. Patel J.C., Tepas J.J.III, Mollitt D.L., Pieper P. Pediatric cervical spine injuries: defining the disease. J Pediatr Surg. 2001;36(2):373-376.
8. Harris P., Karmi M.Z., McClemont E., et al. The prognosis of patients sustaining severe cervical spine injury (C2-C7 inclusive). Paraplegia. 1980;18(5):324-330.
9. Heiden J., Weiss M. Cervical spine injuries with and without neurological deficit. I. Contemp Neurosurg. 1980;2:1-6.
10. Mesard L., Carmody A., Mannarino E., Ruge D. Survival after spinal cord trauma. A life table analysis. Arch Neurol. 1978;35(2):78-83.
11. Riggins R.S., Kraus J.F. The risk of neurologic damage with fractures of the vertebrae. J Trauma. 1977;17(2):126-133.
12. Hadley M.N., Dickman C.A., Browner C.M., Sonntag V.K. Acute axis fractures: a review of 229 cases. J Neurosurg. 1989;71(5 Pt 1):642-647.
13. Reiss S.J., Raque G.H.Jr., Shields C.B., Garretson H.D. Cervical spine fractures with major associated trauma. Neurosurgery. 1986;18(3):327-330.
14. Sonntag V., Hadley M. Management of upper cervical spinal instability. In: Wilkins R., editor. Neurosurgery Update. New York: McGraw Hill; 1991:222-223.
15. Brown R.L., Brunn M.A., Garcia V.F. Cervical spine injuries in children: a review of 103 patients treated consecutively at a level 1 pediatric trauma center. J Pediatr Surg. 2001;36(8):1107-1114.
16. Cirak B., Ziegfeld S., Knight V.M., et al. Spinal injuries in children. J Pediatr Surg. 2004;39(4):607-612.
17. Hadley M.N., Zabramski J.M., Browner C.M., et al. Pediatric spinal trauma. Review of 122 cases of spinal cord and vertebral column injuries. J Neurosurg. 1988;68(1):18-24.
18. Ruge J.R., Sinson G.P., McLone D.G., Cerullo L.J. Pediatric spinal injury: the very young. J Neurosurg. 1988;68(1):25-30.
19. Schneider R. High cervical spine injuries. In: Wilkins R., editor. Neurosurgery. New York: McGraw Hill; 1985:1701-1708.
20. Pollack I.F., Pang D., Sclabassi R. Recurrent spinal cord injury without radiographic abnormalities in children. J Neurosurg. 1988;69(2):177-182.
21. Hadley M.N., Fitzpatrick B.C., Sonntag V.K., Browner C.M. Facet fracture-dislocation injuries of the cervical spine. Neurosurgery. 1992;30(5):661-666.
22. Gehweiler J.A.Jr., Clark W.M., Schaaf R.E., et al. Cervical spine trauma: the common combined conditions. Radiology. 1979;130(1):77-86.
23. Chafetz R.S., Vogel L.C., Betz R.R., et al. International standards for neurological classification of spinal cord injury: training effect on accurate classification. J Spinal Cord Med. 2008;31(5):538-542.
24. Joint Section on Disorders of the Spine and Peripheral Nerves of the American Association of Neurological Surgeons (AANS) and the Congress of Neurological Surgeons (CNS). Guidelines for the management of acute cervical spine and spinal cord injuries. Neurosurgery. 2008. 333
25. Cohen W. Imaging and determination of posttraumatic spinal instability. In: Cooper P., editor. Management of Posttraumatic Spinal Instability: Neurosurgical Topics. Rolling Meadows, IL: AANS Publications; 1990:19-35.
26. Ross S.E., Schwab C.W., David E.T., et al. Clearing the cervical spine: initial radiologic evaluation. J Trauma. 1987;27(9):1055-1060.
27. Hoffman J.R., Mower W.R., Wolfson A.B., et al. Validity of a set of clinical criteria to rule out injury to the cervical spine in patients with blunt trauma. National Emergency X-Radiography Utilization Study Group. N Engl J Med. 2000;343(2):94-99.
28. Freemyer B., Knopp R., Piche J., et al. Comparison of five-view and three-view cervical spine series in the evaluation of patients with cervical trauma. Ann Emerg Med. 1989;18(8):818-821.
29. Nunez D., Zuluaga A., Fuentes-Bernardo D., et al. Cervical spine trauma: how much more do we learn by routinely using helical CT? Radiographics. 1996;16:1318-1321.
30. Berne J.D., Velmahos G.C., El-Tawil Q., et al. Value of complete cervical helical computed tomographic scanning in identifying cervical spine injury in the unevaluable blunt trauma patient with multiple injuries: a prospective study. J Trauma. 1999;47(5):896-902.
31. Horn E.M., Feiz-Erfan I., Lekovic G.P., et al. Survivors of occipitoatlantal dislocation injuries: imaging and clinical correlates. J Neurosurg Spine. 2007;6(2):113-120.
32. Hadley M.N., Sonntag V.K., Amos M.R., et al. Three-dimensional computed tomography in the diagnosis of vertebral column pathological conditions. Neurosurgery. 1987;21(2):186-192.
33. Mirvis S.E., Diaconis J.N., Chirico P.A., et al. Protocol-driven radiologic evaluation of suspected cervical spine injury: efficacy study. Radiology. 1989;170(3 Pt 1):831-834.
34. Radiographic assessment of the cervical spine in asymptomatic trauma patients. Neurosurgery. 2002;50(Suppl 3):S30-S35.
35. Stiell I.G., Wells G.A., Vandemheen K.L., et al. The Canadian C-spine rule for radiography in alert and stable trauma patients. JAMA. 2001;286(15):1841-1848.
36. Dickman C.A., Mamourian A., Sonntag V.K., Drayer B.P. Magnetic resonance imaging of the transverse atlantal ligament for the evaluation of atlantoaxial instability. J Neurosurg. 1991;75(2):221-227.
37. Chakeres D.W., Flickinger F., Bresnahan J.C., et al. MR imaging of acute spinal cord trauma. AJNR Am J Neuroradiol. 1987;8(1):5-10.
38. Pech P., Kilgore D.P., Pojunas K.W., Haughton V.M. Cervical spinal fractures: CT detection. Radiology. 1985;157(1):117-120.
39. Dickman C.A., Douglas R., Sonntag V.K. Occipitocervical fusion: posterior stabilization of the craniovertebral junction and upper cervical spine. BNI Quarterly. 1991;7:2-13.
40. Davis J.W., Parks S.N., Detlefs C.L., et al. Clearing the cervical spine in obtunded patients: the use of dynamic fluoroscopy. J Trauma. 1995;39(3):435-438.
41. Ajani A.E., Cooper D.J., Scheinkestel C.D., et al. Optimal assessment of cervical spine trauma in critically ill patients: a prospective evaluation. Anaesth Intensive Care. 1998;26(5):487-491.
42. Hanson J.A., Blackmore C.C., Mann F.A., Wilson A.J. Cervical spine injury: a clinical decision rule to identify high-risk patients for helical CT screening. AJR Am J Roentgenol. 2000;174(3):713-717.
43. Bools J.C., Rose B.S. Traumatic atlantooccipital dislocation: two cases with survival. AJNR Am J Neuroradiol. 1986;7(5):901-904.
44. Menezes A.H., VanGilder J.C., Graf C.J., McDonnell D.E. Craniocervical abnormalities. A comprehensive surgical approach. J Neurosurg. 1980;53(4):444-455.
45. Ransford A.O., Crockard H.A., Pozo J.L., et al. Craniocervical instability treated by contoured loop fixation. J Bone Joint Surg Br. 1986;68(2):173-177.
46. Diagnosis and management of traumatic atlanto-occipital dislocation injuries. Neurosurgery. 2002;50(Suppl 3):S105-S113.
47. Menezes A., Muhonen M. Management of occipito-cervical instability. In: Cooper P., editor. Management of Posttraumatic Spinal Instability: Neurosurgical Topics. Rolling Meadows, IL: AANS Publications; 1990:65-74.
48. Govender S., Vlok G.J., Fisher-Jeffes N., Du Preez C.P. Traumatic dislocation of the atlanto-occipital joint. J Bone Joint Surg Br. 2003;85(6):875-878.
49. Adams V.I. Neck injuries: I. Occipitoatlantal dislocation—a pathologic study of twelve traffic fatalities. J Forensic Sci. 1992;37(2):556-564.
50. Alker G.J.Jr., Oh Y.S., Leslie E.V. High cervical spine and craniocervical junction injuries in fatal traffic accidents: a radiological study. Orthop Clin North Am. 1978;9(4):1003-1010.
51. Bucholz R.W., Burkhead W.Z. The pathological anatomy of fatal atlanto-occipital dislocations. J Bone Joint Surg Am. 1979;61(2):248-250.
52. Pang D., Nemzek W.R., Zovickian J. Atlanto-occipital dislocation: part 1—normal occipital condyle-C1 interval in 89 children. Neurosurgery. 2007;61(3):514-521.
53. Pang D., Nemzek W.R., Zovickian J. Atlanto-occipital dislocation: part 2—the clinical use of (occipital) condyle-C1 interval, comparison with other diagnostic methods, and the manifestation, management, and outcome of atlanto-occipital dislocation in children. Neurosurgery. 2007;61(5):995-1015.
54. Dziurzynski K., Anderson P.A., Bean D.B., et al. A blinded assessment of radiographic criteria for atlanto-occipital dislocation. Spine. 2005;30(12):1427-1432.
55. Blacksin M.F., Lee H.J. Frequency and significance of fractures of the upper cervical spine detected by CT in patients with severe neck trauma. AJR Am J Roentgenol. 1995;165(5):1201-1204.
56. Alcelik I., Manik K.S., Sian P.S., Khoshneviszadeh S.E. Occipital condylar fractures. Review of the literature and case report. J Bone Joint Surg Br. 2006;88(5):665-669.
57. Cooper P.R., Cohen A., Rosiello A., Koslow M. Posterior stabilization of cervical spine fractures and subluxations using plates and screws. Neurosurgery. 1988;23(3):300-306.
58. Link T.M., Schuierer G., Hufendiek A., et al. Substantial head trauma: value of routine CT examination of the cervicocranium. Radiology. 1995;196(3):741-745.
59. Bloom A.I., Neeman Z., Floman Y., et al. Occipital condyle fracture and ligament injury: imaging by CT. Pediatr Radiol. 1996;26(11):786-790.
60. Noble E.R., Smoker W.R. The forgotten condyle: the appearance, morphology, and classification of occipital condyle fractures. AJNR Am J Neuroradiol. 1996;17(3):507-513.
61. Anderson P.A., Montesano P.X. Morphology and treatment of occipital condyle fractures. Spine. 1988;13(7):731-736.
62. Occipital condyle fractures. Neurosurgery. 2002;50(Suppl 3):S114-S119.
63. Legros B., Fournier P., Chiaroni P., et al. Basal fracture of the skull and lower (IX, X, XI, XII) cranial nerves palsy: four case reports including two fractures of the occipital condyle—a literature review. J Trauma. 2000;48(2):342-348.
64. Kakarla U.K., Chang S.W., Theodore N., Sonntag V.K. Atlas fractures. Neurosurgery. 2010;66(Suppl 3):60-67.
65. Hadley M.N., Dickman C.A., Browner C.M., Sonntag V.K. Acute traumatic atlas fractures: management and long term outcome. Neurosurgery. 1988;23(1):31-35.
66. Landells C.D., Van Peteghem P.K. Fractures of the atlas: classification, treatment and morbidity. Spine. 1988;13(5):450-452.
67. Jefferson G. Fractures of the atlas vertebrae: report of four cases and a review of those previously recorded. Br J Surg. 1920;7:407-422.
68. Gehweiler J., Duff D., Salutario M., et al. Fractures of the atlas vertebra. Skeletel Radiol. 1976;23:97-102.
69. Dickman C.A., Greene K.A., Sonntag V.K. Injuries involving the transverse atlantal ligament: classification and treatment guidelines based upon experience with 39 injuries. Neurosurgery. 1996;38(1):44-50.
70. Spence K.F.Jr., Decker S., Sell K.W. Bursting atlantal fracture associated with rupture of the transverse ligament. J Bone Joint Surg Am. 1970;52(3):543-549.
71. Spence K.F.Jr., Decker S., Sell K.W. Bursting atlantal fracture associated with rupture of the transverse ligament. J Bone Joint Surg Am. 1970;52(3):543-549.
72. Clark C., Apuzzo M. The evaluation and management of trauma to the odontoid process. In: Cooper P., editor. Management of Posttraumatic Spinal Instability: Neurosurgical Topics. Rolling Meadows, IL: AANS Publications; 1990:77-94.
73. Greene K.A., Dickman C.A., Marciano F.F., et al. Acute axis fractures. Analysis of management and outcome in 340 consecutive cases. Spine. 1997;22(16):1843-1852.
74. Bucholz R.W., Burkhead W.Z., Graham W., Petty C. Occult cervical spine injuries in fatal traffic accidents. J Trauma. 1979;19(10):768-771.
75. Hadley M.N., Sonntag V.K., Grahm T.W., et al. Axis fractures resulting from motor vehicle accidents. The need for occupant restraints. Spine. 1986;11(9):861-864.
76. Huelke D.F., O’Day J., Mendelsohn R.A. Cervical injuries suffered in automobile crashes. J Neurosurg. 1981;54(3):316-322.
77. Anderson L.D., D’Alonzo R.T. Fractures of the odontoid process of the axis. J Bone Joint Surg Am. 1974;56(8):1663-1674.
78. Greene K.A., Dickman C.A., Marciano F.F., et al. Acute axis fractures. Analysis of management and outcome in 340 consecutive cases. Spine. 1997;22(16):1843-1852.
79. Lennarson P.J., Mostafavi H., Traynelis V.C., Walters B.C. Management of type II dens fractures: a case-control study. Spine. 2000;25(10):1234-1237.
80. Hadley M.N., Browner C.M., Liu S.S., Sonntag V.K. New subtype of acute odontoid fractures (type IIA). Neurosurgery. 1988;22(1 Pt 1):67-71.
81. Ryan M.D., Henderson J.J. The epidemiology of fractures and fracture-dislocations of the cervical spine. Injury. 1992;23(1):38-40.
82. Mollan R.A., Watt P.C. Hangman’s fracture. Injury. 1982;14(3):265-267.
83. Effendi B., Roy D., Cornish B., et al. Fractures of the ring of the axis. A classification based on the analysis of 131 cases. J Bone Joint Surg Br. 1981;63-B(3):319-327.
84. Hadley M., Browner C., Sonntag V.K. Miscellaneous fractures of the second cervical vertebra. BNI Quarterly. 1985;1:34-39.
85. Verheggen R., Jansen J. Hangman’s fracture: arguments in favor of surgical therapy for type II and III according to Edwards and Levine. Surg Neurol. 1998;49(3):253-261.
86. Isolated fractures of the axis in adults. Neurosurgery. 2002;50(Suppl 3):S125-S139.
87. Borne G.M., Bedou G.L., Pinaudeau M. Treatment of pedicular fractures of the axis. A clinical study and screw fixation technique. J Neurosurg. 1984;60(1):88-93.
88. Levine A.M., Edwards C.C. The management of traumatic spondylolisthesis of the axis. J Bone Joint Surg Am. 1985;67(2):217-226.
89. Benzel E.C., Hart B.L., Ball P.A., et al. Fractures of the C-2 vertebral body. J Neurosurg. 1994;81(2):206-212.
90. Fujimura Y., Nishi Y., Kobayashi K. Classification and treatment of axis body fractures. J Orthop Trauma. 1996;10(8):536-540.
91. Coyne T.J., Fehlings M.G., Wallace M.C., et al. C1-C2 posterior cervical fusion: long-term evaluation of results and efficacy. Neurosurgery. 1995;37(4):688-692.
92. Guiot B., Fessler R.G. Complex atlantoaxial fractures. J Neurosurg. 1999;91(Suppl 2):139-143.
93. Montesano P.X., Anderson P.A., Schlehr F., et al. Odontoid fractures treated by anterior odontoid screw fixation. Spine. 1991;16(Suppl 3):S33-S37.
94. Dickman C.A., Hadley M.N., Browner C., Sonntag V.K. Neurosurgical management of acute atlas-axis combination fractures. A review of 25 cases. J Neurosurg. 1989;70(1):45-49.
95. Horn E.M., Hott J.S., Porter R.W., et al. Atlantoaxial stabilization with the use of C1-3 lateral mass screw fixation. Technical note. J Neurosurg Spine. 2006;5(2):172-177.
96. Geisler F.H., Cheng C., Poka A., Brumback R.J. Anterior screw fixation of posteriorly displaced type II odontoid fractures. Neurosurgery. 1989;25(1):30-37.
97. Brant-Zawadzki M., Miller E.M., Federle M.P. CT in the evaluation of spine trauma. AJR Am J Roentgenol. 1981;136(2):369-375.
98. Sonntag V.K., Hadley M.N. Nonoperative management of cervical spine injuries. Clin Neurosurg. 1988;34:630-649.
99. Caspar W., Barbier D.D., Klara P.M. Anterior cervical fusion and Caspar plate stabilization for cervical trauma. Neurosurgery. 1989;25(4):491-502.
100. Allen B.L.Jr., Ferguson R.L., Lehmann T.R., O’Brien R.P. A mechanistic classification of closed, indirect fractures and dislocations of the lower cervical spine. Spine. 1982;7(1):1-27.
101. Roy-Camille R., Saillant G., Mazel C. Internal fixation of the unstable cervical spine by a posterior osteosynthesis with plates and screws. In: Cervical Spine Research Society. Cervical Spine. Philadelphia: JB Lippincott; 1989:390-396.
102. Cherney W., Sonntag V.K., Douglas R. Lateral mass posterior plating and facet fusion for cervical spine instability. BNI Quarterly. 1991;7:2-11.
103. Halliday A.L., Henderson B.R., Hart B.L., Benzel E.C. The management of unilateral lateral mass/facet fractures of the subaxial cervical spine: the use of magnetic resonance imaging to predict instability. Spine. 1997;22(22):2614-2621.
104. Bucholz R.D., Cheung K.C. Halo vest versus spinal fusion for cervical injury: evidence from an outcome study. J Neurosurg. 1989;70(6):884-892.
105. Fehlings M.G., Cooper P.R., Errico T.J. Posterior plates in the management of cervical instability: long-term results in 44 patients. J Neurosurg. 1994;81(3):341-349.
106. Maiman D.J., Barolat G., Larson S.J. Management of bilateral locked facets of the cervical spine. Neurosurgery. 1986;18(5):542-547.
107. Rockswold G.L., Bergman T.A., Ford S.E. Halo immobilization and surgical fusion: relative indications and effectiveness in the treatment of 140 cervical spine injuries. J Trauma. 1990;30(7):893-898.
108. Wolf A., Levi L., Mirvis S., et al. Operative management of bilateral facet dislocation. J Neurosurg. 1991;75(6):883-890.
109. Treatment of subaxial cervical spinal injuries. Neurosurgery. 2002;50(Suppl 3):S156-S165.
110. Lind B., Sihlbom H., Nordwall A. Halo-vest treatment of unstable traumatic cervical spine injuries. Spine. 1988;13(4):425-432.
111. Hirsch S.A., Hirsch P.J., Hiramoto H., Weiss A. Whiplash syndrome. Fact or fiction? Orthop Clin North Am. 1988;19(4):791-795.
112. Bannister G., Amirfeyz R., Kelley S., Gargan M. Whiplash injury. J Bone Joint Surg Br. 2009;91(7):845-850.
113. Obermann M., Nebel K., Riegel A., et al. Incidence and predictors of chronic headache attributed to whiplash injury. Cephalalgia. 2010;30(5):528-534. (Epub 2010 Feb 1)
114. Spitzer W.O., Skovron M.L., Salmi L.R., et al. Scientific monograph of the Quebec Task Force on Whiplash-Associated Disorders: redefining “whiplash” and its management. Spine. 1995;20(Suppl 8):1S-73S.
115. Pato U., Di S.G., Fravi N., et al. Comparison of randomized treatments for late whiplash. Neurology. 2010;74(15):1223-1230.
116. Pharmacological therapy after acute cervical spinal cord injury. Neurosurgery. 2002;50(Suppl 3):S63-S72.
117. Hurlbert R.J. Methylprednisolone for acute spinal cord injury: an inappropriate standard of care. J Neurosurg. 2000;93(Suppl 1):1-7.
118. Hugenholtz H., Cass D.E., Dvorak M.F., et al. High-dose methylprednisolone for acute closed spinal cord injury—only a treatment option. Can J Neurol Sci. 2002;29(3):227-235.
119. Initial closed reduction of cervical spine fracture-dislocation injuries. Neurosurgery. 2002;50(Suppl 3):S44-S50.
120. Olerud C., Jonsson H.Jr. Compression of the cervical spine cord after reduction of fracture dislocations. Report of 2 cases. Acta Orthop Scand. 1991;62(6):599-601.
121. Grant G.A., Mirza S.K., Chapman J.R., et al. Risk of early closed reduction in cervical spine subluxation injuries. J Neurosurg. 1999;90(Suppl 1):13-18.
122. Vaccaro A.R., Falatyn S.P., Flanders A.E., et al. Magnetic resonance evaluation of the intervertebral disc, spinal ligaments, and spinal cord before and after closed traction reduction of cervical spine dislocations. Spine. 1999;24(12):1210-1217.
123. Dai L., Jia L. Central cord injury complicating acute cervical disc herniation in trauma. Spine. 2000;25(3):331-335.
124. Chen T.Y., Dickman C.A., Eleraky M., Sonntag V.K. The role of decompression for acute incomplete cervical spinal cord injury in cervical spondylosis. Spine. 1998;23(22):2398-2403.
125. Chen T.Y., Lee S.T., Lui T.N., et al. Efficacy of surgical treatment in traumatic central cord syndrome. Surg Neurol. 1997;48(5):435-440.
126. Furlan J.C., Noonan V., Cadotte D.W., Fehlings M.G. Timing of decompressive surgery of spinal cord after traumatic spinal cord injury: an evidence-based examination of pre-clinical and clinical studies. J Neurotrauma. 2011;28(8):1371-1399. (Epub 2010 March 4)
127. Fehlings M.G., Arvin B. The timing of surgery in patients with central spinal cord injury. J Neurosurg Spine. 2009;10(1):1-2.
128. Pang D., Wilberger J.E.Jr. Spinal cord injury without radiographic abnormalities in children. J Neurosurg. 1982;57(1):114-129.
129. Hamilton M.G., Myles S.T. Pediatric spinal injury: review of 174 hospital admissions. J Neurosurg. 1992;77(5):700-704.
130. Grabb P.A., Pang D. Magnetic resonance imaging in the evaluation of spinal cord injury without radiographic abnormality in children. Neurosurgery. 1994;35(3):406-414.
131. Pang D., Pollack I.F. Spinal cord injury without radiographic abnormality in children—the SCIWORA syndrome. J Trauma. 1989;29(5):654-664.