38 Infective meningitis
The brain and spinal cord are surrounded by three membranes, which from the outside inwards are the dura mater, the arachnoid mater and the pia mater. Between the arachnoid mater and the pia mater, in the subarachnoid space, is found the cerebrospinal fluid (CSF) (Fig. 38.1). This fluid, of which there is ∼︀150 mL–1 in a normal individual, is secreted by the choroid plexuses and vascular structures which are in the third, fourth and lateral ventricles. CSF passes from the ventricles via communicating apertures to the subarachnoid space, after which it flows over the surface of the brain and the spinal cord (see Fig. 38.1). The amount of CSF is controlled by resorption into the bloodstream by vascular structures in the subarachnoid space, called the arachnoid villi. Infective meningitis is an inflammation of the arachnoid and pia mater associated with the presence of bacteria, viruses, fungi or protozoa in the CSF. Meningitis is one of the most emotive of infectious diseases, and for good reason: even today, infective meningitis is associated with significant mortality and risk of serious sequelae in survivors.
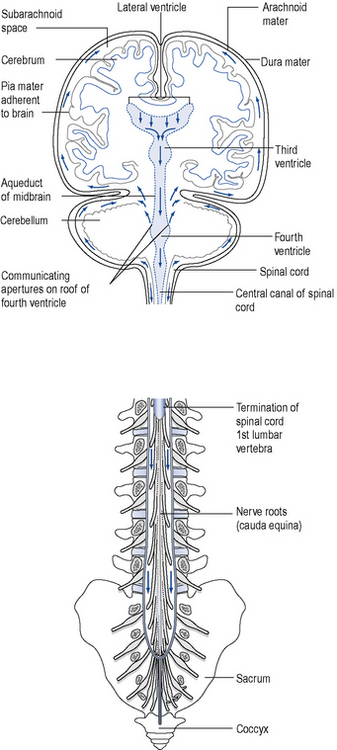
Fig. 38.1 The meninges covering the brain and spinal cord and the flow of cerebrospinal fluid (arrowed)
(modified from Ross and Wilson (1981), by permission of Churchill Livingstone).
Pathophysiology
Diagnosis
In health, the CSF is a clear colourless fluid which, in the lumbar region of the spinal cord, is at a pressure of 50–150 mmH2O. There may be up to 5 cells/μL, the protein concentration is up to 0.4 g/L and the glucose concentration is at least 60% of the blood glucose (usually 2.2–4.4 mmol/L). Table 38.1 shows how the cell count and biochemical measurements can be helpful in determining the type of organism causing meningitis.
Drug treatment
Antimicrobial therapy
Pharmacokinetic considerations
The antimicrobial therapy of meningitis requires attainment of adequate levels of bactericidal agents within the CSF. The principal route of entry of antibiotics into CSF is by the choroid plexus; an alternative route is via the capillaries of the central nervous system into the extracellular fluid and hence into the ventricles and subarachnoid space (see Fig. 38.1). The passage of antibiotics into CSF is dependent on the degree of meningeal inflammation and integrity of the blood–brain barrier created by capillary endothelial cells, as well as the following properties of the antibiotic:
Antimicrobials fall into three categories according to their ability to penetrate the CSF:
Recommended regimens
Antibiotics for meningitis in neonates and infants aged below 3 months
The most important pathogens in neonates include group B streptococci, E. coli and other Enterobacteriaceae, L. monocytogenes. In many centres, a third-generation cephalosporin such as cefotaxime or ceftazidime, along with amoxicillin or ampicillin, is the empiric therapy of choice for neonatal meningitis (Galiza and Heath, 2009). Cephalosporins penetrate into CSF better than aminoglycosides, and their use in Gram-negative bacillary meningitis has contributed to a reduction in mortality to less than 10%. Other centres continue to use an aminoglycoside, such as gentamicin, together with benzylpenicillin, ampicillin or amoxicillin as empiric therapy. This approach remains appropriate, especially in countries such as the UK where group B streptococci are by far the predominant cause of early-onset neonatal meningitis. Whichever empiric regimen is used, therapy can be altered as appropriate once the pathogen has been identified. Suitable dosages are shown in Table 38.2.
Table 38.2 Suitable antibiotic regimens for treatment of acute bacterial meningitis in different age groups
Age group |
---|