Chapter 103 Infections of the Neonatal Infant
103.1 Pathogenesis and Epidemiology
Bizzarro MJ, Dembry LM, Baltimore RS, et al. Changing patterns in neonatal Escherichia coli sepsis and ampicillin resistance in the era of intrapartum antibiotic prophylaxis. Pediatrics. 2008;121:689-696.
Bizzarro MJ, Raskind C, Baltimore RS, et al. Seventy-five years of neonatal sepsis at Yale: 1928–2003. Pediatrics. 2005;116:595-602.
Corey L, Wald A. Maternal and neonatal herpes simplex virus infections. N Engl J Med. 2009;361:1376-1384.
Darville T. Chlamydia trachomatis infections in neonates and young children. Semin Pediatr Infect Dis. 2005;16:235-244.
Vergnano S, Sharland M, Kasembe P, et al. Neonatal sepsis: an international perspective. Arch Dis Child Fetal Neonatal Ed. 2005;90:F220-F224.
Zaidi AKM, Thaver D, Asas S, Khan TA. Pathogens associated with sepsis in newborns and young infants in developing countries. Pediatr Infect Dis J. 2009;28:S10-S18.
103.2 Modes of Transmission and Pathogenesis
Pathogenesis of Intrauterine Infection
Intrauterine infection is a result of clinical or subclinical maternal infection with a variety of agents (cytomegalovirus [CMV], Treponema pallidum, Toxoplasma gondii, rubella virus, varicella virus, parvovirus B19) and hematogenous transplacental transmission to the fetus. Transplacental infection may occur at any time during gestation, and signs and symptoms may be present at birth or may be delayed for months or years (Fig. 103-1). Infection may result in early spontaneous abortion, congenital malformation, intrauterine growth restriction, premature birth, stillbirth, acute or delayed disease in the neonatal period, or asymptomatic persistent infection with sequelae later in life. In some cases, no apparent effects are seen in the newborn infant.
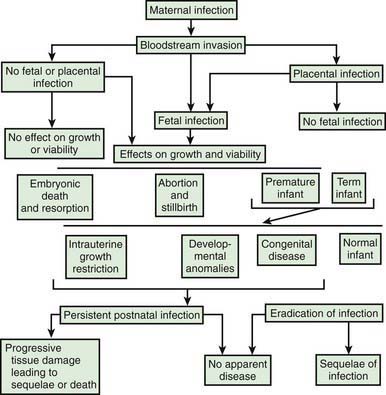
Figure 103-1 Pathogenesis of hematogenous transplacental infections.
(From Klein JO, Remington JS: Current concepts of infections of the fetus and newborn infant. In Remington JS, Klein JO, editors: Infectious diseases of the fetus and newborn infant, ed 5, Philadelphia, 2002, WB Saunders.)
The timing of infection during gestation affects the outcome. First-trimester infection may alter embryogenesis, with resulting congenital malformations (congenital rubella) (Chapter 239). Third-trimester infection often results in active infection at the time of delivery (toxoplasmosis, syphilis) (Chapters 282 and 210). Infections that occur late in gestation may lead to a delay in clinical manifestations until some time after birth (syphilis).
Maternal infection is a necessary prerequisite for transplacental infection. For some etiologic agents (rubella), maternal immunity is effective and antibody is protective for the fetus. For other agents (CMV), maternal antibody may ameliorate the outcome of infection or may have no effect (Chapter 247). Even without maternal antibody, transplacental transmission of infection to a fetus is variable because the placenta may function as an effective barrier.
Pathogenesis of Ascending Bacterial Infection
In most cases, the fetus or neonate is not exposed to potentially pathogenic bacteria until the membranes rupture and the infant passes through the birth canal and/or enters the extrauterine environment. The human birth canal is colonized with aerobic and anaerobic organisms that may result in ascending amniotic infection and/or colonization of the neonate at birth. Vertical transmission of bacterial agents that infect the amniotic fluid and/or vaginal canal may occur in utero or, more commonly, during labor and/or delivery (Fig. 103-2). Chorioamnionitis results from microbial invasion of amniotic fluid, often as a result of prolonged rupture of the chorioamniotic membrane. Amniotic infection may also occur with apparently intact membranes or with a relatively brief duration of membrane rupture. The term chorioamnionitis refers to the clinical syndrome of intrauterine infection, which includes maternal fever, with or without local or systemic signs of chorioamnionitis (uterine tenderness, foul-smelling vaginal discharge/amniotic fluid, maternal leukocytosis, maternal and/or fetal tachycardia). Chorioamnionitis may also be asymptomatic, diagnosed only by amniotic fluid analysis or pathologic examination of the placenta. The rate of histologic chorioamnionitis is inversely related to gestational age at birth (Fig. 103-3) and directly related to duration of membrane rupture. Rupture of membranes for longer than 24 hr was once considered prolonged because microscopic evidence of inflammation of the membranes is uniformly present when the duration of rupture exceeds 24 hr. At 18 hr of membrane rupture, however, the incidence of early-onset disease with group B streptococcus (GBS) increases significantly; 18 hr is the appropriate cutoff for increased risk of neonatal infection.
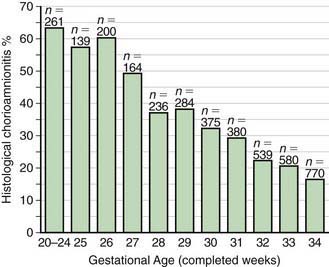
Figure 103-3 Histologic chorioamnionitis in liveborn preterm babies by gestational age (n = 3,928 babies).
(From Lahra MM, Jeffery HE: A fetal response to chorioamnionitis is associated with early survival after preterm birth, Am J Obstet Gynecol 190:147–151, 2004.)
Bacterial colonization does not always result in disease. Factors influencing which colonized infant will experience disease are not well understood but include prematurity, underlying illness, invasive procedures, inoculum size, virulence of the infecting organism, genetic predisposition, the innate immune system, host response, and transplacental maternal antibodies (Fig. 103-4). Aspiration or ingestion of bacteria in amniotic fluid may lead to congenital pneumonia or systemic infection, with manifestations becoming apparent before delivery (fetal distress, tachycardia), at delivery (failure to breathe, respiratory distress, shock), or after a latent period of a few hours (respiratory distress, shock). Aspiration or ingestion of bacteria during the birth process may lead to infection after an interval of 1-2 days.
Mylonakis E, Paliou M, Hohmann EL, et al. Listeriosis during pregnancy. Medicine (Baltimore). 2002;81:260-269.
Nassetta L, Kimberlin D, Whitley R. Treatment of congenital cytomegalovirus infection: implications for future therapeutic strategies. J Antimicrob Chemother. 2009;63:862-867.
Phonesamart W, Yoksan S, Vanaprapa N, et al. Dengue virus infection in late pregnancy and transmission to the infants. Pediatr Infect Dis J. 2008;27:500-504.
Ramful D, Carbonnier M, Pasquet M, et al. Mother-to-child transmission of Chikungunya virus infection. Pediatr Infect Dis J. 2007;26:811-815.
Read JS, Cannom MJ, Stanberry LR, et al. Prevention of mother-to-child transmission of viral infections. Curr Prob Pediatr Adolesc Health Care. 2008;38:269-302.
103.3 Immunity
Natural Killer Cells
Natural killer (NK) cells are a subgroup of lymphocytes that are cytolytic against cells infected with viruses. NK cells also lyse cells coated with antibody in a process called antibody-dependent cell-mediated cytotoxicity (ADCC). NK cells appear early in gestation and are present in cord blood in numbers equivalent to those in adults; neonatal NK cells have decreased cytotoxic activity and ADCC in comparison with adult cells. The diminished cytotoxicity against herpes simplex virus (HSV)–infected cells may predispose to disseminated HSV infection in newborns (Chapter 249).
103.4 Etiology of Fetal and Neonatal Infection
A number of agents may infect newborns in utero, intrapartum, or postpartum (Tables 103-1 and 103-2). Intrauterine transplacental infections of significance to the fetus and/or newborn include syphilis, rubella, CMV, toxoplasmosis, parvovirus B19, and varicella. Although HSV, HIV, hepatitis B virus (HBV), hepatitis C virus, and tuberculosis (TB) can each result in transplacental infection, the most common mode of transmission for these agents is intrapartum, during labor and delivery with passage through an infected birth canal (HIV, HSV, HBV), or postpartum, from contact with an infected mother or caretaker (TB) or with infected breast milk (HIV).
Congenital pneumonia may be caused by CMV, rubella virus, and T. pallidum and, less commonly, by the other agents producing transplacental infection (Table 103-3). Microorganisms causing pneumonia acquired during labor and delivery include GBS, gram-negative enteric aerobes, Listeria monocytogenes, genital Mycoplasma, Chlamydia trachomatis, CMV, HSV, and Candida species.
Table 103-3 ETIOLOGIC AGENTS OF NEONATAL PNEUMONIA ACCORDING TO TIMING OF ACQUISITION
TRANSPLACENTAL
PERINATAL
POSTNATAL
* More likely with mechanical ventilation or indwelling catheters, or after abdominal surgery.
Capretti MG, Lanari M, Lazzarotto T, et al. Very low birth weight infants born to cytomegalovirus-seropositive mothers fed with their mother’s milk: a prospective study. J Pediatr. 2009;154:842-848.
DiGiulio DB, Romero R, Amogan HP, et al. Microbial prevalence, diversity and abundance in amniotic fluid during preterm labor: a molecular and culture-based investigation. PLoS One. 2008;3:e3056.
Horan TC, Andrus M, Dudeck MA. CDC/NHSN surveillance definition of health care-associated infection and criteria for specific types of infections in the acute care setting. Am J Infect Control. 2008;36:309-332.
Mullany LC, Faillace S, Tielsch JM, et al. Incidence and risk factors for newborn umbilical cord infections on Pemba Island, Zanzibar, Tanzania. Pediatr Infect Dis J. 2009;28:503-509.
Stoll BJ, Hansen N, Fanaroff AA, et al. Changes in pathogens causing sepsis in very low birthweight infants. N Engl J Med. 2002;347:240-247.
Stoll BJ, Hansen NI, Sanchez PJ, et al. Early onset neonatal sepsis: the burden of group B streptococcal and E. coli disease continues. Pediatrics. 2011;127(5):817-826.
103.5 Epidemiology of Early- and Late-Onset Neonatal Infections
The terms early-onset infection and late-onset infection refer to the different ages at onset of infection in the neonatal period (Table 103-4). Although these disorders were originally divided arbitrarily into infections occurring before and after 1 wk of life, it is more useful to separate early- and late-onset infections according to peripartum pathogenesis. Early-onset infections are acquired before or during delivery (vertical mother-to-child transmission). Late-onset infections develop after delivery from organisms acquired in the hospital or the community. The age at onset depends on the timing of exposure and virulence of the infecting organism. Very-late-onset infections (onset after 1 mo of life) may also occur, particularly in VLBW preterm infants or term infants requiring prolonged neonatal intensive care.
A study from the National Institute of Child Health and Human Development (NICHD) Neonatal Research Network documented rates of early-onset sepsis among approximately 200,000 live births at Network centers. The overall rate of early-onset sepsis was 1.2 cases per 1000 live births with rates inversely related to birthweight (401-1500 g BW, 12.33/1000; 1501-2500 g BW, 1.96/1000; >2500 g BW, 0.71/1000) (Table 103-5).
Table 103-5 RATES OF EARLY-ONSET SEPSIS PER 1,000 LIVE BIRTHS: NICHD NEONATAL RESEARCH NETWORK/CDC SURVEILLANCE STUDY OF EARLY-ONSET SEPSIS
Prematurity
The most important neonatal factor predisposing to infection is prematurity or LBW. Preterm LBW infants have a 3- to 10-fold higher incidence of infection than full-term normal birthweight infants. Possible explanations are as follows: (1) maternal genital tract infection is considered to be an important cause of preterm labor, with an increased risk of vertical transmission to the newborn (Figs. 103-5 and 103-6); (2) the frequency of intra-amniotic infection is inversely related to gestational age (see Fig. 103-3); (3) premature infants have documented immune dysfunction; and (4) premature infants often require prolonged intravenous access, endotracheal intubation, or other invasive procedures that provide a portal of entry or impair barrier and clearance mechanisms.
Nosocomial Infections
Coagulase-negative staphylococci are the most frequent neonatal nosocomial pathogens. In a cohort of 6,215 VLBW infants in the NICHD Neonatal Research Network, gram-positive agents were associated with 70%, gram-negative with 18%, and fungi with 12% of cases of late-onset sepsis (Table 103-6). Coagulase-negative staphylococcus, the single most common organism, was isolated in 48% of these infections. The emergence of nosocomial bacterial pathogens resistant to multiple antibiotics is a growing concern. Among NICU patients, methicillin-resistant S. aureus, vancomycin-resistant enterococci, and multidrug-resistant gram-negative pathogens are particularly alarming. Organisms responsible for all categories of neonatal sepsis and meningitis may change with time (Table 103-7).
Table 103-6 DISTRIBUTION OF PATHOGENS ASSOCIATED WITH THE 1ST EPISODE OF LATE-ONSET SEPSIS IN VERY LOW BIRTHWEIGHT INFANTS*
ORGANISM† | N | % |
---|---|---|
Gram-positive organisms: | 922 | 70.2 |
Staphylococcus—coagulase negative | 629 | 47.9 |
Staphylococcus aureus | 103 | 7.8 |
Enterococcus spp. | 43 | 3.3 |
Group B streptococci | 30 | 2.3 |
Other | 117 | 8.9 |
Gram-negative organisms: | 231 | 17.6 |
Escherichia coli | 64 | 4.9 |
Klebsiella | 52 | 4.0 |
Pseudomonas | 35 | 2.7 |
Enterobacter | 33 | 2.5 |
Serratia | 29 | 2.2 |
Other | 18 | 1.4 |
Fungi: | 160 | 12.2 |
Candida albicans | 76 | 5.8 |
Candida parapsilosis | 54 | 4.1 |
Other | 30 | 2.3 |
TOTAL | 1,313 | 100 |
* National Institute of Child Health and Human Development Neonatal Research Network, September 1, 1998, through August 31, 2000.
† Patients with dual infections and patients with presumed coagulase-negative staphylococci (CONS) contaminants excluded. According to the definitions in text, 276 (44%) CONS were definite infections and 353 (56%) were possible infections.
From Stoll BJ, Hansen N, Fanaroff AA, et al: Late-onset sepsis in very low birthweight neonates: the experience of the NICHD Neonatal Research Network, Pediatrics 110:285–291, 2002.
Berrebi A, Assouline C, Bessieres MH, et al. Long-term outcome of children with congenital toxoplasmosis. Am J Obstet Gynecol. 2010;203:552e1-552e6.
Bizzarro MJ, Jiang Y, Hussain N, et al. The impact of environmental and genetic factors on neonatal late-onset sepsis. J Pediatr. 2011;158:234-238.
Centers for Disease Control and Prevention. Prevention of perinatal group B streptococcal disease—revised guidelines from the CDC. MMWR. 2010;59(RR-10):1-32.
Cordero L, Ayers LW, Miller RR, et al. Surveillance of ventilator-associated pneumonia in very-low-birth-weight infants. Am J Infect Control. 2002;30:32-39.
Heath PT, Balfour GF, Tighe H, et al. Group B streptococcal disease in infants: a case control study. Arch Dis Child. 2009;94:674-680.
Karlowicz MG, Buescher ES, Surka AE. Fulminant late-onset sepsis in a neonatal intensive care unit, 1988–1997, and the impact of avoiding empiric vancomycin therapy. Pediatrics. 2000;106:1387-1390.
Levent F, Baker CJ, Rench MA, et al. Early outcomes of group B streptococcal meningitis in the 21st century. Pediatr Infect Dis J. 2010;29:1009-1012.
Manzoni P, Rinaldi M, Cattani S, et al. Bovine lactoferrin supplementation for prevention of late-onset sepsis in very low-birth-weight neonates. JAMA. 2009;302:1421-1428.
Muller-Peabody B, Johnson AP, Heath PT, et al. Empirical treatment of neonatal sepsis: are the current guidelines adequate? Arch Dis Child Fetal Neonatal Ed. 2011;96:F4-F8.
Newman TB, Puopolo KM, WI S, et al. Interpreting complete blood counts soon after birth in newborns at risk for sepsis. Pediatrics. 2010;126(5):903-909.
Oddie S, Embleton ND. Risk factors for early onset neonatal group B streptococcal sepsis: case-control study. Br Med J. 2002;325:308.
Phares CR, Lynfield R, Farley MM, et al. Epidemiology of invasive group B streptococcal disease in the United States, 1999–2005. JAMA. 2008;299:2056-2065.
Ronnestad A, Abrahamsen TG, Medbo S, et al. Late-onset septicemia in a Norwegian national cohort of extremely premature infants receiving very early full human milk feeding. Pediatrics. 2005;115:e269-e276.
Ronnestad A, Abrahamsen TG, Medbo S, et al. Septicemia in the first week of life in a Norwegian national cohort of extremely premature infants. Pediatrics. 2005;115:e262-e268.
Samanta S, Farrer K, Breathnach A, et al. Risk factors for late onset gram-negative infections: a case control study. Arch Dis Child Fetal Neonatal Ed. 96, 2011. F15–F14
Stoll BJ, Hansen N, Fanaroff AA, et al. Late-onset sepsis in very low birthweight neonates: the experience of the NICHD Neonatal Research Network. Pediatrics. 2002;110:285-291.
Stoll BJ, Hansen N, Higgins RD, et al. Very low birth weight preterm infants with early onset neonatal sepsis: the predominance of gram-negative infections continues in the NICHD Neonatal Research Network, 2002–2003. Pediatr Infect Dis J. 2005;24:635-639.
Vergnano S, Menson E, Kennea N, et al. Neonatal infections in England: the NeonIN surveillance network. Arch Dis Child Fetal Neonatal Ed. 2011;96:F9-F14.
103.6 Clinical Manifestations of Transplacental Intrauterine Infections
Infection with agents that cross the placenta (CMV, T. pallidum, T. gondii, rubella, parvovirus B19) may be asymptomatic at birth or may cause a spectrum of disease ranging from relatively mild symptoms to multisystem involvement with severe and life-threatening complications. For some agents, disease is characterized by chronicity, recurrence, or both, and the agent may cause ongoing injury. Clinical signs and symptoms do not help make a specific etiologic diagnosis but, rather, raise suspicion of an intrauterine infection and help distinguish these infections from acute bacterial infections that occur during labor and delivery. The following signs and symptoms are common to many of these agents (Table 103-8): intrauterine growth restriction, microcephaly or hydrocephalus, intracranial calcifications, chorioretinitis, cataracts, myocarditis, pneumonia, hepatosplenomegaly, direct hyperbilirubinemia, anemia, thrombocytopenia, hydrops fetalis, and skin manifestations. Many of these agents cause late sequelae, even if the infant is asymptomatic at birth. These adverse outcomes include sensorineural hearing loss, visual disturbances (including blindness), seizures, and neurodevelopmental abnormalities.
Table 103-8 CLINICAL MANIFESTATIONS OF TRANSPLACENTAL INFECTIONS
MANIFESTATION | PATHOGEN |
---|---|
Intrauterine growth restriction | CMV, Plasmodium, rubella, toxoplasmosis, Treponema pallidum, Trypanosoma cruzi, VZV |
Congenital anatomic defects: | |
Cataracts | Rubella |
Heart defects | Rubella |
Hydrocephalus | HSV, lymphocytic choriomeningitis virus, rubella, toxoplasmosis |
Intracranial calcification | CMV, HIV, toxoplasmosis, T. cruzi |
Limb hypoplasia | VZV |
Microcephaly | CMV, HSV, rubella, toxoplasmosis |
Microphthalmos | CMV, rubella, toxoplasmosis |
Neonatal organ involvement: | |
Anemia | CMV, parvovirus, Plasmodium, rubella, toxoplasmosis, T. cruzi, T. pallidum |
Carditis | Coxsackieviruses, rubella, T. cruzi |
Encephalitis | CMV, enteroviruses, HSV, rubella, toxoplasmosis, T. cruzi, T. pallidum |
Hepatitis | CMV, enteroviruses, HSV |
Hepatosplenomegaly | CMV, enteroviruses, HIV, HSV, Plasmodium, rubella, T. cruzi, T. pallidum |
Hydrops | Parvovirus, T. pallidum, toxoplasmosis |
Lymphadenopathy | CMV, HIV, rubella, toxoplasmosis, T. pallidum |
Osteitis | Rubella, T. pallidum |
Petechiae, purpura | CMV, enteroviruses, rubella, T. cruzi |
Pneumonitis | CMV, enteroviruses, HSV, measles, rubella, toxoplasmosis, T. pallidum, VZV |
Retinitis | CMV, HSV, lymphocytic choriomeningitis virus, rubella, toxoplasmosis, T. pallidum, West Nile virus |
Rhinitis | Enteroviruses, T. pallidum |
Skin lesions | Entroviruses, HSV, measles, rubella, T. pallidum, VZV |
Thrombocytopenia | CMV, enteroviruses, HIV, HSV, rubella, toxoplasmosis, T. pallidum |
Late sequelae: | |
Convulsions | CMV, enteroviruses, rubella, toxoplasmosis |
Deafness | CMV, rubella, toxoplasmosis |
Dental/skeletal problems | Rubella, T. pallidum |
Endocrinopathies | Rubella, toxoplasmosis |
Eye pathology | HSV, rubella, toxoplasmosis, T. cruzi, T. pallidum. VZV |
Hepatitis | Hepatitis B |
Mental retardation | CMV, HIV, HSV, rubella, toxoplasmosis, T. cruzi, VZV |
Nephrotic syndrome | Plasmodium, T. pallidum |
CMV, cytomegalovirus; HSV, herpes simplex virus; VZV, varicella-zoster virus.
Bacterial Sepsis
Neonates with bacterial sepsis may have either nonspecific signs and symptoms or focal signs of infection (Tables 103-9 and 103-10), including temperature instability, hypotension, poor perfusion with pallor and mottled skin, metabolic acidosis, tachycardia or bradycardia, apnea, respiratory distress, grunting, cyanosis, irritability, lethargy, seizures, feeding intolerance, abdominal distention, jaundice, petechiae, purpura, and bleeding. International criteria for bacterial sepsis are listed in Table 103-11. The initial manifestation may involve only limited symptomatology and only 1 system, such as apnea alone or tachypnea with retractions or tachycardia, or it may be an acute catastrophic manifestation with multiorgan dysfunction. Infants should be reevaluated over time to determine whether the symptoms have progressed from mild to severe. Later complications of sepsis include respiratory failure, pulmonary hypertension, cardiac failure, shock, renal failure, liver dysfunction, cerebral edema or thrombosis, adrenal hemorrhage and/or insufficiency, bone marrow dysfunction (neutropenia, thrombocytopenia, anemia), and disseminated intravascular coagulopathy (DIC).
Table 103-9 INITIAL SIGNS AND SYMPTOMS OF INFECTION IN NEWBORN INFANTS
GENERAL
GASTROINTESTINAL SYSTEM
RESPIRATORY SYTEM
RENAL SYSTEM
CARDIOVASCULAR SYSTEM
CENTRAL NERVOUS SYSTEM
HEMATOLOGIC SYSTEM
IMCI CRITERIA FOR SEVERE BACTERIAL INFECTION* | WHO YOUNG INFANT STUDY GROUP† | |
---|---|---|
Convulsions | X | X |
Respiratory rate >60 breaths/min | X | X (divided by age group) |
Severe chest indrawing | X | X |
Nasal flaring | X | |
Grunting | X | |
Bulging fontanel | X | |
Pus draining from the ear | X | |
Redness around umbilicus extending to the skin | X | |
Temperature >37.7°C (or feels hot) or <35.5°C (or feels cold) | X | X |
Lethargic or unconscious | X | X (not aroused by minimal stimulus) |
Reduced movements | X | X (change in activity) |
Not able to feed | X | X (not able to sustain such) |
Not attaching to the breast | X | |
No suckling at all | X | |
Crepitations | X | |
Cyanosis | X | |
Reduced digital capillary refill time | X |
IMCI, Integrated Management of Childhood Illness; WHO, World Health Organization.
* Any of the signs listed implies high suspicion for serious bacterial infection.
† Each symptom or sign is associated with a score. The score indicates the probability of disease.
From Vergnano S, Sharland M, Kazembe P, et al: Neonatal sepsis: an international perspective, Arch Dis Child Fetal Neonatal Ed 90:F220–F224, 2005.
A variety of noninfectious conditions can occur together with neonatal infection or can make the diagnosis of infection more difficult. Respiratory distress syndrome (RDS) secondary to surfactant deficiency can coexist with bacterial pneumonia. Because bacterial sepsis can be rapidly progressive, the physician must be alert to the signs and symptoms of possible infection and must initiate diagnostic evaluation and empirical therapy in a timely manner. The differential diagnosis of many of the signs and symptoms that suggest infection is extensive; these noninfectious disorders must also be considered (Table 103-12).
Table 103-12 SERIOUS SYSTEMIC ILLNESS IN NEWBORNS: DIFFERENTIAL DIAGNOSIS OF NEONATAL SEPSIS
CARDIAC
GASTROINTESTINAL
HEMATOLOGIC
METABOLIC
NEUROLOGIC
RESPIRATORY
Systemic Inflammatory Response Syndrome
The clinical manifestations of infection depend on the virulence of the infecting organism and the body’s inflammatory response. The term systemic inflammatory response syndrome (SIRS) is most frequently used to describe this unique process of infection and the subsequent systemic response (Chapter 64). In addition to infection, SIRS may result from trauma, hemorrhagic shock, other causes of ischemia, NEC, and pancreatitis.
Patients with SIRS have a spectrum of clinical symptoms that represent progressive stages of the pathologic process. In adults, SIRS is defined by the presence of 2 or more of the following: (1) fever or hypothermia, (2) tachycardia, (3) tachypnea, and (4) abnormal white blood cell (WBC) count or an increase in immature forms. In neonates and pediatric patients, SIRS manifests as temperature instability, respiratory dysfunction (altered gas exchange, hypoxemia, acute respiratory distress syndrome [ARDS]), cardiac dysfunction (tachycardia, delayed capillary refill, hypotension), and perfusion abnormalities (oliguria, metabolic acidosis) (Table 103-13). Increased vascular permeability results in capillary leak into peripheral tissues and the lungs, with resultant pulmonary and peripheral edema. DIC results in the more severely affected cases. The cascade of escalating tissue injury may lead to multisystem organ failure and death.
Table 103-13 DEFINITIONS OF SYSTEMIC INFLAMMATORY RESPIRATORY RESPONSE SYNDROME AND SEPSIS IN PEDIATRIC PATIENTS
From Adams-Chapman I, Stoll BJ: Systemic inflammatory response syndrome, Semin Pediatr Infect Dis 12:5–16, 2001.
Fever
Only about 50% of infected newborn infants have a temperature higher than 37.8°C (axillary) (Chapter 100). Fever in newborn infants does not always signify infection; it may be caused by increased ambient temperature, isolette or radiant warmer malfunction, dehydration, central nervous system (CNS) disorders, hyperthyroidism, familial dysautonomia, or ectodermal dysplasia. A single temperature elevation is infrequently associated with infection; fever sustained over 1 hr is more likely to be due to infection. Most febrile infected infants have additional signs compatible with infection, although a focus of infection is not always apparent. Acute febrile illnesses occurring later in the neonatal period may be caused by urinary tract infection, meningitis, pneumonia, osteomyelitis, or gastroenteritis, in addition to sepsis, thus underscoring the importance of a diagnostic evaluation that includes blood culture, urine culture, LP, and other studies as indicated (see later). Many agents may cause these late infections, including HSV, enteroviruses, RSV, and bacterial pathogens. In premature infants, hypothermia or temperature instability requiring increasing ambient (isolette, warmer) temperatures is more likely to accompany infection.
Rash
Cutaneous manifestations of infection include impetigo, cellulitis, mastitis, omphalitis, and subcutaneous abscesses. Ecthyma gangrenosum is indicative of infection with Pseudomonas species. The presence of small salmon-pink papules suggests L. monocytogenes infection. A vesicular rash is consistent with herpesvirus infection. The mucocutaneous lesions of Candida albicans are discussed elsewhere (Chapter 226.1). Petechiae and purpura may have an infectious cause. Purple papulonodular lesions are referred to as “blueberry muffin” rash and represent dermal erythropoiesis. Causes include congenital viral infections (CMV, rubella, and parvovirus), congenital neoplastic disease, and Rh hemolytic disease.
Omphalitis
Omphalitis is a neonatal infection resulting from inadequate care of the umbilical cord, which continues to be a problem, particularly in developing countries. The umbilical stump is colonized by bacteria from the maternal genital tract and the environment (Chapter 99). The necrotic tissue of the umbilical cord is an excellent medium for bacterial growth. Omphalitis may remain a localized infection or may spread to the abdominal wall, the peritoneum, the umbilical or portal vessels, or the liver. Abdominal wall cellulitis or necrotizing fasciitis with associated sepsis and a high mortality rate may develop in infants with omphalitis. Prompt diagnosis and treatment are necessary to avoid serious complications.
Pneumonia
The progression of neonatal pneumonia can be variable. Fulminant infection is most commonly associated with pyogenic organisms such as GBS (Chapter 177). Onset may occur during the 1st hours or days of life, with the infant often manifesting rapidly progressive circulatory collapse and respiratory failure. With early-onset pneumonia, the clinical course and radiographs of the chest may be indistinguishable from those with severe RDS.
Buhimschi CS, Buhimschi IA, Abdel-Razeq S, et al. Proteomic biomarkers of intra-amniotic inflammation: relationship with funisitis and early-onset sepsis in the premature neonate. Pediatr Res. 2007;61:318-324.
Centers for Disease Control and Prevention. Possible congenital infection with La Crosse encephalitis virus—West Virginia, 2006–2007. MMWR Morb Mortal Wkly Rep. 2009;58:4-6.
103.7 Diagnosis
The maternal history may provide important information about the mother’s exposure to infection, immunity (natural or acquired), and colonization as well as about obstetric risk factors (prematurity, prolonged ruptured membranes, maternal chorioamnionitis) (see Tables 89-2 and 89-3).
Table 103-14 INDICATIONS FOR INTRAPARTUM ANTIBIOTIC PROPHYLAXIS TO PREVENT EARLY-ONSET GBS DISEASE
INTRAPARTUM GBS PROPHYLAXIS INDICATED | INTRAPARTUM GBS PROPHYLAXIS NOT INDICATED |
---|---|
Previous infant with invasive GBS disease | Colonization with GBS during a previous pregnancy (unless an indication for GBS prophylaxis is present for current pregnancy) |
GBS bacteriuria during any trimester of the current pregnancy | GBS bacteriuria during previous pregnancy (unless another indication for GBS prophylaxis is present for current pregnancy) |
Positive GBS screening culture during current pregnancy (unless a cesarean delivery is performed before onset of labor or amniotic membrane rupture) | Cesarean delivery before onset of labor or amniotic membrane rupture, regardless of GBS colonization status or gestational age |
Unknown GBS status at the onset of labor (culture not done, incomplete, or results unknown) and any of the following: Delivery at <37 weeks’ gestation* Amniotic membrane rupture ≥18 hr Intrapartum temperature ≥100.4°F (≥38.0°C)† Intrapartum NAAT‡ positive for GBS |
Negative vaginal and rectal GBS screening culture in late gestation during the current pregnancy, regardless of intrapartum risk factors |
GBS, group B streptococcus; NAAT, nucleic acid amplification test.
* Recommendations for the use of intrapartum antibiotics for prevention of early-onset GBS disease in the setting of threatened preterm delivery are presented in Figures 103-7 and 103-8.
† If amnionitis is suspected, broad-spectrum antibiotic therapy that includes an agent known to be active against GBS should replace GBS prophylaxis.
‡ If intrapartum NAAT is negative for GBS but any other intrapartum risk factor (delivery at <37 weeks’ gestation, amniotic membrane rupture ≥18 hr, or temperature ≥100.4°F [≥38.0°C]) is present, then intrapartum antibiotic prophylaxis is indicated.
From Verani J, McGee L, Schrag S: Prevention of perinatal group B streptococcal disease—revised guidelines from CDC, 2010, MMWR Recomm Rep 59(RR-10):1–36, 2010.
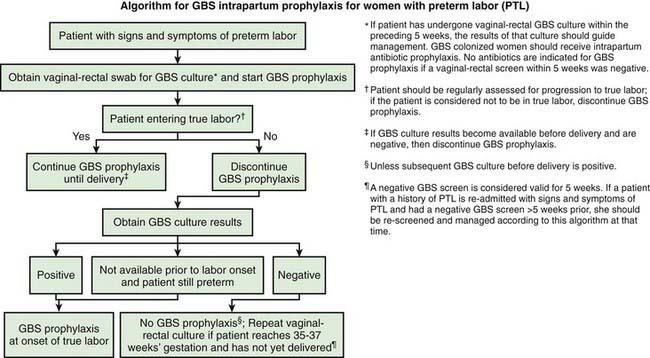
Figure 103-7 Algorithm for group B streptococcus (GBS) intrapartum prophylaxis for women with preterm labor.
(From Verani J, McGee L, Schrag S: Prevention of perinatal group B streptococcal disease—revised guidelines from CDC, 2010, MMWR Recomm Rep 59[RR-10]:1–36, 2010.)
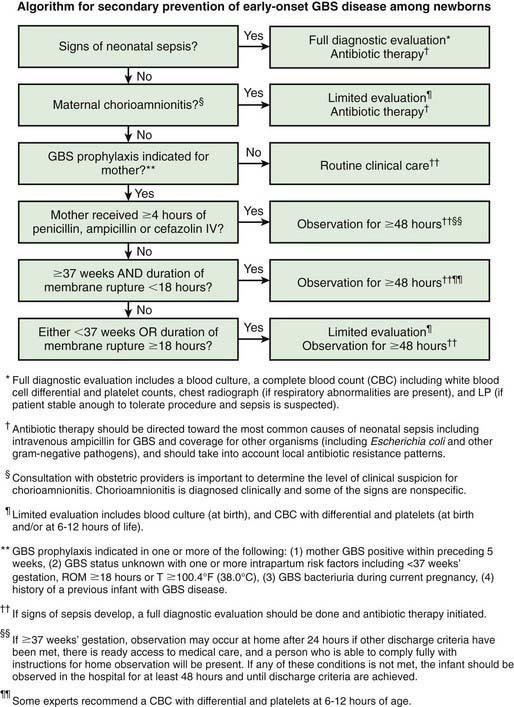
Figure 103-9 Algorithm for secondary prevention of early-onset group B streptococcus (GBS) disease among newborns.
(From Verani J, McGee L, Schrag S: Prevention of perinatal group B streptococcal disease—revised guidelines from CDC, 2010, MMWR Recomm Rep 59[RR-10]:1–36, 2010.)
Suspected Intrauterine Infection
The acronym TORCH refers to toxoplasmosis, other agents (syphilis, varicella, parvovirus B19, many more), rubella, CMV, and HSV. Although the term may be helpful in remembering some of the etiologic agents of intrauterine infection, the TORCH battery of serologic tests has a poor diagnostic yield, and specific diagnostic studies should be selected for each etiologic agent under consideration. CMV and HSV require culture or polymerase chain reaction (PCR) methods; toxoplasmosis is diagnosed by serologic tests and PCR, whereas syphilis and rubella are diagnosed by serologic methods (Table 103-15).
Table 103-15 EVALUATION OF A NEWBORN FOR INFECTION OR SEPSIS
HISTORY (SPECIFIC RISK FACTORS)
EVIDENCE OF OTHER DISEASES*
EVIDENCE OF FOCAL OR SYSTEMIC DISEASE
LABORATORY STUDIES
Evidence of Infection
Evidence of Inflammation
Evidence of Multiorgan System Disease
CSF, cerebrospinal fluid.
* Diseases that increase the risk of infection or may overlap with signs of sepsis.
Suspected Bacterial or Fungal Infections
Documentation of a positive blood culture result is the first diagnostic criterion that must be met for sepsis (see Table 103-15). It is important to note, however, that some patients with bacterial infection may have negative blood culture results (“clinical infection”), and other approaches to identification of infection are needed. A variety of diagnostic markers of infection are being evaluated. Although the total WBC count and differential counts and the ratio of immature to total neutrophils have limitations in sensitivity and specificity, an immature-to-total neutrophil ratio of ≥0.2 suggests bacterial infection. Neutropenia is more common than neutrophilia in severe neonatal sepsis, but neutropenia also occurs in association with maternal hypertension, preeclampsia, and intrauterine growth restriction. Thrombocytopenia is a nonspecific indicator of infection. Tests to demonstrate an inflammatory response include determinations of C-reactive protein, procalcitonin, haptoglobin, fibrinogen, proteomic markers in amniotic fluid, inflammatory cytokines (including IL-6, IL-8, and TNFα), and cell surface markers. It is unclear which surrogate markers for infection are most helpful.
Diagnostic evaluation (including blood culture) is indicated for asymptomatic infants born to mothers with chorioamnionitis. The probability of neonatal infection correlates with the degree of prematurity and bacterial contamination of the amniotic fluid. Some experts recommend presumptive treatment with antibiotics. By contrast, all symptomatic infants should be treated with antibiotics after blood cultures are obtained. There is controversy over whether an LP is necessary for all term infants with suspected early-onset sepsis. If the blood culture result is positive or if the infant becomes symptomatic, LP should definitely be performed. If the mother has been treated with antibiotics for chorioamnionitis, the newborn’s blood culture result may be negative, and the clinician must rely on clinical observation and other laboratory tests (see Fig. 103-9).
Other tests of potential value in evaluating neonates with possible infectious pneumonitis are discussed under diagnosis of infections (Chapter 164). The differential diagnosis of pneumonitis in neonates is broad and includes RDS, meconium aspiration syndrome, persistent pulmonary hypertension, diaphragmatic hernia, transient tachypnea of the newborn, congenital heart disease, and BPD.
Ahmed A, Hickey SM, Ehrett S, et al. Cerebrospinal fluid values in the term neonate. Pediatr Infect Dis J. 1996;15:298-303.
Bang AT, Bang RA, Reddy MH, et al. Simple clinical criteria to identify sepsis or pneumonia in neonates in the community needing treatment or referral. Pediatr Infect Dis J. 2005;24:325-341.
Cobo T, Palacio M, Navarro-Sastre A, et al. Predictive value of combined amniotic fluid proteomic biomarkers and interleukin-6 in preterm labor with intact membranes. Am J Obstet Gynecol. 2009;200:499.e1-499.e6.
El Helali N, Nguyen JC, Ly A, et al. Diagnostic accuracy of a rapid real-time polymerase chain reaction assay for universal intrapartum group B streptococcus screening. Clin Infect Dis. 2009;49:417-423.
Kumar Y, Qunibi M, Neal TJ, et al. Time to positivity of neonatal blood cultures. Arch Dis Child Fetal Neonatal Ed. 2001;85:F182-F186.
Luck S, Sharland M. Postnatal cytomegalovirus: innocent bystander or hidden problem? Arch Dis Child Fetal Neonatal Ed. 2009;94:F58-F64.
Maniaci V, Bauber A, Weiss S, et al. Procalcitonin in young febrile infants for the detection of serious bacterial infections. Pediatrics. 2008;122:701-710.
Miralles R, Hodge R, McParland PC, et al. Relationship between antenatal inflammation and antenatal infection identified by detection of microbial genes by polymerase chain reaction. Pediatr Res. 2005;57:570-577.
Ng PC. Diagnostic markers of infection in neonates. Arch Dis Child Fetal Neonatal Ed. 2004;89:F229-F235.
Stoll BJ, Hansen N, Fanaroff AA, et al. To tap or not to tap: high likelihood of meningitis without sepsis among very low birth weight infants. Pediatrics. 2004;113:1181-1186.
Verboon-Maciolek MA, Krediet TG, Gerards LJ, et al. Severe neonatal parechovirus infection and similarity with enterovirus infection. Pediatr Infect Dis J. 2008;27:241-245.
103.8 Treatment
Treatment of suspected bacterial infection is determined by the pattern of disease and the organisms that are common for the age of the infant and the flora of the nursery. Once appropriate culture specimens have been obtained, intravenous or, less often, intramuscular antibiotic therapy should be instituted immediately. Initial empirical treatment of early-onset bacterial infections should consist of ampicillin and an aminoglycoside (usually gentamicin). Nosocomial infections acquired in a NICU are more likely to be caused by staphylococci, various Enterobacteriaceae, Pseudomonas species, or Candida species. Thus, an antistaphylococcal drug (oxacillin or nafcillin for S. aureus or, more often, vancomycin for coagulase-negative staphylococci or methicillin-resistant S. aureus) should be substituted for ampicillin. A history of recent antimicrobial therapy or the presence of antibiotic-resistant infections in the NICU suggests the need for a different agent. When the history or the presence of necrotic skin lesions suggests Pseudomonas infection, initial therapy should consist of piperacillin, ticarcillin, or ceftazidime and an aminoglycoside. Some experts recommend antifungal prophylaxis with fluconazole for particularly high-risk newborns—that is, those of extremely LBW (<1000 g) and low gestational age (<27 wk). Doses of commonly used antibiotics are provided in Table 103-16. Peak and trough levels of gentamicin (peak, 5-10 µg/mL; trough, <2 µg/mL) and vancomycin (peak, 25-40 µg/mL; trough, <10 µg/mL) are useful to ensure therapeutic levels and minimize toxicity if the agent is administered for more than 2-3 days. The use of antifungal therapy should be considered in VLBW infants who have had previous antibiotic therapy, may have mucosal colonization with C. albicans, and are at high risk for invasive disease (Chapter 226.1).
Meningitis caused by GBS usually responds within 24-48 hr and should be treated for 14-21 days. Gram-negative bacilli may continue to grow from repeated CSF samples for 72-96 hr after therapy despite the use of appropriate antibiotics. Treatment of gram-negative meningitis should be continued for 21 days or for at least 14 days after sterilization of the CSF, whichever is longer. P. aeruginosa meningitis should be treated with ceftazidime. Metronidazole is the treatment of choice for infection caused by B. fragilis. Prolonged antibiotic administration, with or without drainage for treatment and diagnosis, is indicated for neonatal cerebral abscesses. CT scans are recommended for patients with suspected ventriculitis, hydrocephalus, or cerebral abscess (initial and follow-up assessments) and for those with an unexpectedly complicated course (prolonged coma, focal neurologic deficits, persistent or recurrent fever). Neonatal herpes meningoencephalitis should be treated with acyclovir, and empirical therapy should be considered in symptomatic infants with a CSF mononuclear pleocytosis. Pleconaril is the treatment of choice for severe enteroviral infections such as meningoencephalitis, carditis, and hepatitis. Treatment of candidal meningitis is discussed in Chapter 226.
It is important to remember that nonbacterial infectious agents can produce the syndrome of neonatal sepsis. HSV infection requires immediate specific treatment, as does systemic Candida infection. Treatment and other aspects of various nonbacterial infections are discussed in detail in other sections: TB (Chapter 207), syphilis (Chapter 210), genital mycoplasmas (Chapter 216), C. trachomatis (Chapter 218), Candida (Chapter 221), rubella (Chapter 239), enteroviruses (Chapter 242), parvovirus B19 (Chapter 243), HSV (Chapter 244), VZV (Chapter 245), and CMV (Chapter 247).
Brecht M, Clerihew L, McGuire W. Prevention and treatment of invasive fungal infection in very low birthweight infants. Arch Dis Fetal Neonatal Ed. 2009;94:F65-F69.
Darmstadt GL, Batra M, Zaidi KM. Parenteral antibiotics for the treatment of serious neonatal bacterial infections in developing country settings. Pediatr Infect Dis J. 2009;28:S37-S42.
Healy CM, Baker CJ. Fluconazole prophylaxis in the neonatal intensive care unit. Pediatr Infect Dis J. 2009;28:49-51.
Healy CM, Campbell JR, Zaccaria E, et al. Fluconazole prophylaxis in extremely low birth weight neonates reduces invasive candidiasis mortality rates without emergence of fluconazole-resistant Candida species. Pediatrics. 2008;121:703-710.
Lesprit P, Brun-Buisson C. Hospital antibiotic stewardship. Curr Opin Infect Dis. 2008;21:344-349.
Montoya JG, Remington JS. Management of Toxoplasma gondii infection during pregnancy. Clin Infect Dis. 2008;47:554-566.
103.9 Complications and Prognosis
Mortality rates from the sepsis syndrome depend on the definition of sepsis. In adults, the mortality rate approaches 50%, and the rate in newborn infants is probably at least that high. Reported mortality rates in neonatal sepsis are as low as 10% because all bacteremic infections are included in the definition. Several studies have documented that the sepsis case fatality rate is highest for gram-negative and fungal infections (Table 103-17).
Table 103-17 INFECTING PATHOGEN VERSUS DEATH RATE IN LATE-ONSET SEPSIS IN VERY LOW BIRTHWEIGHT INFANTS*
ORGANISM† | N | DEATH N‡ |
---|---|---|
All gram-positive organisms | 905 | 101 (11.2%) |
Staphylococcus—coagulase negative | 606 | 55 (9.1%) |
Staphylococcus aureus | 99 | 17 (17.2%) |
Group B streptococcus | 32 | 7 (21.9%) |
All other streptococci | 65 | 7 (10.8%) |
All gram-negative organisms | 257 | 93 (36.2%) |
Escherichia coli | 53 | 18 (34.0%) |
Klebsiella | 62 | 14 (22.6%) |
Pseudomonas | 43 | 32 (74.4%) |
Enterobacter | 41 | 11 (26.8%) |
Serratia | 39 | 14 (35.9%) |
All fungal organisms | 151 | 48 (31.8%) |
Candida albicans | 82 | 36 (43.9%) |
Candida parapsilosis | 44 | 7 (15.9%) |
* From late-onset sepsis review in VLBW infants, National Institute of Child Health and Human Development Neonatal Research Network, September 1, 1998, through August 31, 2000.
† Organisms found on the last positive blood culture before death or discharge.
‡ The odds ratios for death, with control for gestational age, study center, race, and sex, were as follows: gram-positive vs other infections, 0.26 (0.19-0.35), P <.001; gram-negative vs other infections, 3.5 (2.5-4.9), P <.001; and fungi vs other infections, 2.0 (1.3-3.0), P <.01.
From Stoll BJ, Hansen N, Fanaroff AA, et al: Late-onset sepsis in very low birthweight neonates: the experience of the NICHD Neonatal Research Network, Pediatrics 110:285–291, 2002.
Ameh EA, Nmadu PT. Major complications of omphalitis in neonates and infants. Pediatr Surg Int. 2002;18:413-416.
Chau V, Poskitt KJ, McFadden DE, et al. Effect of chorioamnionitis on brain development and injury in premature infants. Ann Neurol. 2009;66:155-164.
Lahra MM, Beeby PJ, Jeffrey HE. Intrauterine inflammation, neonatal sepsis, and chronic lung disease: a 13-year hospital cohort study. Pediatrics. 2009;123:1314-1319.
Stoll BJ, Hansen NI, Adams-Chapman I, et al. Neurodevelopmental and growth impairment among extremely low-birth-weight infants with neonatal infection. JAMA. 2004;292:2357-2365.
103.10 Prevention
Aggressive management of suspected maternal chorioamnionitis with antibiotic therapy during labor, along with rapid delivery of the infant, reduces the risk of early-onset neonatal sepsis. Vertical transmission of GBS (Chapter 177) is significantly reduced by selective intrapartum chemoprophylaxis. Neonatal infection with Chlamydia can be prevented by identification and treatment of infected pregnant women (Chapter 218). Mother-to-child transmission of HIV is significantly reduced by maternal antiretroviral therapy during pregnancy, labor, and delivery, cesarean section delivery prior to rupture of membranes, and antiretroviral treatment of the infant after birth (Chapter 268).
Prevention of Nosocomial Infection
Principles for the prevention of nosocomial infection include adherence to universal precautions with all patient contact, avoiding nursery crowding and limiting nurse-to-patient ratios, strict compliance with handwashing, meticulous neonatal skin care, minimizing the risk of catheter contamination, decreasing the number of venipunctures and heelsticks, reducing the duration of catheter and mechanical ventilation days, encouraging appropriate advancement of enteral feedings, providing education and feedback to nursery personnel, and ongoing monitoring and surveillance of nosocomial infection rates in the NICU (Table 103-18).
Table 103-18 PRINCIPLES FOR THE PREVENTION OF NOSOCOMIAL INFECTION IN THE NEONATAL INTENSIVE CARE UNIT
CVC, central venous catheter.
From Adams-Chapman I, Stoll BJ: Prevention of nosocomial infections in the neonatal intensive care unit, Curr Opin Pediatr 14:157–164, 2002.
Oral administration of bovine lactoferrin either alone or with a probiotic (Lactobacillus rhamnosus GG) for 30 days in LBW infants has been demonstrated to reduce the incidence of late-onset bacterial and fungal sepsis; probiotics have also reduced the incidence of NEC (Chapter 96.2).
Adams-Chapman I, Stoll BJ. Prevention of nosocomial infections in the neonatal intensive care unit. Curr Opin Pediatr. 2002;14:157-164.
Centers for Disease Control and Prevention. Intrauterine West Nile virus infection—New York, 2002. MMWR Morb Mortal Wkly Rep. 2002;51:1135-1136.
Demirjian A, Levy O. Safety and efficacy of neonatal vaccination. Eur J Immunol. 2009;39:36-46.
Hodge D, Puntis JWL. Diagnosis, prevention, and management of catheter related bloodstream infection during long-term parenteral nutrition. Arch Dis Child Fetal Neonatal Ed. 2002;87:F21-F24.
Kuhn P, Messer J, Paupe A, et al. A multicenter, randomized, placebo-controlled trial of prophylactic recombinant granulocyte-colony stimulating factor in preterm neonates with neutropenia. J Pediatr. 2009;155:324-330.
Rhee V, Mullany LC, Khatry SK, et al. Maternal and birth attendant hand washing and neonatal mortality in southern Nepal. Arch Pediatr Adolesc Med. 2008;162:603-608.
Shennan AH, Chandiramani M. Antibiotics for spontaneous preterm birth. BMJ. 2009;338:306-307.
Van Dyke MK, Phares CR, Lynfield R, et al. Evaluation of universal antenatal screening for group B streptococcus. N Engl J Med. 2009;360:2626-2636.
Verani J, McGee L, Schrag S. Prevention of perinatal group B streptococcal disease—revised guidelines from CDC, 2010. MMWR Recomm Rep. 2010;59(RR-10):1-31.