Chapter 19 Immunological Tolerance
• Immunological tolerance is the state of unresponsiveness to a particular antigen which is primarily established in T- and B-lymphocytes. The clonal receptors of lymphocytes are generated by random recombination of the many genes that code for the antigen binding regions. This creates the need to sort out dangerous receptors that could recognize and destroy self tissues. The breakdown of immunological tolerance to self-antigens is the cause of autoimmune diseases.
• Immunological tolerance is achieved by many different mechanisms operating on different cell types.
• Central tolerance refers to the selection processes which T cell precursors undergo in the thymus before they are released as mature naive T cells. Thymic epithelial cells and dendritic cells present self-antigens to the immature T cell precursors. Those T cell precursors that respond strongly to the self-antigens presented in the thymus undergo apoptosis. This is called negative selection. A specialized population of thymic epithelial cells is capable of expressing genes which are expressed in a strictly organ specific manner (e.g. insulin, which is expressed only in the pancreas and the thymus).
• Peripheral tolerance refers to the diverse mechanism that enforce and maintain T-cell tolerance outside the thymus. These include the prevention of contact between auto-reactive T-cells and their target antigens (immunological ignorance), the peripheral deletion of auto-reactive T-cells by activation induced cell death or cytokine withdrawal, the incapacity of T cells to mount effector responses upon recognizing their target antigen (anergy), and the suppression of immune responses by regulatory T cells.
• B cell tolerance is established by several mechanisms including clonal deletion of autoreactive B cells, mostly in the bone marrow; the rearrangement of autoreactive B cell receptors (receptor editing) or by B cell anergy. In addition B-cell tolerance is maintained by tolerant T cells. The production of high-affinity class-switched antibodies depends on T-cell help. Therefore, if tolerance to a particular antigen is firmly established in the T-cell compartment, B-cells that recognize this antigen will usually remain tolerant.
• To establish or re-establish tolerance is a major goal for innovative treatments for autoimmunity, allergy and transplantation. In contrast, to overcome immunological tolerance is one major goal for innovative treatments against cancer.
Generation of autoreactive antigen receptors during lymphocyte development
The specificity of the antigen receptors of T cells and B cells is the result of random shuffling of the many genes that encode the antigen-binding site of these receptors. Theoretically, this process could generate more than 1015 different T-cell receptors, including some that can bind to autoantigens (Fig. 19.1). Similar considerations apply to B-cell receptors. Cells expressing such receptors are often called self-reactive lymphocytes. The immune system has to fulfill two contradictory requirements: on the one hand the repertoire of different antigen receptors needs to be as large as possible to avoid ‘holes in the repertoire’ that could be exploited by pathogens to evade immune detection. On the other hand, the receptor repertoire must be shaped to prevent the immune system from attacking the organism that harbors it. Any disturbance in this delicately balanced system can have pathogenic or even lethal consequences, either from infections or from the unwanted reaction with autoantigens or harmless external antigens as in allergy. This paradox was recognized at the beginning of the last century by Paul Ehrlich who coined the term ‘horror autotoxicus’ for the necessity to avoid immunological reactions against self-antigens. Tolerance is the process that eliminates or neutralizes such autoreactive cells, and a breakdown of this system can cause autoimmunity. To avoid autoreactivity the randomly generated repertoire of T- and B-cell receptors is censored by several different mechanisms. CD4+ TH cells are pivotal for the multitude of immunological mechanisms that induce and maintain immunological tolerance. In this chapter we will discuss immunological tolerance in B cells and conventional αβ TCR expressing T cells.
T cell tolerance
Central T-cell tolerance develops in the thymus
The chief mechanism of T-cell tolerance is the deletion of self-reactive T cells in the thymus. Immature T cell precursors migrate from the bone marrow to the thymus. There, they proliferate, differentiate and undergo selection processes before a selected few re-enter the blood stream as mature naive T cells. These differentiation and selection processes depend on interactions with thymic epithelial cells and dendritic cells in specialized microenvironments within the thymus (Fig. 19.2).
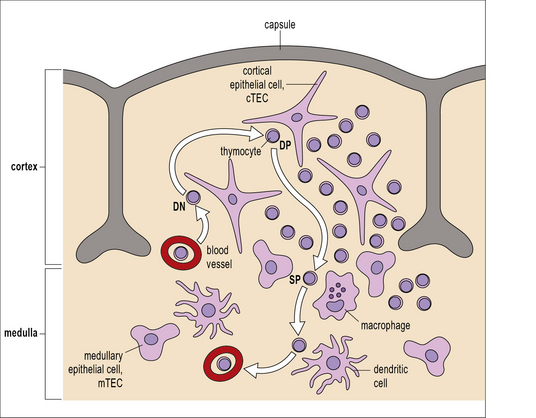
Fig. 19.2 T cell repertoire selection in the thymus
(Adapted from Kyewsky B, Klein L, Ann Rev Immunol 2006;24:571–606.)
Thymocytes are positively selected for their ability to interact with self MHC molecules
• in the DP T cells RAG is active and TCR α chains are continuously rearranged to maximize the chance of producing a TCR capable of interacting with self MHC;
• only upon recognition of a peptide/MHC complex via the TCR is RAG expression halted and the cell is committed to express a particular αβ TCR.
Moreover, recognition of a peptide/MHC complex via the TCR is necessary for the DP T cell to receive a survival signal. This is called positive selection (see Fig 19.2). Experiments have shown that T cells are positively selected by interaction with self-MHC to prepare them for subsequent activation by non-self-peptide/self-MHC complexes, indicating the biological benefit of positive selection.
Lack of survival signals leads to death by neglect
DP T cells whose receptors have a very low affinity for the peptide/MHC complexes they encounter during their approximately 3–4-day lifespan in the thymic cortex do not receive survival signals and undergo apoptosis in the thymus (see Fig. 19.w2). This is called death by neglect. Accordingly, T cells do not progress beyond the DP stage in mice that have been manipulated to lack expression of MHC molecules. Analyses of the germ line repertoire of TCRs have revealed that this unselected repertoire contains more self-MHC reactive TCRs than expected by chance. In other words: co-evolution of TCR and MHC molecules has shaped the germline αβ TCR repertoire to favor the generation of receptors that can interact with self-MHC.
Thymocytes are negatively selected if they bind strongly to self-peptides on MHC molecules
After positive selection and commitment to the CD4 or CD8 lineage in the thymic epithelium, thymocytes express the chemokine receptor CCR7 and migrate towards the central region of the thymus, the thymic medulla, where the CCR7 ligands CCL19 and CCL20 are produced. In the thymic medulla the thymocytes are probed for another 4–5 days. T cells whose receptors have a high affinity for the peptide/MHC complexes encountered in the medulla are autoreactive and therefore, potentially dangerous. They receive death signals and undergo apoptosis. This is called negative selection (see Fig 19.2). Experimental evidence suggests that the majority of the DP T cells which were positively selected are later eliminated by negative selection.
A library of self antigens is presented to developing T cells in the thymus
The induction of central tolerance requires the presence of autoantigens in the thymus. This poses an obvious problem for thymic selection: Some autoantigens, e.g. insulin are expressed in a tissue-specific manner, are frequently called tissue-restricted antigens (TRAs). The question, then, is (how) do TRAs get into the thymus for presentation to developing T cells? Some might be brought into the thymus by immigrating antigen presenting cells but it is highly unlikely that this would yield a reliable representation of the organism’s TRAs. Moreover, developmentally regulated TRAs, e.g. antigens that are only expressed after puberty, would not gain access to the fetal thymus. The answer is that specialized cells in the thymus, the medullary thymic epithelial cells (mTECs) express proteins that are otherwise strictly tissue restricted. This has been called ectopic or promiscuous gene expression (Fig. 19.3).
AIRE controls promiscuous expression of genes in the thymus
What enables mTECs to express a broad array of TRAs independently of spatial or developmental regulation? mTECs express the transcriptional regulator Aire (autoimmune regulator). Aire controls the expression of a large number of TRAs in mTECs (see Fig. 19.3).
Peripheral T-cell tolerance
Immunological ignorance occurs if T cells do not encounter their cognate antigen
The importance of immunological ignorance was demonstrated in mice that express a transgene-encoded TCR which recognizes a peptide derived from the lymphocytic choriomeningitis virus (LCMV). These mice were bred with another transgenic strain that expressed the viral peptide on the surface of their pancreatic islet cells. Surprisingly, diabetes did not develop in the offspring even though in vitro their T cells could kill cells that displayed the viral peptide (Fig. 19.4). The T cells in these double-transgenic mice were therefore, not tolerant in vivo, instead they were ignorant of their target cells.
When the mice were infected with LCMV, the transgenic T cells became activated, invaded the pancreas and destroyed the islet cells. Consequently the mice succumbed to diabetes. Importantly, LCMV-infection did not cause diabetes in those mice that expressed the transgenic TCR but not the LCMV-peptide in the pancreas. Once activated by LCMV infection, the hitherto ignorant autoreactive T cells (autoreactive because the LCMV-derived peptide was transgenically expressed in the pancreas) acquired the capacity to migrate into their target tissue where they recognized and destroyed the LCMV-peptide expressing islet cells (see Fig. 19.4).
Self-reactive T cells and experimental autoimmunity
Q. Why might it be important to maintain these mice in germ-free conditions, to prevent them from getting EAE?
A. Activation of lymphocytes by microorganisms enhances the traffic of lymphocytes into tissues, including the brain (see Chapter 6). If an autoreactive cell then encounters its antigen (MBP) a cycle of inflammation can develop.
Some self antigens are sequestered in immunologically privileged tissues
One explanation is the sequestration of potentially harmful T cells from the tissues in which their target self-antigens are expressed. Sequestration can be achieved when antigens are physically separated from T cells (e.g. by the blood–brain barrier, see Chapter 12). The blood–brain barrier can be surmounted by activated lymphocytes, however, and many organs do not possess a physical barrier to prevent lymphocytes entering from the bloodstream. Instead, lymphocyte migration is controlled by chemokines, selectins and their receptors.
The amount of released self antigen critically affects sensitization
How does the immune system decide which autoreactive T cells may survive ignorantly and which need to be deleted? Antigen dose (Fig. 19.w1) and TCR avidity play a major role. One key experiment used two different strains of mice that expressed ovalbumin (OVA) specifically in the pancreas. One strain expressed OVA at low levels and the other expressed it at high levels. Only in the high-expressing strain was OVA presented to T cells in the draining lymph nodes, resulting in the deletion of adoptively transferred OVA-specific TC cells. In the low-expressing cells the OVA-specific T cells remained ignorant. Further in vitro assays revealed that the low-level OVA-expression was still sufficient to allow recognition and killing of the OVA-expressing pancreatic β cells by the OVA-specific CTL. Therefore, tissue restricted self-antigens need to be expressed at sufficiently high levels to be presented in the draining lymph nodes. These experiments also clearly showed that self-reactive T cells remain dangerous and poised to wreak havoc even if they are temporarily ignorant. In fact, destruction of pancreatic islet cells can induce the release of sufficient amounts of self-antigen to activate OVA-specific CTL in the OVA-low expressing mice.