Chapter 15 Immunity to Protozoa and Worms
• Parasites stimulate a variety of immune defense mechanisms.
• Parasitic infections are often chronic and affect many people. They are generally host specific and most cause chronic infections. Many are spread by invertebrate vectors and have complicated life cycles. Their antigens are often stage specific.
• Innate immune responses are the first line of immune defense.
• T and B cells are pivotal in the development of immunity. Both CD4 and CD8 T cells are needed for protection from some parasites, and cytokines, chemokines, and their receptors have important roles.
• Effector cells such as macrophages, neutrophils, eosinophils, and platelets can kill both protozoa and worms. They secrete cytotoxic molecules such as reactive oxygen radicals and nitric oxide (NO•). All are more effective when activated by cytokines. Worm infections are usually associated with an increase in eosinophil number and circulating IgE, which are characteristic of TH2 responses. TH2 cells are necessary for the elimination of intestinal worms.
• Parasites have many different escape mechanisms to avoid being eliminated by the immune system. Some exploit the host response for their own development.
• Inflammatory responses can be a consequence of eliminating parasitic infections.
• Parasitic infections have immunopathological consequences. Parasitic infections are associated with pathology, which can include autoimmunity, splenomegaly, and hepatomegaly. Much immunopathology may be mediated by the adaptive immune response.
• Vaccines against human parasites are not yet routinely available.
Parasite infections
Parasitic infections typically stimulate a number of immune defense mechanisms, both antibody and cell mediated, and the responses that are most effective depend upon the particular parasite and the stage of infection. Some of the more important parasitic infections of humans (Fig. 15.1) affect the host in diverse ways. Parasitic protozoa may live:
• in the blood (e.g. African trypanosomes);
• within erythrocytes (e.g. Plasmodium spp.);
• in macrophages (e.g. Leishmania spp., Toxoplasma gondii);
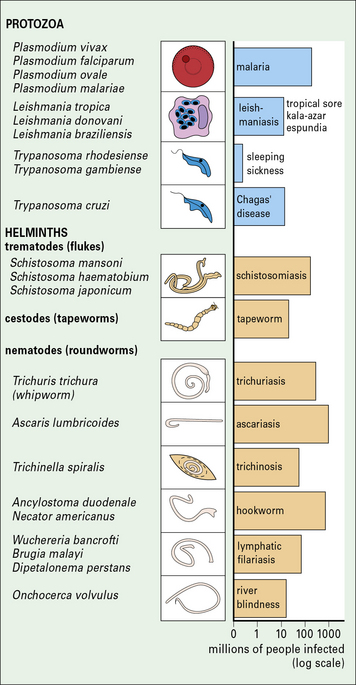
Fig. 15.1 Important parasitic infections of humans
Important parasitic infections, including data from the World Health Organization (1993). Their sizes range from 1 m for the tapeworm to around 10− 5 m for Plasmodium spp. (cf. Fig. 15.w1).
Tapeworms and adult hookworms inhabit the gut, adult schistosomes live in blood vessels, and some filarial worms live in the lymphatics (Fig. 15.2). It is clear that there is widespread potential for damaging pathological reactions.
Parasitic infections are often chronic and affect many people
Parasitic infections present a major medical problem, especially in tropical countries (see Fig. 15.1), for example:
• malaria kills 1–2 million people every year;
• intestinal worms infect one-third of the world’s population – the severity of disease depends upon the worm burden, but in children even moderate intensities of infection may be associated with stunted growth and slow mental development.
Anemia and malnutrition are also associated with parasitic disease.
There are some exceptions to this general rule, for example:
• the protozoan parasite T. gondii is not only able to invade and multiply in all nucleated mammalian cells, but can also infect immature mammalian erythrocytes, insect cell cultures, and the nucleated erythrocytes of birds and fish;
• similarly, the tapeworm of the pig can also infect humans.
Protozoan parasites and worms are considerably larger than bacteria and viruses (Fig. 15.w1), and have very different strategies for avoiding the host immune response.
Q. Apart from size, what is the basic biological difference between bacterial pathogens and parasites, and how would this affect the way they are recognized by the immune system?
A. Bacteria are prokaryotes, whereas parasites are eukaryotes. The plasma membrane and cell wall structures in bacteria are different, so they have distinct pathogen-associated molecular patterns (PAMPs, see Chapter 6). In addition, protein synthesis (initiated with formyl methionine) is different in prokaryotes.
Protozoa that are small enough to live inside human cells have evolved a special mode of entry:
• the merozoite, the invasive form of the blood stage of the malarial parasite, binds to certain receptors on the surface of the erythrocyte and uses a specialized organelle, the rhoptry, to enter the cell.
• Leishmania spp. parasites, which inhabit macrophages, use complement and Fc receptors to encourage the cells to engulf them.
Leishmania can also gain entry to the cell by using the mannose receptor (see Fig. 7.11) on the macrophage surface.
Immune defenses against parasites
Host resistance to parasite infection may be genetic
The resistance of individual hosts to infection varies, and may be controlled by a number of genes. These may be MHC, non-MHC, or other genes (Fig. 15.3).
Host defense depends upon a number of immunological mechanisms
In very general terms, humoral responses are important to eliminate extracellular parasites such as those that live in blood (Fig. 15.4), body fluids, or the gut.
Innate immune responses
Many different cells are involved in generating innate responses including phagocytic cells and NK cells. It is also becoming clear that early recognition of parasites by antigen-presenting cells (APCs), for example dendritic cells, determines the phenotype of the adaptive response (Fig. 15.5).
Q. Which groups of receptors and soluble molecules recognize PAMPs?
A. Toll-like receptors (see Fig. 6.20), the mannose receptor (see Fig. 7.11) and scavenger receptors (see Fig. 7.10) allow phagocytes to directly recognize pathogens. Ficolins, collectins, and pentraxins act as soluble opsonins by binding to pathogen surfaces (see Fig. 6.w3).
While major advances are being achieved in the area of microbial recognition by PRRs, a small but growing number of studies show that parasites also possess specific molecular patterns capable of engaging PRRs. Examples of some parasite PAMPs along with their receptors are given in Figure 15.6.
Toll-like receptors recognize parasite molecules
A few studies have examined the role of TLRs in immunity to parasites. For example:
• T. gondii binds TLR11 via profilin and associates with TLR3, TLR7, and TLR9 via the endoplasmic reticulum protein UNC93B1;
• TLR9 mediates innate immune activation by the malaria pigment hemozoin;
• lyso-phosphatidylserine (lyso-PS) from S. mansoni, glycophosphatidylinositol (GPI) anchors, and Tc52 from T. cruzi are capable of signaling through TLR2.
Interestingly, TLR2 triggering by these diverse parasite patterns leads to different immune outcomes: for S. mansoni, triggering leads to the development of fully mature dendritic cells capable of inducing a Treg response (see Chapter 11), characterized by elevated IL-10 levels; for T. cruzi, mature dendritic cells induce a TH1 response (see Chapter 7) with raised levels of IL-12. This dichotomous response could, in part, be explained by the cooperation between TLR2 and other TLRs. However, there has been difficulty in assigning definitive contributions of TLRs to activation by specific parasite proteins since samples are easily contaminated, e.g. with bacterial PAMPs.
Classical human PRRs also contribute to recognition of parasites
Classical PRRs also play important roles in the innate response to parasite infection (see Fig. 15.6) and include collectins (e.g. MBL), pentraxins (e.g. CRP), C-type lectins (e.g. macrophage mannose receptor, and scavenger receptors (e.g. CD36) – see Chapters 6 and 7. For example, MBL binds mannose-rich LPG from Leishmania, Plasmodium, trypanosomes, and schistosomes; and polymorphisms in the MBL gene are associated with increased susceptibility to severe malaria.
Complement receptors are archetypal PRRs
Complement receptors, in particular CR3, are archetypal PRRs involved in innate immune responses (see Fig. 15.6). They are truly multifunctional, being involved in phagocyte adhesion, recognition, migration, activation, and microbe elimination.
• CR3 offers a multiplicity of binding sites, enabling opsonic or non-opsonic binding;
• phagocytosis by CR3 alone does not generate an oxidative burst in phagocytic cells;
Adaptive immune responses to parasites
T and B cells are pivotal in the development of immunity
The T cell requirement is also demonstrable because nude (athymic) or T-deprived mice fail to clear otherwise non-lethal infections of protozoa such as T. cruzi or Plasmodium yoelii, and T cell-deprived rats fail to expel the intestinal worm Nippostrongylus brasiliensis (Fig. 15.7).
B cells also play key roles in regulating and controlling immunity to parasites. For example:
• B cells and antibodies are required for resistance to the parasitic gastrointestinal nematode Trichuris muris; and
Both CD4 and CD8 T cells are needed for protection from some parasites
• CD4+ T cells mediate immunity against blood-stage P. yoelii;
• CD8+ T cells protect against the liver stage of Plasmodium berghei.
The action of CD8+ T cells is twofold:
• they secrete IFNγ, which inhibits the multiplication of parasites within hepatocytes;
• they are able to kill infected hepatocytes, but not infected erythrocytes.
Regulatory T cells are able to modulate the extremes of both TH2 and TH1 responses.
Cytokines, chemokines, and their receptors have important roles
• the accumulation of macrophages in the granulomas that develop in the liver in schistosomiasis;
• the eosinophilia characteristic of helminth infections; and
• the recruitment of eosinophils and mast cells into the gut mucosa that occurs in worm infections of the gastrointestinal tract.
IL-10 and transforming growth factor-β (TGFβ), the regulatory cytokines (see Chapter 11), downregulate the proinflammatory response and thus minimize pathological damage.