Chapter 12 Immune Responses in Tissues
• A tissue can influence local immune responses, promoting some classes of immunity and suppressing others. Vascular endothelium in each tissue expresses chemokines and adhesion molecules that attract specific subsets of leukocytes.
• Certain sites in the body are immunologically privileged and fully allogeneic tissue can be transplanted into them without risk of rejection. These sites, which include the anterior chamber of the eye and the CNS, promote beneficial classes of immune responses while suppressing classes that can do irreparable local damage.
• The endothelium in the CNS has barrier properties, which exclude most serum proteins from the tissue. Acute inflammation in the CNS is characterized by TH1 cells, TH17 cells and mononuclear phagocytes.
• Immune responses in gut and lung distinguish between pathogens and innocuous organisms and antigens. The immune response in mucosal tissues tends to promote TH2-type responses with IgA production. Gut enterocytes influence the local immune response. Intraepithelial lymphocytes (IELs) respond to stress-induced class Ib molecules and produce many immunomodulatory cytokines. Regulatory T cells normally limit the level of inflammatory reactions.
• T cells are present in normal skin and immune responses are characterized by T-cell infiltration. The endothelium of the dermis attracts TH1 cells, which express cutaneous lymphocyte antigen (CLA) and receptors for IFNγ-induced chemokines.
Tissue-specific immune responses
What determines whether an immune response should be comprised of, for example, activated cytotoxic T lymphocytes (CTLs) or a particular class of antibodies? Although immune responses are primarily tailored to the pathogen, there is also a strong influence from the local tissue, where the immune response occurs (Fig. 12.1).
• the features of immune responses that are unique to individual tissues; and
• the mechanisms by which the tissues influence local and systemic characteristics.
There are several reasons why a particular organ may need to modify local immunity.
Moreover, some types of immune response are only appropriate in specific tissues.
Locally produced cytokine and chemokines influence tissue-specific immune responses
• a tissue can promote some classes of immunity and suppress others;
• the vascular endothelium plays a major role in determining which leukocytes will enter the tissue by secretion of distinct blends of chemokines, and expression of site specific adhesion molecules;
• cells in the tissue can exert their effects via cytokines or by direct cell–cell interactions. In effect, cells of the tissue can signal infection, damage or distress;
• a tissue can have several microenvironments, each of which has its own physiology and preferred immune response;
Endothelium controls which leukocytes enter a tissue
It is now clear that a major element controlling inflammation and the immune response is the vascular endothelium in each tissue, which has its own characteristics; different endothelia produce distinctive blends of chemokines (Fig. 12.2). In addition the endothelium can transport chemokines produced by cells in the tissue from the basal to the lumenal surface by transcytosis, or by surface diffusion in tissues that lack barrier properties (Fig. 12.3).
Hence the different sets of leukocytes present in each tissue can be partly related to the chemokines synthesized by the cells present, particularly the endothelium. For example, in normal lung there is a high level of macrophage migration, which relates to the high expression of CCL2 (macrophage chemotactic protein-1) by lung endothelium. In allergic asthma, the proportion of eosinophils increases, due to the production of IL-5 and CCL3 (eotaxin), which are characteristic of the TH2 response that tends to predominate in mucosal tissues (Fig. 12.4). By contrast, in the CNS lymphocytes and mononuclear phagocytes predominate in most immune reactions.
Immune reactions in the CNS
• the blood–brain barrier (endothelium plus astrocytes) prevents the movement of over 99% of large serum proteins into the brain tissue (IgG, complement, etc.); there are similar barriers in the eye (blood–retinal barrier);
• low levels of MHC molecule expression, and co-stimulatory molecules result in inefficient antigen presentation;
• there is no conventional lymphatic drainage system from brain tissue to local lymph nodes;
• there are low levels of leukocyte traffic into the CNS in comparison with other tissues;
• neurons have direct immunosuppressive actions on glial cells;
• astrocytes, neurons and some glial cells produce immunosuppressive cytokines.
The blood–brain barrier excludes most antibodies from the CNS
The blood–brain barrier is a composite structure formed by the specialized brain endothelium and the foot processes of astrocytes. Astrocytes are required to induce the special properties of brain endothelial cells, which have continuous belts of tight junctions connecting them to other endothelial cells (Fig. 12.5). An estimate of the tightness of the barrier is given by its trans-endothelial resistance, which is up to 2000 Ω/cm2 in the CNS by comparison with values <10 Ω/cm2 in most other tissues. In addition the brain endothelial cells have an array of transporters that allow nutrients into the CNS and a set of multi-drug resistance pumps that prevent many toxic molecules and therapeutic drugs from entering the brain. However, it is the very low permeability of the endothelium to serum proteins which is of particular interest for immunologists (see Fig. 12.5). For example, the level of IgG found in the CNS is normally approximately 0.2% of the level found in serum. The level may rise during an immune reaction as the endothelial barrier becomes more permeable in response to inflammatory cytokines. In some conditions, such as multiple sclerosis, there is often local synthesis of antibody within the CNS, which is reflected in abnormally high antibody levels in cerebrospinal fluid, even accounting for the increased leakage into the CNS. This finding demonstrates that some B cells have migrated into the CNS, and plasma cells have been identified in the spaces surrounding the larger blood vessels. Macrophages also contribute to immune reactions in CNS and they can synthesize some complement components locally (e.g. C3). However the overall level of serum proteins including antibodies and complement rarely exceeds 2% of the levels in serum even in the most severe inflammatory reactions.
Neurons suppress immune reactivity in neighboring glial cells
Subsequently it was found that when neurons are co-cultured in contact with astrocytes, then the ability to induce MHC molecules was suppressed, but if the cells were not in contact, they were not repressed (Fig. 12.6).
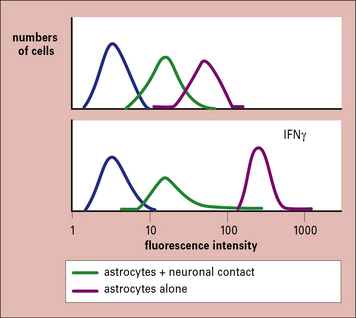
Fig. 12.6 Immunosuppression by neurons
(Based on data from Tonsch U, Rott O. Immunology 1993;80:507.)
• expression of CD200 and fractalkine (a cell surface chemokine CX3CL1) on the surface of neurons inhibits the activation of microglia, by binding to CD200L and the chemokine receptor CX3CR1 respectively, on the microglia;
• neuronal CD47 binds to a microglial signal regulatory protein (SIRP-1α) which inhibits phagocytosis and TNFα production by the microglia.
Immunosuppressive cytokines regulate immunity in the normal CNS
Observations of immune reactions in the CNS have led to the view that TH1-type immune responses with macrophage activation are generally most damaging, as are responses mediated by IL-17-secreting T cells (TH17 cells). By comparison TH2-type immune responses are generally less damaging, and strains of animals that make strong autoantibody responses against CNS antigens, are often less susceptible to CNS pathology than strains that make weaker antibody responses. Certainly, the production of IL-12, IL-23, and TNFα are associated with damaging responses in CNS. Interestingly IFNγ appears to have a dual role, involved in the acute-phase of CNS inflammation, but also necessary for the recovery phase (Fig. 12.7). Such observations have led to the view that immune deviation (the switching of an immune response from TH1 towards a TH2 type) can be protective in the CNS (see Fig. 11.2).
This view is supported by findings that cells of the normal CNS can produce cytokines associated with TH2 responses. For example, astrocytes produce TGF-β, while microglia can produce IL-10 when cocultured with T cells, and both astrocytes and neurons secrete prostaglandins, which inhibit lymphocyte activation. Additionally, several neuropeptides and transmitters (e.g. vaso-active intestinal peptide, VIP) are suppressive. Acute immune responses can develop in the CNS, particularly in susceptible strains, but the normal immunosuppresive controls usually reassert themselves within 1–2 weeks, causing remission. Such a relapsing-remitting pattern of disease occurs in multiple sclerosis and the animal model of CNS inflammation, CREAE (chronic relapsing experimental allergic encephalomyelitis) (Fig. 12.8).
Immune reactions in CNS damage oligodendrocytes
Oligodendrocytes are glial cells that produce the myelin sheaths which act as electrical insulation around nerve axons. When immune reactions occur in the CNS these cells appear to be particularly vulnerable (Fig. 12.9). For example, multiple sclerosis is characterized by focal areas of myelin loss called plaques, typically a few millimeters in diameter. Nerve transmission through these demyelinated areas is seriously impaired, which may cause disease symptoms – weakness and loss of sensation. But it is only in the later stages of the disease that the neurons themselves are damaged.
Immune reactions in the eye
The eye is a complex organ and subject to immune privilege. Indeed the retina and optic nerve are extensions of the CNS, with neurons, glia, and a blood–retinal barrier, which is analagous to the blood–brain barrier (Fig. 12.w1). Also, like the CNS, the eye lacks a conventional lymphatic drainage system.
The eye has powerful immunosuppressive mechanisms
Allogeneic corneal grafting is usually successful as a result of immune privelege, although rejection may occur – the eye has very limited self-regenerative capacity and can be completely destroyed by a cell-mediated immune response with the concomitant local production of TNFα and IFNγ (see Fig. 12.w1). The eye has therefore evolved several major mechanisms to actively suppress cell-mediated responses.
• First, the epithelial cells lining the anterior chamber and the cornea express Fas ligand; corneal allografts that lack Fas ligand (in rats) are almost always rejected. This mechanism is thought to partly protect the cells of the eye from damage by TC cells, if they have become infected by virus. Observations that the expression of Fas ligand is associated with the resolution of anterior uveitis and limited angiogenesis support this view.
• Second, the fluid of the anterior chamber contains cytokines such as transforming growth factor-β (TGFβ) and IL-10, which deviate the immune response towards the less-damaging TH2-type and promote development of regulatory T cells.
• Third, the cells of the iris and ciliary body, secrete immunomodulatory cytokines, including TGFβ, vasoactive intestinal peptide (involved in control of the synthesis of the secretory piece of IgA), γ-melanocyte-stimulating hormone (γMSH), and calcitonin gene-related peptide.
These cytokine and molecular controls on immunity are underpinned by the cellular organization of the eye, which has barriers that limit the movement of molecules into the retina, anterior chamber, and vitreous (Fig. 12.w2). As with the CNS, it is the combination of immunosuppressive and tolerogenic mechanisms which normally maintains immune privelege in the eye.
Local immune privilege may extend systemically
In experiments in mice, where allogeneic tumors were transplanted into the eye, it was found that the recipients became more generally tolerant of grafts which shared the tumor antigens, regardless of the site of transplantation (Fig. 12.w3).
Immune responses in the gut and lung
In contrast to the CNS, the gut and lung are examples of tissues that are continuously in contact with high levels of harmless commensal organisms and innocuous antigens as well as potential pathogens. Mucosal tissues contain a high proportion of the body’s lymphoid tissues, but the responses in these tissues are concerned not just with whether an immune response takes place, but also on the quality of that response. Antigens introduced orally tend to invoke an immune response that is appropriate for the gut and other mucosal surfaces, namely the production of local IgA, and some systemic IgG (Fig. 12.10). There is generally little production of TH1 cells or CTLs, and no cell-mediated immune response.
Q. What factors allow the immune system to distinguish between pathogens and innocuous organisms and antigens?
A. Many pathogenic organisms have components that are recognized by pattern recognition receptors (PRRs) including Toll-like receptors (see Fig. 6.20), which when ligated can lead to efficient antigen presentation.
The gut immune system tolerates many antigens but reacts to pathogens
Q. Can you give an example of tolerance induced by administration of antigen across mucosal tissues?
A. Tolerance in mice administered myelin basic protein (MBP) by aerosol can suppress the induction of experimental allergic encephalomyelitis (EAE) in response to the same antigen given intradermally in adjuvant (see Fig. 11.2).
Oral tolerance, and the related phenomenon of nasal tolerance, illustrate two points:
• it is systemic in that it can influence responses at non-mucosal sites;
• it is dominant in that it is transferable to naive individuals by CD4 (or occasionally CD8) T cells.
• shigellae contain antigens that activate the intracellular PRR, NOD-1 (see Fig. 7.w6);
• the polio genome is ssRNA (recognized by TLR7 and TLR8) and it replicates using a dsRNA intermediate, which is recognized by TLR3. Also, the attenuated polio vaccine proliferates in the gut and lymphoid tissue, and therefore induces tissue damage.
Gut enterocytes influence the local immune response
Intestinal epithelial cells (enterocytes) are the major cell-type forming the epithelium of the gut. These cells have continuous tight junctions and thus form a barrier to prevent antigens from entering the body. The enterocytes of the gut considerably influence the local immune response by secreting a variety of immunomodulatory factors such as TGFβ, VIP, IL-1, IL-6, IL-7, CXCL8, and CCL3. The Paneth cells at the bottoms of the crypts meanwhile produce several natural antibiotic peptides (Fig. 12.11), which help prevent bacterial overgrowth in these sensitive sites of cellular differentiation.
The major areas of gut-associated lymphoid tissue (GALT) include the Peyer’s patches in the small intestine, the lymphoid follicles throughout the gut, and the mesenteric lymph nodes which receive lymph draining from the intestinal villi and the other lymphoid tissues (see Fig. 2.53). It is worth noting that food antigens are primarily present in the small intestine, whereas bacterial antigens are more prevalent in the large intestine.
IELs produce many immunomodulatory cytokines
Tolerance to food antigens depends on a variety of overlapping mechanisms, but the production of TGFβ and IL-10 are particularly important. For example, IL-10 knockout mice develop colitis, if they contact appropriate triggering micro-organisms and in humans, IL-10 is a susceptibility locus for ulcerative colitis. TGFβ is an important element in the TH2 spectrum of cytokines and also induces FoxP3 in naive T cells, thus promoting development of regulatory T cells. Tregs, which are abundant in the lamina propria also produce IL-10 and TGFβ, which further limit inflammatory reactions in the gut (Fig. 12.12). It is not clear exactly how Tregs are stimulated within the gut; they express high levels of TLRs, particularly TLR2, 4, and 8, so they can respond to the high potential load of MAMPs, but they clearly respond differently to APCs and effector CD4+ T cells.
Immune responses in the lung
The lung is another example of a tissue that is in normal contact with external antigens, and pathogens, including bacteria from the upper airways. Particles trapped in the trachea and on the bronchial mucosa are propelled upwards on the ciliary escalator, and this considerably reduces the antigen load reaching the lung. Bronchioles and alveoli contain large numbers of pulmonary macrophages, while the lung tissue also contains lymphocytes and respiratory (plasmacytoid) dendritic cells (pDCs) (Fig. 12.w4). The macrophages and pDCs are major sources of IFNα, and IFNβ, which are particularly important in controlling the initial spread of viral infections.
Immune reactions in the skin
The skin is the largest organ of the body, and in humans there may be up to 106 T cells/cm2, in the dermis, tissue macrophages and at least two major populations of dendritic cells, the Langerhans’ cells in the epidermis and the plasmacytoid dendritic cells, mostly in the dermis. T cells constitute the major cell type present both in normal skin and in immune reactions (Fig. 12.13). They are characterized by a skin-homing marker CLA (cutaneous lymphocyte antigen) and the chemokine receptors CCR4 and CCR6; CCR4 recognizes CCL5 (RANTES) which is strongly expressed by dermal endothelium (see Fig. 12.2). CLA is a sialylated molecule that binds to ELAM-1 (endothelial leukocyte adhesion molecule-1), a lectin-like molecule that is strongly expressed on endothelium in the skin. CLA+ lymphocytes also express high levels of receptors for IL-12 and IL-2 and the chemokine receptor CXCR3, which recognizes IFNγ-induced chemokines. This can explain why active immune responses in skin are characterized by a TH1-type immune response, with high expression of IFNγ and low IL-4. However, as inflammation subsides, there is an increase in IL-4 production and a shift towards a TH2-type response. An outline of the intiation and effector phases of immune responses in the skin (type-IV hypersensitivity) are shown in Figures 26.4–26.7. Immune reactions in skin are also seen in psoriasis and mycosis fungoides, both with strong T cell infiltration.
Galea I., Bechmann I., Perry V.H. What is immune privilege? Trends Immunol. 2007;28:12–18.
Brandtzaeg P. History of oral tolerance and mucosal immunity. Ann N Y Acad Sci. 1996;778:1–27.
Cheroutre H. Starting at the beginning: new perspectives on the biology of mucosal T cells. Annu Rev Immunol. 2004;22:217–246.
Debendictis C., Joubeh S., Zhang G., et al. Immune functions of the skin. Clin Dermatol. 2001;19:573–585.
Engelhardt B., Ransohoff R.M. The ins and outs of T-lymphocyte trafficking to the CNS: anatomical sites and molecular mechanisms. Trends Immunol. 2005;26:485–495.
Fagarasan S., Kawamoto S., Kanagawa O. Adaptive immune regulation in the gut: T cell dependent and T cell independent IgA synthesis. Ann Rev Immunol. 2010;28:243–273.
Greenwood J., Begley D.J., Segal M.B. New concepts of a blood brain barrier. New York: Plenum Press, 1995.
Izcue A., Coombes J.L. Regulatory lymphocytes and intestinal inflammation. Ann Rev Immunol. 2009;27:313–318.
Spellberg B. The cutaneous citadel: a holistic view of skin and immunity. Life Sci. 2002;67:477–502.
Wayne Streilein J. Regional immunology of the eye. In: Pepose J.S., Holland G.N., Wilhelmus K.R. Ocular infection and immunity. Oxford: Elsevier; 1996:19–33.