Chapter 171 Imaging
Trauma
Techniques for Imaging Spine Trauma
Radiographs
Alignment is well assessed with plain films. Soft tissue injury can be inferred from prevertebral soft tissue swelling in the cervical spine. In the lumbar and thoracic spine, paraspinous swelling on the AP view is a sign of acute injury. Otherwise, however, radiographs are insensitive to the detection of significant soft tissue. The sensitivity of plain films to fracture varies depending on the location of the fracture. Vertebral body fractures are usually well visualized on radiographs, but fractures in the dorsal elements can be difficult. Sensitivity of radiographs to dorsal element fractures in the cervical spine has been found to be as low as 50%.1–3 Fractures of the larger vertebrae of the thoracic and lumbar spine are usually well visualized on plain films.
Computed Tomography
CT has assumed a major role in imaging spine trauma because of the technological advances of the multidetector helical technique. It is possible to screen very rapidly for cervical spine trauma with the initial imaging evaluation of a patient with major trauma using a high-resolution technique that permits multiplanar reconstruction. Although not completely eliminated, the limitations of earlier CT technique, such as patient motion, lower resolution in reconstructed images, and relative insensitivity of CT for axially oriented fractures, are greatly diminished. Reformatted CT views in sagittal, coronal, or other planes are essential in such cases, as well as for viewing alignment. As CT increases in speed and technical capability, it has become feasible to incorporate it in a routine manner in the evaluation of major trauma, especially in cervical spine evaluation.4–7
Magnetic Resonance Imaging
MRI is highly sensitive to soft tissues, especially for edema.8–10 MRI is the best method for visualizing the spinal cord. Compression or deformity of the spinal cord, edema, and hemorrhage are visualized well with MRI. It is also excellent for evaluating the intervertebral discs (Fig. 171-1). For example, detection of an acute disc disruption may alter plans for the surgical approach.11 Cases have been reported in which the reduction of a dislocation worsened symptoms because of further herniation or displacement of disc material. MRI permits detection of such herniation before surgery. Ligament disruption can occasionally be directly visualized with MRI, especially the anterior and posterior longitudinal ligaments. Some evidence suggests that the extent of ligament disruption may correlate with the risk of instability in cervical spine dorsal element fractures.
The soft tissues around the vertebra are also visualized with MRI. Extensive edema can serve as a marker for acute injury and the need for further evaluation. Deep, interspinous edema in the setting of acute trauma may be an indication of high risk of instability due to flexion injury. Tears or stretching of the anterior and posterior longitudinal ligaments are especially concerning for instability. MRI can be helpful in a variety of clinical situations in which the combination of clinical and initial imaging findings is ambiguous or nondefinitive. For example, degenerative changes are very common in the cervical spine and can make detection of acute fractures difficult. Although CT can help to identify fractures, subluxation or chronic deformity can still be a challenge. A negative MRI in such situations, showing no evidence of any significant nearby soft tissue edema, makes acute injury unlikely as a cause of subluxation. MRI can be especially helpful when clinical assessment is limited, such as in the obtunded or intubated patient.12
Although it is impossible to specify MRI parameters that should be used because of the great variety of manufacturers, machines, and software available, some broad principles apply. A T2-weighted sequence is important for detecting edema. Fast-spin echo imaging, in which multiple echoes are acquired during each pulse sequence, is nearly always used in standard spine imaging. Such sequences are much faster than spin echo sequences and produce excellent signal-to-noise and high-quality images. Fast-spin echo sequences can be excellent for visualizing the spinal cord, for example. However, it is important to note that fat remains very bright on such sequences, even with T2-weighting. Therefore, if adjacent soft tissue edema is to be demonstrated, different sequences must be used that suppress the signal from fat (Fig. 171-2). A fat saturation pulse can be added to fast-spin echo imaging sequences. Alternatively, inversion recovery sequences (short-tau inversion recovery [STIR]) accomplish the same effect of heavy T2 weighting and fat suppression.
Motion Radiography Studies
Radiographs of the spine in different positions (i.e., flexion and extension views in a lateral position) are excellent for evaluating the stability of the spine in a delayed or chronic setting (Fig. 171-3). Such studies have significant limitations in the acute setting, however. Most importantly, they pose major risks if the spine is in fact unstable. Complications of flexion-extension radiographs are rare but well known. If motion studies are to be undertaken, it is highly desirable that the patient be fully alert, cooperative, and capable of controlling or stopping the motion. If flexion and extension are performed on an obtunded or comatose patient, performing the study under fluoroscopy should improve the safety. The motion can be immediately stopped as soon as subluxation or abnormal movement is visualized. However, data on the safety and accuracy of performing motion radiography on an obtunded patient in the setting of acute injury are very limited. Such studies are often time-consuming, and visualization of the cervicothoracic junction is frequently difficult.
A second limitation in the acute setting is a high incidence of muscle spasm or guarding. From one quarter to one third of patients with acute cervical spine injury may have nondiagnostic results because there is inadequate movement of the neck to assess stability.13 Delayed studies, several weeks after trauma and after muscle spasm has subsided, with the patient cooperative and in control of neck motion, remain the gold standard for evaluating the stability of the cervical spine.
Imaging Findings
Cervicocranial Junction and Upper Cervical Spine
Upper cervical spine injuries can be multiple, complex, and difficult to identify on imaging.
C1 Fractures
The Jefferson burst fracture of C1 is a relatively common injury. CT can demonstrate the multiple fractures of the ring of C1 and the extent of displacement (Fig. 171-4). Plain films usually show prevertebral soft tissue swelling. Some components of the fractures can be visible on plain radiographs, more so if the fractures are displaced. The lateral masses of C1 are likely to be displaced laterally if the transverse ligament is disrupted. Total displacement of greater than 7 mm as seen on an odontoid view has been suggested as a guideline to the presence of transverse ligament rupture.14 The transverse ligament can be visualized directly on MRI, and fluid signal in place of the expected low signal intensity of the ligament is evidence of rupture.15
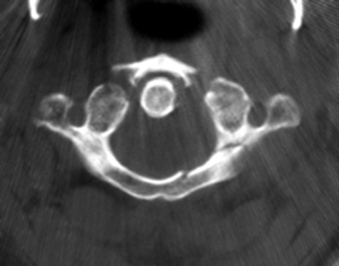
FIGURE 171-4 Jefferson fracture. Patient fell from a ladder onto the head. CT shows multiple fractures of the ring of C1.
The imaging findings just described apply to adults. In at least the first 4 years of life, the lateral masses of C1 often project lateral to the lateral margins of C2 on an AP view.16 Because Jefferson fractures are uncommon in children, CT may be necessary to demonstrate such fractures in children.
Isolated fractures of the dorsal arch of C1 can occur with hyperextension injuries (Fig. 171-5). In such cases, prevertebral soft tissue swelling would likely be absent, and the dorsal arch fracture can be seen on a lateral radiograph. Another type of hyperextension injury at C1 is an avulsion at the ventral caudal portion of the ventral arch, at the attachment of the atlantoaxial ligament. In this case, the fracture is visible on the lateral view, and focal prevertebral soft tissue swelling is usually present.
Transverse Ligament Injury
Transverse ligament rupture can be seen in association with a variety of upper cervical spine fractures, and this possibility should be considered in any such injury. In addition, transverse ligament injury uncommonly may occur without other fractures. Loss of integrity of the transverse ligament can result either from rupture in the midportion of the ligament or from avulsion of the ligament at one of the attachments to the lateral mass of C1. In the latter case, a small fracture is often visible at the tubercle where the ligament attaches (see Fig. 171-26). If the ventral atlantodental space is widened, transverse ligament rupture should be suspected. The ligament itself can be seen directly using MRI. In the case of rupture, fluid signal intensity (bright on T2-weighted images) can be seen in the expected location of the ligament, and fluid is also likely to be present between the dens and ventral arch of C1.
C2 Fractures
Odontoid fractures can occur from a variety of mechanisms. Anderson and D’Alonzo17 described three types of odontoid fractures: type I, an oblique fracture near the apex of the dens; type II, a transverse fracture through the lower third of the dens but above the body of C2 (Fig. 171-6); and type III, which is a fracture below the base of the dens and through the body of C2 (Fig. 171-7). As with transversely oriented fractures elsewhere, odontoid fractures can be difficult to identify by CT. Axial images may show only a region of lucency or subtle gaps in the cortical margin. Reconstructed images from thin-slice axial images, especially from rapid spiral acquisitions, can be very helpful for identifying odontoid fractures on CT. Plain radiographs should be carefully inspected for signs of odontoid fractures, including prevertebral soft tissue swelling, abnormal angulation of the odontoid process, offset of the dens with respect to the body of C2, and disruption of the cortical margin. The type III fracture (or type 3 C2 body fracture) is a horizontally oriented, rostral fracture at the base of the dens. In such fractures, the lateral radiograph shows a break in the apparent ring that results from the superimposition of densities from the junction of the pedicle and body, dens and body, and dorsal cortex of the C2 body.18 The AP or odontoid view usually shows a fracture with inferior convexity.