CHAPTER 257 Image-Guided Robotic Radiosurgery
The CyberKnife
The CyberKnife is the combination of a real-time image guidance system and lightweight linear accelerator (LINAC) mounted on a highly maneuverable industrial robot. The cascade of properties that emerge from this combination includes the ability to correct in real time, with submillimeter accuracy,1–5 for changes in target position; to treat non-isocentrically, as well as isocentrically; to easily fractionate the treatments; to treat intracranial as well as extracranial targets; and to treat moving targets while preserving a tight dosimetry around the lesion.
The heart of the CyberKnife is its image guidance system. During patient setup and repeatedly during treatment, an x-ray imaging system using amorphous silicon detectors positioned on either side of the patient acquires real-time digital radiographs of the region of interest (Fig. 257-1). The images are automatically registered to a library of digitally reconstructed radiographs (DRRs) derived from the treatment planning computed tomography data set. DRR libraries that anticipate the range of patient orientations likely to be experienced are generated. Transformations and rotations can be estimated on both of the x-ray images based on comparison with the DRRs. The resulting calculations are used to ensure proper initial patient setup and to adjust the aim of the treatment beam in response to small patient movements during treatment sessions. In intracranial applications, the targets are detected and tracked on the basis of information from nearby skull anatomy.5 In the spine, target tracking was initially accomplished with the help of radiopaque fiducials implanted in nearby vertebrae.6 However, recent advances (Xsight Spine Tracking System, Accuray, Inc.) have tracked the nearby vertebral anatomy, thereby alleviating the need for fiducials while maintaining submillimeter lesion tracking.2,3
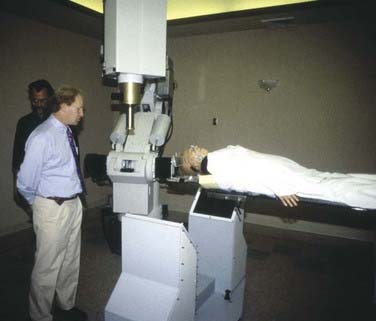
FIGURE 257-1 Photo of the first patient treatment being performed at Stanford in 1994 on the original “alpha” CyberKnife system.
The CyberKnife treatment planning system exploits the robot’s six-degree-of-freedom maneuverability and allows an array of overlapping beams to be superimposed without an isocenter.7 An inverse planning procedure optimizes the set of beam directions and dose to be used on lesions of arbitrary shape and has been demonstrated to deliver dose distributions that closely conform to even highly irregular volumes (see an example of CyberKnife treatment planning in Fig. 257-2).8,9 Even for quite irregularly shaped lesions, this non-isocentric planning can achieve excellent dose homogeneity.8 Similar dose homogeneity may be difficult to achieve with multiple-isocenter methods.9
Perhaps the most important benefit of having successfully eliminated the necessity of the stereotactic frame is the ability to conveniently treat patients in multiple sessions, or fractions. This is a capability that is difficult to match with frame-based radiosurgery. Fractionation is critical for the successful application of radiosurgery outside the central nervous system (CNS) and also has important applications for the treatment of brain and spine lesions that are very near eloquent brain structures and the spinal cord; in such cases, limited fractionation may afford protection to these delicate structures while delivering doses of radiation that control these lesions.10,11 Recently, the American Association of Neurological Surgeons recognized the therapeutic potential of limited fractionation by defining radiosurgery as an ablative procedure that can be performed in one to five fractions.12
Although applications of radiosurgery outside the CNS are of less importance to neurosurgery, the advances of the CyberKnife have expanded radiosurgery out of the confines of neurosurgery, CNS radiation oncology, and neuro-oncology into the general field of oncology. Our most common cancers, prostate and lung malignancies,13,14 can now be targeted with noninvasive fractionated radiosurgery. With prostate tumors, for example, hypofractioned treatment plans allow the delivery of radiation therapy to prostate tumors that is noninvasive (unlike seeds) and has a shorter course (usually five or fewer fractions) than needed with traditional external beam radiation.14,15 The unparalleled ability to track and treat lesions that move with respiration, such as those in the lung and liver, greatly enhances the general utility of the CyberKnife and radiosurgery as a tool in oncology. We believe that it is a neurosurgeon’s role to introduce to our thoracic surgery, surgical oncology, head and neck surgery, and urology colleagues the benefits of radiosurgery and educate them about this tool. Few if any will have had formal exposure to radiosurgery during their training or subsequently.
Clinical Applications of Robotic Image-Guided Radiosurgery
Intracranial Lesions
Frameless radiosurgery has introduced the possibility of treating brain targets with the widest array of penetration trajectories. Rigid frames may exclude the skull base from treatment because the beams cannot pass below the plane of the frame. The CyberKnife can exploit beams penetrating through the splanchnocranium (portion of the skull arising from the first three branchial arches and forming the supporting structure of the jaw) while sparing the brain altogether on the way to extra-axial targets such as vestibular schwannomas or the trigeminal nerve. The ability to hypofractionate treatments may permit safer irradiation of tumors and lesions located close to exquisitely radiosensitive structures such as the optic pathways and brainstem.10,11
The CyberKnife has been used successfully to treat traditional radiosurgery targets, including brain metastases,1 acoustic neuromas,11 arteriovenous malformations,16 and trigeminal neuralgia.17 Outcomes with this frameless radiosurgery approach have been similar to those reported with traditional frame-based treatment. In one study, patients with multiple brain metastases (53 patients, 132 lesions) underwent CyberKnife radiosurgery.18 Overall, 91% of the tumors were controlled with a low rate of radiation necrosis. These findings confirm that frameless CyberKnife radiosurgery can provide control of brain metastases.
The most obvious use of fractionation is around the optic apparatus, such as for meningioma or pituitary adenoma (less commonly metastasis), where doses up to the maximal tolerance of the optic nerves or chiasm rather than the ideal treatment dose for the tumor are frequently delivered with stereotactic radiosurgery. Multiple studies have reported on the safe application of stereotactic radiosurgery for lesions located immediately adjacent to the optic apparatus.10,19,20 In one series of 49 patients with tumors located within 2 mm of the optic pathways, follow-up at a mean of 49 months showed that vision was stable in 38 patients (78%), improved in 8 (16%), and worsened in 3 (6%).10 In the case of visual decline, tumor progression and eventual patient death from the tumor were noted. The treatments were delivered in two to five fractions. These findings suggest that fractionated CyberKnife radiosurgery can be performed safely despite proximity to the optic apparatus and can result in a high rate of tumor control (>92%) at years’ follow-up.
Spinal Lesions
Robotic radiosurgery provides an important new tool for treating a broad spectrum of spinal lesions with the same measure of accuracy and conformality that characterize standard frame-based intracranial radiosurgery.20a Two significant challenges with spinal radiosurgery are tracking of lesions without rigid fixation and the sensitivity of the spinal cord to radiation damage. The CyberKnife addresses the first either through fiducial tracking or, more recently, by tracking of the adjacent vertebral anatomy. Limiting radiation exposure of the spinal cord is accomplished by the submillimeter accuracy of the CyberKnife, which minimizes the dose received by the spinal cord, and by delivering the dose in two to five fractions, which may allow the spinal cord to tolerate higher total radiation doses (however, see Gerszten and colleagues21).
The first clinical report of image-guided robotic spinal radiosurgery demonstrated the safety and short-term efficacy of this treatment for a variety of neoplastic and vascular lesions.6 Staged delivery in two to five sessions was typically used to minimize the risk of radiation injury to the spinal cord. More recently, the safety and efficacy of single-session spinal radiosurgery have been reported in a series of studies by Gerstzen and coworkers.21–25 In the largest prospective series to date, 500 lesions in 393 patients were treated.21 The mean maximum dose was 20 Gy in a single fraction (range, 12.5 to 25 Gy). In the 336 patients treated for pain as the primary indication, long-term pain relief was seen in 86%. Long-term radiographic control was observed in 88% of the 51 patients treated primarily to prevent tumor progression; for breast and lung metastases, tumor control was 100%. The same group recently showed that treatment of metastatic tumors of the spine can be combined with kyphoplasty to repair pathologic compression fractures.26
Extracranial Radiosurgery
The CyberKnife is currently unique in its capability of dynamically treating moving targets. The combination of real-time image guidance and robotic delivery has been described in several reports as a safe and effective, minimally invasive or noninvasive tool to treat thoracic, abdominal, and pelvic lesions.8,13,14,27,28 The maneuverability of the robotic LINAC provides the ability to move the beams synchronously with the target as the patient breathes.29,30 Advantages of radiosurgical treatment of extracranial lesions with image-guided robotic radiosurgery (versus conventional fractionated radiation treatments) include enhanced conformality, sparing of surrounding normal tissue, and capability of delivering the treatment in one or just a few sessions. Several trials are now being performed throughout the world to evaluate the role of extracranial radiosurgery for the treatment of a wide variety of benign and malignant lesions. The impact of radiosurgery on future treatment protocols is, of course, hard to estimate, but on the basis of the explosive growth of the extracranial applications of CyberKnife radiosurgery during recent years, it is foreseeable that image-guided robotic radiosurgery will assume a substantial role in the setting of multimodality cancer therapy protocols.
, Chang SD, Gibbs IC, Sakamoto GT, et al. Staged stereotactic irradiation for acoustic neuroma. Neurosurgery. 2005;56:1254-1261.
, Chang SD, Main W, Martin DP, et al. An analysis of the accuracy of the CyberKnife: a robotic frameless stereotactic radiosurgical system. Neurosurgery. 2003;52:140-146.
, Gerszten PC, Burton SA, Ozhasoglu C, et al. Radiosurgery for spinal metastases: clinical experience in 500 cases from a single institution. Spine. 2007;32:193-199.
, Romanelli P, Adler JRJr. Technology insight: image-guided robotic radiosurgery—a new approach for noninvasive ablation of spinal lesions. Nat Clin Pract Oncol. 2008;5(7):405-414.
, Sinclair J, Chang SD, Gibbs IC, et al. Multisession CyberKnife radiosurgery for intramedullary spinal cord arteriovenous malformations. Neurosurgery. 2006;58:1081-1089.
1 Chang SD, Main W, Martin DP, et al. An analysis of the accuracy of the CyberKnife: a robotic frameless stereotactic radiosurgical system. Neurosurgery. 2003;52:140-146.
2 Ho AK, Fu D, Cotrutz C, et al. A study of the accuracy of CyberKnife spinal radiosurgery using skeletal structure tracking. Neurosurgery. 2007;60:ONS147-156.
3 Muacevic A, Staehler M, Drexler C, et al. Technical description, phantom accuracy, and clinical feasibility for fiducial-free frameless real-time image-guided spinal radiosurgery. J Neurosurg Spine. 2006;5:303-312.
4 Yu C, Main W, Taylor D, et al. An anthropomorphic phantom study of the accuracy of CyberKnife spinal radiosurgery. Neurosurgery. 2004;55:1138-1149.
5 Fu D, Kuduvalli G. Automated skull tracking for the CyberKnife image-guided radiosurgery system. Proc SPIE Med Imaging. 2005;5774:366-376.
6 Ryu SI, Chang SD, Kim DH, et al. Image-guided hypo-fractionated stereotactic radiosurgery to spinal lesions. Neurosurgery. 2001;49:838-846.
7 Adler JRJr, Murphy MJ, Chang SD, et al. Image-guided robotic radiosurgery. Neurosurgery. 1999;44:1299-1306.
8 Collins SP, Coppa ND, Zhang Y, et al. CyberKnife radiosurgery in the treatment of complex skull base tumors: analysis of treatment planning parameters. Radiat Oncol. 2006;1:46.
9 Yu C, Jozsef G, Apuzzo ML, et al. Dosimetric comparison of CyberKnife with other radiosurgical modalities for an ellipsoidal target. Neurosurgery. 2003;53:1155-1162.
10 Adler JRJr, Gibbs IC, Puataweepong P, et al. Visual field preservation after multisession CyberKnife radiosurgery for perioptic lesions. Neurosurgery. 2006;59:244-254.
11 Chang SD, Gibbs IC, Sakamoto GT, et al. Staged stereotactic irradiation for acoustic neuroma. Neurosurgery. 2005;56:1254-1261.
12 Barnett GH, Linskey ME, Adler JR, et al. Stereotactic radiosurgery—an organized neurosurgery-sanctioned definition. J Neurosurg. 2007;106:1-5.
13 Collins BT, Erickson K, Reichner CA, et al. Radical stereotactic radiosurgery with real-time tumor motion tracking in the treatment of small peripheral lung tumors. Radiat Oncol. 2007;2:39.
14 Fuller DB, Naitoh J, Lee C, et al. Virtual HDR CyberKnife treatment for localized prostatic carcinoma: dosimetry comparison with HDR brachytherapy and preliminary clinical observations. Int J Radiat Oncol Biol Phys. 2008;70:1588-1597.
15 Hara R, Itami J, Kondo T, et al. Clinical outcomes of single-fraction stereotactic radiation therapy of lung tumors. Cancer. 2006;106:1347-1352.
16 Sinclair J, Chang SD, Gibbs IC, et al. Multisession CyberKnife radiosurgery for intramedullary spinal cord arteriovenous malformations. Neurosurgery. 2006;58:1081-1089.
17 Lim M, Cotrutz C, Romanelli P, et al. Stereotactic radiosurgery using CT cisternography and non-isocentric planning for the treatment of trigeminal neuralgia. Comput Aided Surg. 2006;11:11-20.
18 Chang SD, Lee E, Sakamoto GT, et al. Stereotactic radiosurgery in patients with multiple brain metastases. Neurosurg Focus. 2000;9(2):e3.
19 Mehta VK, Lee QT, Chang SD, et al. Image guided stereotactic radiosurgery for lesions in proximity to the anterior visual pathways: a preliminary report. Technol Cancer Res Treat. 2002;1:173-180.
20 Pham CJ, Chang SD, Gibbs IC, et al. Preliminary visual field preservation after staged CyberKnife radiosurgery for perioptic lesions. Neurosurgery. 2004;54:799-810.
20a Romanelli P, Adler JRJr. Technology insight: image-guided robotic radiosurgery—a new approach for noninvasive ablation of spinal lesions. Nat Clin Pract Oncol. 2008;5(7):405-414.
21 Gerszten PC, Burton SA, Ozhasoglu C, et al. Radiosurgery for spinal metastases: clinical experience in 500 cases from a single institution. Spine. 2007;32:193-199.
22 Gerszten PC, Burton SA, Belani CP, et al. Radiosurgery for the treatment of spinal lung metastases. Cancer. 2006;107:2653-2661.
23 Gerszten PC, Burton SA, Ozhasoglu C, et al. Stereotactic radiosurgery for spinal metastases from renal cell carcinoma. J Neurosurg Spine. 2005;3:288-295.
24 Gerszten PC, Burton SA, Welch WC, et al. Single-fraction radiosurgery for the treatment of spinal breast metastases. Cancer. 2005;104:2244-2254.
25 Gerszten PC, Ozhasoglu C, Burton SA, et al. CyberKnife frameless stereotactic radiosurgery for spinal lesions: clinical experience in 125 cases. Neurosurgery. 2004;55:89-98.
26 Gerszten PC, Germanwala A, Burton SA, et al. Combination kyphoplasty and spinal radiosurgery: a new treatment paradigm for pathological fractures. J Neurosurg Spine. 2005;3:296-301.
27 Romanelli P, Chang S, Koong A, et al. Extracranial radiosurgery using the CyberKnife. Tech Neurosurg. 2003;9:226-231.
28 Koong AC, Le QT, Ho A, et al. Phase I study of stereotactic radiosurgery in patients with locally advanced pancreatic cancer. Int J Radiat Oncol Biol Phys. 2004;58:1017-1021.
29 Seppenwoolde Y, Berbeco RI, Nishioka S, et al. Accuracy of tumor motion compensation algorithm from a robotic respiratory tracking system: a simulation study. Med Phys. 2007;34:2774-2784.
30 Wong KH, Dieterich S, Tang J, et al. Quantitative measurement of CyberKnife robotic arm steering. Technol Cancer Res Treat. 2007;6:589-594.