12 Image-Guided Adaptive Radiotherapy
Many factors cause anatomic changes to the tumor and normal tissue throughout a course of therapy:


Adaptive radiotherapy can be defined as temporally changing the treatment plan delivered to a patient based on observed anatomic changes caused by tumor shrinkage, weight loss, or internal motion, for example. Adaptive radiotherapy can occur at three different timescales: offline between treatments, online immediately prior to a treatment, and in real time during a treatment. Simple forms of adaptive radiotherapy apply corrective measures based on a predetermined set of scenarios known as a multiadaptive image-guided radiation therapy (IGRT) plan.1 Advanced forms of adaptive radiotherapy use deformable image registration for transferring anatomic contours and dose between images, facilitating automatic treatment planning and deformable dose summation. Even more advanced forms of adaptive radiotherapy reconstruct an estimate of the dose delivered to the patient from in-room imaging procedures and use this estimated delivered dose as a basis for future adaptation.
To put the need for temporally changing radiotherapy into context, we first need to quantify the amount of anatomic change. The magnitude of inter- and intrafraction organ motion was comprehensively reviewed for a variety of treatment sites by Langen and Jones.2 For the prostate, the motion is typically of the order of millimeters with occasional observed motion of more than a centimeter. For the liver, pancreas, and kidney, all studies reviewed (apart from those performed in the standing position) showed an average motion in excess of one centimeter. In addition, tumor shrinkage has been quantified in lung,3–6 cervix,7 and head and neck cancers.8,9 In a series of 10 lung cancer patients imaged daily with megavoltage computed tomography (CT), Kupelian and colleagues3 observed shrinkage in all patients with an average decrease in volume of 1.2% per day (range 0.6% to 2.3%). From serial magnetic resonance imaging (MRI) studies of 34 uterine cervix cancer patients, Mayr and colleagues7 found that half of the tumors regressed to less than 20% of their initial volume and that this shrinkage correlated significantly with clinical outcome. Barker and colleagues8 analyzed daily CT scans from 14 head and neck cancer patients and found a median reduction in the initial gross tumor volume (GTV) of 70%. They also found the tumor shrinkage was frequently asymmetric. In addition to tumor changes, parotid glands also decreased in volume and generally shifted medially, as independently confirmed by Lee and colleagues.10
The radiation oncology community has long known about these anatomic changes; however, what is new are the tools for pretreatment and real-time imaging, deformable image registration, and fast treatment planning that facilitate image-guided adaptive radiotherapy. This chapter describes the clinical rationale for adaptive radiotherapy, the timescales of image-guided adaptive radiotherapy, the tools and workflow implications, as well as quality-assurance issues. Relevant chapters in this book for background to this chapter are “Imaging in Radiation Oncology” (Chapter 8) and “Three-Dimensional Conformal Radiotherapy and Intensity-Modulated Radiation Therapy” (Chapter 10).
Clinical Rationale for Image-Guided Adaptive Radiotherapy
In a pioneering work on the clinical implementation of an offline adaptive radiotherapy process, Martinez and collegues11 described a 150-patient prostate cancer protocol in which repeat CT scans and portal images from the first week of treatment were used to define a patient-specific PTV,12 which was used to re-plan the patient’s subsequent treatments. The patient-specific PTVs were decreased by 24% with a range of 5% to 43%. They found that the level of dose escalation achievable on a per-patient basis was variable. For intensity-modulated radiation therapy the average dose increase with adaptive radiotherapy was 7.5%, with a 2.5% to 15% range.
The offline approach does not reduce the random component of error. The effectiveness of these techniques depends on the relative magnitude of random error, which contributes to systematic error correction uncertainty and potential clinical target volume (CTV) underdoses resulting from large daily fluctuations in target location. Online image-guided implementation uses each day’s estimated anatomy to correct for both random and systematic organ motion. Ghilezan and colleagues13 demonstrated that “idealized” online adaptive radiotherapy for prostate cancer (exactly reproducing plan optimization on each day’s anatomy), could escalate doses by 13% (relative to a fixed level of severe rectal complications) to more than 50% for relative radiosensitive structures (bladder). However, such conformality is achievable only if the uncertainty of online anatomy estimates is small relative to the daily random error.14
Motion and tumor shrinkage, as described in the introduction, make head and neck, thoracic, and abdominal tumor sites good candidates for image-guided adaptive radiotherapy. In describing a feasible online adaptive radiotherapy strategy of deforming intensities, Mohan and colleagues15 found a substantial decrease of coverage of the CTV and lymph nodes without adaptation resulting from the changing anatomy; however, with adaptation, target coverage was maintained. Similarly, Lee and colleagues16 found a 15% daily variation in the delivered versus planned mean parotid dose of head and neck cancer patients resulting from parotid migration into the high-dose region progressively throughout treatment. For stage I non–small cell lung cancer, Underberg and colleagues17 found significant (>5 mm) shifts of the average tumor position with respect to the bony anatomy in 26% to 43% of cases. Tumor shifting toward other normal tissues (e.g., the esophagus and spinal cord) may result in unintentional dose increases to these structures, which could be ameliorated by adaptive strategies. Ramsey and colleagues18 conducted a planning study on lung patients imaged with daily megavoltage CT. The observed tumor shrinkage (60%-80% through the course of treatment) was taken into account in their study, and they demonstrated a significant (17%-23%) reduction in the volume of dose, receiving more than 20 Gy in the ipsilateral lung. A caveat to focusing on the shrinking gross tumor alone is that there is concern about the lack of coverage of the subclinical disease in the CTV.19
An important consideration when performing image-guided adaptive radiotherapy with x-ray–producing devices is the dose received by the patient. The benefit of the use of the x-ray imaging devices must be outweighed by the benefit to the patient of more accurate treatment to obtain the optimum trade off for imaging frequency. A discussion of the dose from imaging procedures and guidelines for use is summarized in the American Association of Physicists in Medicine (AAPM) Task Group 75.20
To date, there are no known prospective randomized trials comparing adaptive radiotherapy and standard-of-care, and the ethics and viability of such a protocol are an issue of much debate.21 It is the responsibility of the scientific community to develop carefully designed studies to quantify the clinical effect of adaptive radiotherapy and make a compelling argument for its use.
Timescales of Image-Guided Adaptive Radiotherapy
There are three timescales for image-guided adaptive radiotherapy: offline, online, and real-time as depicted schematically in Fig. 12-1. As the time between the intervention is reduced from offline through to real-time adaptation, the reliance on automated processes is increased. The increased potential for anatomic conformality offered by adaptation on a shorter timescale also increases the error risk of adaptive processes and careful human operator oversight is necessary.
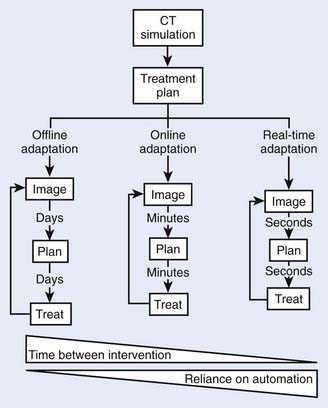
FIGURE 12-1 • The different timescales—and associated reliance on automation—for image-guided adaptive radiotherapy.
A key tool for image-guided adaptive radiotherapy is deformable image registration that allows dose and contour information to be transferred between images, substantially reducing workload. As the timescales for adaptation decrease, the computation time of deformable image registration must correspondingly decrease. Development of an effective image registration technique has been one of the most important areas of research in medical applications of imaging technology.22–28 Briefly, registration means to align two imaging data sets in a common coordinate space by transforming one image set while keeping the other one fixed. Deformable registration is complicated because it entails modeling of voxel-dependent distortion. Clinically, the need for robust deformable image registration algorithms to fuse images representing the same structures imaged in different conditions or on different modalities is ever increasing because of the extensive use of multimodality imaging and the emergence of new imaging techniques and methods. Examples of such applications in adaptive radiotherapy include (1) better tumor target definition,23 (2) image enhancement,29,30 (3) propagation of organ contours from one image set to another,31,32 (4) calculation of accumulated dose in organs experiencing inter- or intrafraction organ deformation for 4-D or adaptive therapy planning,24,33–38 and (5) 4-D image reconstruction.39–42
According to the nature of image matching, registration can be broadly divided into manual, landmark-based, surface-based, intensity-based, and mutual information–based registrations. There are numerous algorithms developed for different applications in medical sciences and other fields. Depending on the mechanism or method used to model the deformation, registration can usually be categorized into elastic model,43–45 viscous fluid model,46 optical flow model,47–49 finite element model,44,50 radial-basis function model such as basis spline model,51–53 and thin-plate spline model.54–57 Each of these approaches has its advantages and disadvantages. Deformable image registration is a rapidly evolving area and intense efforts are being made from both academic institutions and industrial companies to make deformable registration a clinical reality.
A significant promise of adaptive radiotherapy is the compensation of uncertainties, including organ deformation and interfraction organ motion as well as dosimetric errors incurred in previous fractions.15,58–61 To realize adaptive radiotherapy clinically, and maximally exploit the potential of this new form of IGRT, a robust planning strategy must be in place to support the decision-making process. In addition, there are a number of other software-related issues, such as visualization of doses, statistical process control, and so on, that need to be resolved before adaptive radiotherapy becomes widely accepted in the clinic.
Offline Image-Guided Adaptive Radiotherapy
Offline adaptation means that the imaging session and treatment session are separate. In Fig. 12-1, the time separation is shown to be on the order of a day or more. Head and neck cancer is particularly amenable to offline adaption because tumor shrinkage is significant. The rationale for offline image-guided adaptive radiotherapy is shown in Fig. 12-2, in which substantial shrinkage of the tumor throughout the course of therapy is observed between the anatomy at the time of the original CT scan and the cone beam CT scans acquired during treatment. A further example of tumor changes during head and neck cancer radiotherapy is given in Fig. 12-3, in which repeat CT scans (the second acquired for re-planning purposes) were acquired. The GTV contours from the original CT scan are shown overlaid on the mid-treatment scan, as well as the new GTV volumes deformably mapped from the original scan to the mid-treatment scan using Mimvista.
The CT scans from Fig. 12-3 were used for treatment planning, with the 95% and 100% isodose curves shown in Fig. 12-4. Notable are the large differences between the treatment volume from the initial scan and the mid-treatment scan. The corresponding dose volume histograms are shown in Fig. 12-5, demonstrating, as expected, that smaller treatment volumes result in significantly reduced normal tissue doses, and conversely, the larger normal tissue doses in the absence of adaptation. Care must be taken, as with all radiotherapy processes, with adaptation. Images are only that, and the interpretation of the images has a large interobserver variability.62–65 Furthermore, questions arise for how to adapt the CTVs, in which by definition there is suspected but no image-visible disease. Further study as to the optimal clinical method or methods for adaptation, which are likely to be tumor-site–specific, is needed.
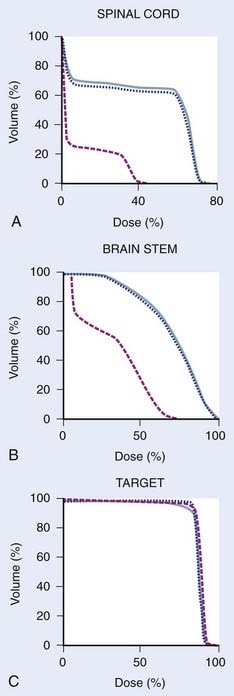
FIGURE 12-5 • Dose volume histograms of spinal cord (A), brain stem (B), and targets of the treatment plans referred to in Figure 12-4 (C). Original treatment plan on the original treatment planning computed tomography (CT) scan (dotted blue); original treatment plan recalculated onto the mid-treatment CT scan indicating the dose in the absence of modification (solid blue); adapted treatment plan (dashed red) (C). A substantial dose reduction to normal tissue is possible in some cases with adaptive radiotherapy in the presence of shrinking tumors.
The imaging system used for offline adaptive radiotherapy is typically either a conventional CT scanner or an in-room CT scanner. The adaptation can be triggered by a protocol (for example after 20 and 40 Gy delivered dose), by clinical observations of masks not fitting, weight loss, or other surface changes, or by changes observed on an in-room imaging system. Because of an increased scatter contribution found in cone beam rather than fan beam images, cone beam CT scans, particularly kilovoltage cone beam CT, can result in cupping artifacts and erroneous electron density values.33,38,66 Improved reconstruction algorithms and other methods to solve or reduce the effects of this problem are actively being developed.
Online Image-Guided Adaptive Radiotherapy
Online adaptation means that the imaging session and treatment session occur in the treatment room within a given treatment fraction. In Fig. 12-1 the time separation is shown to be on the order of minutes or less. Head and neck cancer is particularly amenable to online adaptation, because variable neck flexion can be significant and change the position of the lower neck and supraclavicular node targets with respect to the primary tumor and upper-neck nodal targets from that planned. Involved field prostate radiotherapy is also amenable to online adaptation because the prostate can move independently of the treated pelvic nodes, and therefore the two targets are in different geometric locations from each other to that planned. The rationale for online image-guided adaptive radiotherapy is shown in Fig. 12-6 (head and neck) and Fig. 12-7 (prostate).
Real-Time Image-Guided Adaptive Radiotherapy
Real-time adaptation means that the imaging session and treatment session are intertwined. In Fig. 12-1 the time separation is shown to be on the order of seconds or less. Abdominal and thoracic tumors are particularly amenable to online adaption because in many cases respiration-induced tumor motion is significant. The rationale for real-time image-guided adaptive radiotherapy is shown in Fig. 12-8, in which a significant drift in the mean position of a lung tumor during a stereotactic radiotherapy treatment is demonstrated. Fig. 12-9 highlights the complexity of respiration-induced tumor motion and the challenges of radiotherapy in general, as well as methods to adapt to the motion in real time. Other than real-time image-guided adaptive radiotherapy, there are other options to manage intrafraction motion, particularly respiratory-induced motion. These methods include motion-inclusive methods,67–70 respiratory gating,71–89 abdominal compression,90–100 and breath-hold techniques.101–107 A review and use guidelines for these methods are given in the AAPM Task Group 76 report,108 and more information can be found in a Seminars in Radiation Oncology special issue on intrafraction organ motion and its management, edited by Bortfeld and Chen.109
Real-time image-guided adaptation is clearly the most complex form of adaptation, requiring more imaging, faster decision making, and even more reliance on the system for guidance, plan changes, and fidelity of delivery to moving targets. Desirable characteristics of the ideal imaging and radiation delivery system for real-time adaptive therapy are shown in Table 12-1. Unfortunately, no such imaging or radiation delivery currently exists. Promising real-time volumetric imaging possibilities include MRI,110–113 which may be applicable to the majority of tumor sites (although major implementation challenges need to be addressed); and ultrasound,114–117 which may be useful for pelvic and abdominal tumors. Lower-dimensionality real-time methods are possible through x-ray,84–86,88,89,118,119 combined x-ray and optical imaging,118–121 and electromagnetic target localization122–124 methods that can give measured or estimated positions of a point or several points (typically implanted markers) with time. This signal can be used as an input to a real-time adaptive delivery system.
Table 12-1 Desired Characteristics of In-Room Imaging and Radiation Delivery Systems for Real-Time Image-Guided Adaptive Radiotherapy
Desired Characteristics of an In-Room Imaging System | Desired Characteristics of a Radiation Delivery System |
---|---|
Delivery systems under investigation for real-time adaptation to tumor motion include the CyberKnife robotic treatment system (available clinically),120,121,125,126 the multileaf collimator,127–139 the couch compensation system,140–143 and the gimbaled linear accelerator.144 Proton and heavy-ion treatment systems145,146 are also being investigated for real-time adaptation. Real-time systems must react to change at least as fast as it occurs. A system’s reaction time depends on a number of factors. Time is required to detect a change in the target position, process and filter the position data, communicate with the beam-alignment system, and complete the repositioning of either the beam or patient. If a single-motion detection method (fluoroscopic imaging, for example) is used in a first-order, open-loop system, then all of these time delays combine linearly in series to give the total reaction time.
Acknowledgments
The authors wish to thank Drs. Dimitre Hristov and Billy W. Loo from Stanford University for Fig. 12-8, and the editorial team for their patience with the draft.
2 Langen KM, Jones DT. Organ motion and its management. Int J Radiat Oncol Biol Phys. 2001;50:265-278.
3 Kupelian PA, Ramsey C, Meeks SL, et al. Serial megavoltage CT imaging during external beam radiotherapy for non-small-cell lung cancer: observations on tumor regression during treatment. Int J Radiat Oncol Biol Phys. 2005;63:1024-1028.
4 Bosmans G, van Baardwijk A, Dekker A, et al. Intra-patient variability of tumor volume and tumor motion during conventionally fractionated radiotherapy for locally advanced non-small-cell lung cancer: a prospective clinical study. Int J Radiat Oncol Biol Phys. 2006;66:748-753.
5 Britton KR, Starkschall G, Tucker SL, et al. Assessment of gross tumor volume regression and motion changes during radiotherapy for non-small-cell lung cancer as measured by four-dimensional computed tomography. Int J Radiat Oncol Biol Phys. 2007;68:1036-1046.
6 Siker ML, Tome WA, Mehta MP. Tumor volume changes on serial imaging with megavoltage CT for non-small-cell lung cancer during intensity-modulated radiotherapy: how reliable, consistent, and meaningful is the effect? Int J Radiat Oncol Biol Phys. 2006;66:135-141.
7 Mayr NA, Magnotta VA, Ehrhardt JC, et al. Usefulness of tumor volumetry by magnetic resonance imaging in assessing response to radiation therapy in carcinoma of the uterine cervix. Int J Radiat Oncol Biol Phys. 1996;35:915-924.
8 Barker JL, Garden AS, Ang KK, et al. Quantification of volumetric and geometric changes occurring during fractionated radiotherapy for head-and-neck cancer using an integrated CT/linear accelerator system. Int J Radiat Oncol Biol Phys. 2004;59:960-970.
9 Hansen EK, Bucci MK, Quivey JM, et al. Repeat CT imaging and replanning during the course of IMRT for head-and-neck cancer. Int J Radiat Oncol Biol Phys. 2006;64:355-362.
10 Lee C, Langen KM, Lu W, et al. Evaluation of geometric changes of parotid glands during head and neck cancer radiotherapy using daily MVCT and automatic deformable registration. Radiother Oncol. 2008;89:81-88.
11 Martinez AA, Yan D, Lockman D, et al. Improvement in dose escalation using the process of adaptive radiotherapy combined with three-dimensional conformal or intensity-modulated beams for prostate cancer. Int J Radiat Oncol Biol Phys. 2001;50:1226-1234.
12 Yan D, Lockman D, Brabbins D, et al. An off-line strategy for constructing a patient-specific planning target volume in adaptive treatment process for prostate cancer. Int J Radiat Oncol Biol Phys. 2000;48:289-302.
13 Ghilezan M, Yan D, Liang J, et al. Online image-guided intensity-modulated radiotherapy for prostate cancer: How much improvement can we expect? A theoretical assessment of clinical benefits and potential dose escalation by improving precision and accuracy of radiation delivery. Int J Radiat Oncol Biol Phys. 2004;60:1602-1610.
14 Serago CF, Chungbin SJ, Buskirk SJ, et al. Initial experience with ultrasound localization for positioning prostate cancer patients for external beam radiotherapy. Int J Radiat Oncol Biol Phys. 2002;53:1130-1138.
15 Mohan R, Zhang X, Wang H, et al. Use of deformed intensity distributions for on-line modification of image-guided IMRT to account for interfractional anatomic changes. Int J Radiat Oncol Biol Phys. 2005;61:1258-1266.
16 Lee C, Langen KM, Lu W, et al. Assessment of parotid gland dose changes during head and neck cancer radiotherapy using daily megavoltage computed tomography and deformable image registration. Int J Radiat Oncol Biol Phys. 2008;71:1563-1571.
17 Underberg RW, Lagerwaard FJ, van Tinteren H, et al. Time trends in target volumes for stage I non-small-cell lung cancer after stereotactic radiotherapy. Int J Radiat Oncol Biol Phys. 2006;64:1221-1228.
18 Ramsey CR, Langen KM, Kupelian PA, et al. A technique for adaptive image-guided helical tomotherapy for lung cancer. Int J Radiat Oncol Biol Phys. 2006;64:1237-1244.
19 Giraud P, Antoine M, Larrouy A, et al. Evaluation of microscopic tumor extension in non-small-cell lung cancer for three-dimensional conformal radiotherapy planning. Int J Radiat Oncol Biol Phys. 2000;48:1015-1024.
20 Murphy MJ, Balter J, Balter S, et al. The management of imaging dose during image-guided radiotherapy: report of the AAPM Task Group 75. Med Phys. 2007;34:4041-4063.
21 Bentzen SM. Randomized controlled trials in health technology assessment: overkill or overdue? Radiother Oncol. 2008;86:142-147.
22 Mackie TR, Kapatoes J, Ruchala K, et al. Image guidance for precise conformal radiotherapy. Int J Radiat Oncol Biol Phys. 2003;56:89-105.
23 Xing L, Thorndyke B, Schreibmann E, et al. Overview of image-guided radiation therapy. Med Dosim. 2006;31:91-112.
24 Keall P. 4-dimensional computed tomography imaging and treatment planning. Semin Radiat Oncol. 2004;14:81-90.
25 Foskey M, Davis B, Goyal L, et al. Large deformation three-dimensional image registration in image-guided radiation therapy. Phys Med Biol. 2005;50:5869-5892.
26 Christensen GE, Carlson B, Chao KS, et al. Image-based dose planning of intracavitary brachytherapy: registration of serial-imaging studies using deformable anatomic templates. Int J Radiat Oncol Biol Phys. 2001;51:227-243.
27 Lu W, Chen ML, Olivera GH, et al. Fast free-form deformable registration via calculus of variations. Phys Med Biol. 2004;49:3067-3087.
28 Pelizzari CA. Image processing in stereotactic planning: volume visualization and image registration. Med Dosim. 1998;23:137-145.
29 Thorndyke B, Schreibmann E, Maxim P, et al. Enhancing 4D PET through retrospective stacking. Medical Physics. 2005;32:2096.
30 Li T, Schreibmann E, Thorndyke B, et al. Radiation dose reduction in 4D computed tomography. Medical Physics. 2005;32:2094.
31 Lu W, Olivera GH, Chen Q, et al. Automatic re-contouring in 4D radiotherapy. Phys Med Biol. 2006;51:1077-1099.
32 Chao M, Schreibmann E, Li T, et al: Knowledge-based auto-contouring in 4D radiation therapy. Paper presented at the American Association of Physicists in Medicine Annual Meeting, 2006, Orlando, Florida.
33 Yang Y, Schreibmann E, Li T, et al. Evaluation of on-board kV cone beam CT (CBCT)-based dose calculation. Phys Med Biol. 2007;52:685-706.
34 De la Zerda A, Armbruster B, Xing L: Formulating adaptive radiation therapy planning into closed-loop control framework, Phys Med Biol (in press).
35 De la Zerda A, Armbruster B, Xing L. Inverse planning for adaptive radiation therapy using dynamic algorithm. Int J Radiat Oncol Biol Phys. 2006;66:S123-S124.
36 Keall PJ, Joshi S, Vedam SS, et al. Four-dimensional radiotherapy planning for DMLC-based respiratory motion tracking. Med Phys. 2005;32:942-951.
37 Rosu M, Balter JM, Chetty IJ, et al. How extensive of a 4D dataset is needed to estimate cumulative dose distribution plan evaluation metrics in conformal lung therapy? Med Phys. 2007;34:233-245.
38 Langen KM, Meeks SL, Poole DO, et al. The use of megavoltage CT (MVCT) images for dose recomputations. Phys Med Biol. 2005;50:4259-4276.
39 Li T, Schreibmann E, Yang Y, et al. Motion correction for improved target localization with on-board cone-beam computed tomography. Phys Med Biol. 2006;51:253-267.
40 Li T, Xing L, McGuinness C, et al. Four-dimensional cone-beam CT using an on-board imager. Med Phys. 2006;33:3825-3833.
41 Li T, Thorndyke B, Schreibmann E, et al. Model-based image reconstruction for four-dimensional PET. Med Phys. 2006;33:1288-1298.
42 Zeng R, Fessler JA, Balter JM. Estimating 3-D respiratory motion from orbiting views by tomographic image registration. IEEE Trans Med Imaging. 2007;26:153-163.
43 Bajcsy R, Kovacic S. Multiresolution elastic matching. Comp Vis Graph Image Process. 1989;46:1-21.
44 Gee JC, Haynor DR, Reivich M, et al. Finite element approach to warping of brain images. Proceeding, SPIE Med Imag. 1994;2167:18-27.
45 Gee JC, Reivich M, Bajcsy R. Elastically deforming 3D atlas to match anatomical brain images. J Comput Assist Tomogr. 1993;17:225-236.
46 Christensen GE, Rabitt RD, Miller MI. Deformable templates using large deformable kinematics. IEEE, Trans Med Imaging. 1996;5:1435-1447.
47 Thirion JP. Image matching as the diffusion process: an analogy with Maxwell’s demons. Med Image Analy. 1998;2:243-260.
48 Huang TC, Zhang G, Guerrero T, et al. Semi-automated CT segmentation using optic flow and Fourier interpolation techniques. Comput Methods Programs Biomed. 2006;84:124-134.
49 Wang H, Dong L, Lii MF, et al. Implementation and validation of a three-dimensional deformable registration algorithm for targeted prostate cancer radiotherapy. Int J Radiat Oncol Biol Phys. 2005;61:725-735.
50 Brock KM, Balter JM, Dawson LA, et al. Automated generation of a four-dimensional model of the liver using warping and mutual information. Med Phys. 2003;30:1128-1133.
51 Schreibmann E, Xing L. Image registration with auto-mapped control volumes. Med Phys. 2006;33:1165-1179.
52 Schreibmann E, Yang Y, Boyer A, et al. Image interpolation in 4D CT using a BSpline deformable registration model. Med Phys. 2005;32:1924.
53 Coselmon MM, Balter JM, McShan DL, et al. Mutual information based CT registration of the lung at exhale and inhale breathing states using thin-plate splines. Med Phys. 2004;31:2942.
54 Bookstein FL. Principal warping: Thin plate splines and the decomposition of deformations. IEEE Trans Pattern Analy Machine Intelligence. 1989;11:567-585.
55 Lian J, Xing L, Hunjan S, et al. Mapping of the prostate in endorectal coil-based MRI/MRSI and CT: a deformable registration and validation study. Med Phys. 2004;31:3087-3094.
56 Brock KK, Hollister SJ, Dawson LA, et al. Technical note: creating a four-dimensional model of the liver using finite element analysis. Med Phys. 2002;29:1403-1405.
57 Fei B, Kemper C, Wilson DL. A comparative study of warping and rigid body registration for the prostate and pelvic MR volumes. Comp Med Imag Graph. 2003;4:267-281.
58 Paganetti H, Jiang H, Trofimov A. 4D Monte Carlo simulation of proton beam scanning: Modelling of variations in time and space to study the interplay between scanning pattern and time-dependent patient geometry. Phys Med Biol. 2005;50:983-990.
59 Wu Q, Liang J, Yan D. Application of dose compensation in image-guided radiotherapy of prostate cancer. Phys Med Biol. 2006;51:1405-1419.
60 de la Zerda A, Armbruster B, Xing L. Inverse planning for adaptive radiation therapy using dynamic programming. Paper presented at Annual Meeting of ASTRO, Philadelphia. 2006.
61 Lu W, Chen ML, Olivera GH, et al. Fast free-form deformable registration via calculus of variations. Phys Med Biol. 2004;49:3067-3087.
62 Van de Steene J, Linthout N, de Mey J, et al. Definition of gross tumor volume in lung cancer: Inter-observer variability. Radiother Oncol. 2002;62:37-49.
63 Fiorino C, Reni M, Bolognesi A, et al. Intra- and inter-observer variability in contouring prostate and seminal vesicles: implications for conformal treatment planning. Radiother Oncol. 1998;47:285-292.
64 Fiorino C, Vavassori V, Sanguineti G, et al. Rectum contouring variability in patients treated for prostate cancer: impact on rectum dose-volume histograms and normal tissue complication probability. Radiother Oncol. 2002;63:249-255.
65 Hermans R, Feron M, Bellon E, et al. Laryngeal tumor volume measurements determined with CT: a study on intra- and interobserver variability. Int J Radiat Oncol Biol Phys. 1998;40:553-557.
66 Yoo S, Yin FF. Dosimetric feasibility of cone-beam CT-based treatment planning compared to CT-based treatment planning. Int J Radiat Oncol Biol Phys. 2006;66:1553-1561.
67 Zhang P, Hugo GD, Yan D. Planning study comparison of real-time target tracking and four-dimensional inverse planning for managing patient respiratory motion. Int J Radiat Oncol Biol Phys. 2008;72:1221-1227.
68 Trofimov A, Rietzel E, Lu HM, et al. Temporo-spatial IMRT optimization: concepts, implementation and initial results. Phys Med Biol. 2005;50:2779-2798.
69 Shih HA, Jiang SB, Aljarrah KM, et al. Internal target volume determined with expansion margins beyond composite gross tumor volume in three-dimensional conformal radiotherapy for lung cancer. Int J Radiat Oncol Biol Phys. 2004;60:613-622.
70 Starkschall G, Forster KM, Kitamura K, et al. Correlation of gross tumor volume excursion with potential benefits of respiratory gating. Int J Radiat Oncol Biol Phys. 2004;60:1291-1297.
71 Kubo HD, Len PM, Minohara S, et al. Breathing-synchronized radiotherapy program at the University of California Davis Cancer Center. Med Phys. 2000;27:346-353.
72 Vedam SS, Keall PJ, Kini VR, et al. Determining parameters for respiration-gated radiotherapy. Med Phys. 2001;28:2139-2146.
73 Mageras GS, Yorke E, Rosenzweig K, et al. Fluoroscopic evaluation of diaphragmatic motion reduction with a respiratory gated radiotherapy system. J Applied Clin Med Phys. 2001;2:191-200.
74 Ford EC, Mageras GS, Yorke E, et al. Evaluation of respiratory movement during gated radiotherapy using film and electronic portal imaging. Int J Radiat Oncol Biol Phys. 2002;52:522-531.
75 Kini VR, Vedam SS, Keall PJ, et al. Patient training in respiratory-gated radiotherapy. Med Dosim. 2003;28:7-11.
76 Vedam SS, Kini VR, Keall PJ, et al. Quantifying the predictability of diaphragm motion during respiration with a noninvasive external marker. Med Phys. 2003;30:505-513.
77 Wagman R, Yorke E, Giraud P, et al. Reproducibility of organ position with respiratory gating for liver tumors: use in dose-escalation. Int J Radiat Oncol Biol Phys. 2003;55:659-668.
78 Mageras GS, Yorke E. Deep inspiration breath hold and respiratory gating strategies for reducing organ motion in radiation treatment. Semin Radiat Oncol. 2004;14:65-75.
79 Kitamura K, Shirato H, Onimaru R, et al. Feasibility study of hypofractionated gated irradiation using a real-time tumor-tracking radiation therapy system for malignant liver tumors. Int J Radiat Oncol Biol Phys. 2002;54:125-126.
80 Kitamura K, Shirato H, Seppenwoolde Y, et al. Three-dimensional intrafractional movement of prostate measured during real-time tumor-tracking radiotherapy in supine and prone treatment positions. Int J Radiat Oncol Biol Phys. 2002;53:1117-1123.
81 Kitamura K, Shirato H, Seppenwoolde Y, et al. Tumor location, cirrhosis, and surgical history contribute to tumor movement in the liver, as measured during stereotactic irradiation using a real-time tumor-tracking radiotherapy system. Int J Radiat Oncol Biol Phys. 2003;56:221-228.
82 Kitamura K, Shirato H, Shimizu S, et al. Registration accuracy and possible migration of internal fiducial gold marker implanted in prostate and liver treated with real-time tumor-tracking radiation therapy (RTRT). Radiother Oncol. 2002;62:275-281.
83 Seppenwoolde Y, Shirato H, Kitamura K, et al. Precise and real-time measurement of 3D tumor motion in lung due to breathing and heartbeat, measured during radiotherapy. Int J Radiat Oncol Biol Phys. 2002;53:822-834.
84 Shimizu S, Shirato H, Kitamura K, et al. Fluoroscopic real-time tumor-tracking radiation treatment (RTRT) can reduce internal margin (IM) and set-up margin (SM) of planning target volume (PTV) for lung tumors. Int J Radiat Oncol Biol Phys. 2000;48:166-167.
85 Shimizu S, Shirato H, Kitamura K, et al. Use of an implanted marker and real-time tracking of the marker for the positioning of prostate and bladder cancers. Int J Radiat Oncol Biol Phys. 2000;48:1591-1597.
86 Shimizu S, Shirato H, Ogura S, et al. Detection of lung tumor movement in real-time tumor-tracking radiotherapy. Int J Radiat Oncol Biol Phys. 2001;51:304-310.
87 Shirato H, Harada T, Harabayashi T, et al. Feasibility of insertion/implantation of 2.0-mm-diameter gold internal fiducial markers for precise setup and real-time tumor tracking in radiotherapy. Int J Radiat Oncol Biol Phys. 2003;56:240-247.
88 Shirato H, Shimizu S, Kunieda T, et al. Physical aspects of a real-time tumor-tracking system for gated radiotherapy. Int J Radiat Oncol Biol Phys. 2000;48:1187-1195.
89 Shirato H, Shimizu S, Shimizu T, et al. Real-time tumour-tracking radiotherapy. Lancet. 1999;353:1331-1332.
90 Lax I, Blomgren H, Naslund I, et al. Stereotactic radiotherapy of malignancies in the abdomen. Methodological aspects. Acta Oncol. 1994;33:677-683.
91 Blomgren H, Lax I, Naslund I, et al. Stereotactic high dose fraction radiation therapy of extracranial tumors using an accelerator. Clinical experience of the first thirty-one patients. Acta Oncol. 1995;34:861-870.
92 Lax I. Target dose versus extratarget dose in stereotactic radiosurgery. Acta Oncol. 1993;32:453-457.
93 Negoro Y, Nagata Y, Aoki T, et al. The effectiveness of an immobilization device in conformal radiotherapy for lung tumor: reduction of respiratory tumor movement and evaluation of the daily setup accuracy. Int J Radiat Oncol Biol Phys. 2001;50:889-898.
94 Wulf J, Hadinger U, Oppitz U, et al. Stereotactic radiotherapy of extracranial targets: CT-simulation and accuracy of treatment in the stereotactic body frame. Radiother Oncol. 2000;57:225-236.
95 Timmerman R, Papiez L, McGarry R, et al. Extracranial stereotactic radioablation: results of a phase I study in medically inoperable stage I non-small cell lung cancer. Chest. 2003;124:1946-1955.
96 Papiez L, Timmerman R, DesRosiers C, et al. Extracranial stereotactic radioablation: physical principles. Acta Oncol. 2003;42:882-894.
97 Herfarth KK, Debus J, Lohr F, et al. Extracranial stereotactic radiation therapy: set-up accuracy of patients treated for liver metastases. Int J Radiat Oncol Biol Phys. 2000;46:329-335.
98 Lohr F, Debus J, Frank C, et al. Noninvasive patient fixation for extracranial stereotactic radiotherapy. Int J Radiat Oncol Biol Phys. 1999;45:521-527.
99 McGarry RC, Papiez L, Williams M, et al. Stereotactic body radiation therapy of early-stage non-small-cell lung carcinoma: Phase I study. Int J Radiat Oncol Biol Phys. 2005;63:1010-1015.
100 Timmerman R, Papiez L, Suntharalingam M. Extracranial stereotactic radiation delivery: Expansion of technology beyond the brain. Technol Cancer Res Treat. 2003;2:153-160.
101 Smith RP, Bloch P, Harris EE, et al. Analysis of interfraction and intrafraction variation during tangential breast irradiation with an electronic portal imaging device. Int J Radiat Oncol Biol Phys. 2005;62:373-378.
102 Korreman SS, Pedersen AN, Nottrup TJ, et al. Breathing adapted radiotherapy for breast cancer: comparison of free breathing gating with the breath-hold technique. Radiother Oncol. 2005;76:311-318.
103 Lu HM, Cash E, Chen MH, et al. Reduction of cardiac volume in left-breast treatment fields by respiratory maneuvers: a CT study. Int J Radiat Oncol Biol Phys. 2000;47:895-904.
104 Pedersen AN, Korreman S, Nystrom H, et al. Breathing adapted radiotherapy of breast cancer: reduction of cardiac and pulmonary doses using voluntary inspiration breath-hold. Radiother Oncol. 2004;72:53-60.
105 Remouchamps VM, Letts N, Vicini FA, et al. Initial clinical experience with moderate deep-inspiration breath hold using an active breathing control device in the treatment of patients with left-sided breast cancer using external beam radiation therapy. Int J Radiat Oncol Biol Phys. 2003;56:704-715.
106 Remouchamps VM, Vicini FA, Sharpe MB, et al. Significant reductions in heart and lung doses using deep inspiration breath hold with active breathing control and intensity-modulated radiation therapy for patients treated with locoregional breast irradiation. Int J Radiat Oncol Biol Phys. 2003;55:392-406.
107 Sixel KE, Aznar MC, Ung YC. Deep inspiration breath hold to reduce irradiated heart volume in breast cancer patients. Int J Radiat Oncol Biol Phys. 2001;49:199-204.
108 Keall PJ, Mageras GS, Balter JM, et al. The management of respiratory motion in radiation oncology report of AAPM Task Group 76. Med Phys. 2006;33:3874-3900.
109 Bortfeld T, Chen GT. Special issue: Intrafraction organ motion and its management. Sem Radiat Oncol. 14(1), 2004.
110 Raaymakers BW, Raaijmakers AJ, Kotte AN, et al. Integrating a MRI scanner with a 6 MV radiotherapy accelerator: dose deposition in a transverse magnetic field. Phys Med Biol. 2004;49:4109-4118.
111 Lagendijk JJ, Raaymakers BW, Raaijmakers AJ, et al. MRI/linac integration. Radiother Oncol. 2008;86:25-29.
112 Raaijmakers AJ, Raaymakers BW, Lagendijk JJ. Experimental verification of magnetic field dose effects for the MRI-accelerator. Phys Med Biol. 2007;52:4283-4291.
113 Kirkby C, Stanescu T, Rathee S, et al. Patient dosimetry for hybrid MRI-radiotherapy systems. Med Phys. 2008;35:1019-1027.
114 Hsu A, Miller NR, Evans PM, et al. Feasibility of using ultrasound for real-time tracking during radiotherapy. Med Phys. 2005;32:1500-1512.
115 Wu J, Dandekar O, Nazareth D, et al. Effect of ultrasound probe on dose delivery during real-time ultrasound-guided tumor tracking. Conf Proc IEEE Eng Med Biol Soc. 2006;1:3799-3802.
116 Sawada A, Yoda K, Kokubo M, et al. A technique for noninvasive respiratory gated radiation treatment system based on a real time 3D ultrasound image correlation: A phantom study. Med Phys. 2004;31:245-250.
117 Fuss M, Boda-Heggemann J, Papanikolau N, et al. Image-guidance for stereotactic body radiation therapy. Med Dosim. 2007;32:102-110.
118 Berbeco RI, Jiang SB, Sharp GC, et al. Integrated radiotherapy imaging system (IRIS): Design considerations of tumour tracking with linac gantry-mounted diagnostic x-ray systems with flat-panel detectors. Phys Med Biol. 2004;49:243-255.
119 Cho B, Suh Y, Dieterich S, et al. A monoscopic method for real-time tumour tracking using combined occasional x-ray imaging and continuous respiratory monitoring. Phys Med Biol. 2008;53:2837-2855.
120 Murphy MJ. Tracking moving organs in real time. Semin Radiat Oncol. 2004;14:91-100.
121 Schweikard A, Shiomi H, Adler J. Respiration tracking in radiosurgery. Med Phys. 2004;31:2738-2741.
122 Willoughby TR, Kupelian PA, Pouliot J, et al. Target localization and real-time tracking using the Calypso 4D localization system in patients with localized prostate cancer. Int J Radiat Oncol Biol Phys. 2006;65:528-534.
123 Balter JM, Wright JN, Newell LJ, et al. Accuracy of a wireless localization system for radiotherapy. Int J Radiat Oncol Biol Phys. 2005;61:933-937.
124 Kupelian P, Willoughby T, Mahadevan A, et al. Multi-institutional clinical experience with the Calypso System in localization and continuous, real-time monitoring of the prostate gland during external radiotherapy. Int J Radiat Oncol Biol Phys. 2007;67:1088-1098.
125 Ozhasoglu C, Murphy MJ, Glosser G, et al: Real-time tracking of the tumor volume in precision radiotherapy and body radiosurgery—A novel approach to compensate for respiratory motion, Paper presented at the Computer Assisted Radiology and Surgery Conference, San Francisco, California, 2000.
126 Seppenwoolde Y, Berbeco RI, Nishioka S, et al. Accuracy of tumor motion compensation algorithm from a robotic respiratory tracking system: A simulation study. Med Phys. 2007;34:2774-2784.
127 McClelland JR, Webb S, McQuaid D, et al. Tracking “differential organ motion” with a “breathing” multileaf collimator: magnitude of problem assessed using 4D CT data and a motion-compensation strategy. Phys Med Biol. 2007;52:4805-4826.
128 McQuaid D, Webb S. IMRT delivery to a moving target by dynamic MLC tracking: delivery for targets moving in two dimensions in the beam’s eye view. Phys Med Biol. 2006;51:4819-4839.
129 Webb S, Binnie DM. A strategy to minimize errors from differential intrafraction organ motion using a single configuration for a “breathing” multileaf collimator. Phys Med Biol. 2006;51:4517-4531.
130 Webb S. Quantification of the fluence error in the motion-compensated dynamic MLC (DMLC) technique for delivering intensity-modulated radiotherapy (IMRT). Phys Med Biol. 2006;51:L17-21.
131 Papiez L, Rangaraj D. DMLC leaf-pair optimal control for mobile, deforming target. Med Phys. 2005;32:275-285.
132 Papiez L, Rangaraj D, Keall P. Real-time DMLC IMRT delivery for mobile and deforming targets. Med Phys. 2005;32:3037-3048.
133 Rangaraj D, Papiez L. Synchronized delivery of DMLC intensity modulated radiation therapy for stationary and moving targets. Med Phys. 2005;32:1802-1817.
134 McMahon R, Papiez L, Rangaraj D. Dynamic-MLC leaf control utilizing on-flight intensity calculations: a robust method for real-time IMRT delivery over moving rigid targets. Med Phys. 2007;34:3211-3223.
135 Keall PJ, Cattell H, Pokhrel D, et al. Geometric accuracy of a real-time target tracking system with dynamic multileaf collimator tracking system. Int J Radiat Oncol Biol Phys. 2006;65:1579-1584.
136 Keall PJ, Kini VR, Vedam SS, et al. Motion adaptive x-ray therapy: a feasibility study. Phys Med Biol. 2001;46:1-10.
137 Neicu T, Shirato H, Seppenwoolde Y, et al. Synchronized moving aperture radiation therapy (SMART): average tumour trajectory for lung patients. Phys Med Biol. 2003;48:587-598.
138 Sawant A, Venkat R, Srivastava V, et al. Management of three-dimensional intrafraction motion through real-time DMLC tracking. Med Phys. 2008;35:2050-2061.
139 Zimmerman J, Korreman S, Persson G, et al. DMLC motion tracking of moving targets for intensity modulated arc therapy treatment—a feasibility study. Acta Oncol. 2008:1-6.
140 D’Souza WD, McAvoy TJ. An analysis of the treatment couch and control system dynamics for respiration-induced motion compensation. Med Phys. 2006;33:4701-4709.
141 D’Souza WD, Naqvi SA, Yu CX. Real-time intra-fraction-motion tracking using the treatment couch: a feasibility study. Phys Med Biol. 2005;50:4021-4033.
142 Qiu P, D’Souza WD, McAvoy TJ, et al. Inferential modeling and predictive feedback control in real-time motion compensation using the treatment couch during radiotherapy. Phys Med Biol. 2007;52:5831-5854.
143 Wilbert J, Meyer J, Baier K, et al. Tumor tracking and motion compensation with an adaptive tumor tracking system (ATTS): system description and prototype testing. Med Phys. 2008;35:3911-3921.
144 Kamino Y, Takayama K, Kokubo M, et al. Development of a four-dimensional image-guided radiotherapy system with a gimbaled x-ray head. Int J Radiat Oncol Biol Phys. 2006;66:271-278.
145 Bert C, Saito N, Schmidt A, et al. Target motion tracking with a scanned particle beam. Med Phys. 2007;34:4768-4771.
146 Grozinger SO, Rietzel E, Li Q, et al. Simulations to design an online motion compensation system for scanned particle beams. Phys Med Biol. 2006;51:3517-3531.