Chapter 26 Hypersensitivity (Type IV)
• DTH reflects the presence of antigen-specific T cell-mediated inflammation.
• There are three variants of type IV hypersensitivity reaction – contact, tuberculin, and granulomatous.
• Contact hypersensitivity occurs at the site of contact with an allergen. Sensitization occurs when skin dendritic cells internalize and process epicutaneously applied hapten and migrate to the draining lymph nodes where they activate antigen-specific T cells. On re-exposure to antigen, cytokines produced by skin cells (e.g. keratinocytes, Langerhans’ cells), recruit antigen-specific, and also non-specific T cells, and macrophages.
• Tuberculin-type hypersensitivity is induced by CD4 T cell responses to soluble antigens from a variety of organisms. It is useful as a diagnostic test to detect infection with a number of infectious agents.
• Granulomatous hypersensitivity is clinically the most important form of type IV hypersensitivity. Persistence of antigen leads to chronic T cell activation, differentiation of macrophages into epithelioid cells, and their fusion to form giant cells. This granulomatous reaction results in tissue pathology. Granuloma formation is driven by T cell activation of macrophages, and is dependent on TNF. Inhibition of TNF leads to breakdown in granulomas.
• Many chronic diseases manifest type IV granulomatous hypersensitivity. These include tuberculosis, leprosy, schistosomiasis, sarcoidosis, and Crohn’s disease.
Delayed hypersensitivity
For example, the late-phase IgE-mediated reaction may peak 12–24 hours after contact with allergen, and TH2 cells and eosinophils contribute to the inflammation as well as IgE (see Chapter 23).
There are three variants of type IV hypersensitivity reaction
Three variants of type IV hypersensitivity reaction are recognized (Fig. 26.1):
• contact hypersensitivity and tuberculin-type hypersensitivity both occur within 72 hours of re-exposure to antigen;
• granulomatous hypersensitivity reactions develop over a period of 21–28 days – the granulomas are formed by the aggregation of macrophages and lymphocytes and may persist for weeks – this is the most important type of type IV hypersensitivity response for producing clinical consequences.
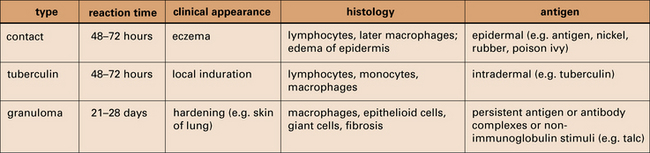
Fig. 26.1 Delayed hypersensitivity reactions
The characteristics of type IV reactions comparing contact, tuberculin, and granulomatous reactions.
Contact hypersensitivity
Contact hypersensitivity is characterized by an eczematous skin reaction at the site of contact with an allergen (Fig. 26.2). Sensitizing agents for humans include metal ions, such as nickel and chromium, many industrial chemicals including those in rubber and leather and natural products present in dyes, drugs, fragrances and plants, such as pentadecacatechol, the sensitizing chemical in poison ivy. This is distinct from the non-immune-mediated inflammatory response to irritants.
Sensitizing agents behave as haptens. Haptens are:
• low molecular weight chemicals (< 1 kDa) that are not immunogenic by themselves
• lipophilic and penetrate the epidermis and dermis where they bind covalently to cysteine or lysine residues in self proteins to form new antigenic determinants.
• metal ions, which chelate with self-peptides in the groove of MHC class II.
A contact hypersensitivity reaction has two stages – sensitization and elicitation
Dendritic cells and keratinocytes have key roles in the sensitization phase
Antigen presenting cells (APC) in the skin include Langerhans’ cell (LCs), located in the suprabasal epidermis, and dermal dendritic cells (dDCs). Contact hypersensitivity is primarily an epidermal reaction, and epidermal LCs were considered to be the APC responsible for initiating contact sensitivity (Fig. 26.3). More recent studies have established that dDCs are essential for stimulating hapten-specific T cells.
Langerhans’ cells (see Chapter 2) are specialized DCs which extend dendritic processes throughout the epidermis, allowing them to sample environmental antigens. LCs express MHC class II, CD1 and the C-type lectin, langerin (CD207), which is responsible for the development of Birbeck granules, the cell membrane-derived organelle characteristic of LCs (see Fig. 26.3). The majority of dermal DCs are Langerin−, but there is a small population of Langerin+ dDCs, which are distinct from LCs, but also migrate rapidly to draining lymph nodes on exposure to sensitizers and activate hapten-specific CD8+ T cells. Both LCs and dDCs take up hapten-modified proteins by micropinocytosis but they also absorb lipid-soluble haptens, which modify cytoplasmic proteins. Under the influence of IL-1 and TNF secreted by keratinocytes and other cells, these DCs undergo maturation and increase expression of MHC and co-stimulatory molecules. Both LCs and dDCs are inactivated by ultraviolet B, which can therefore prevent or alleviate the effects of contact hypersensitivity.
Keratinocytes produce cytokines important to the contact hypersensitivity response
Activated keratinocytes produce a wide range of cytokines, including:
• TNF, IL-1, and granulocyte–macrophage colony stimulating factor (GM–CSF), which activate LCs and dDCs;
• IL-3 which activates LCs and co-stimulates T cell proliferative responses, recruits mast cells, and induces secretion of immunosuppressive cytokines, such as IL-10 and transforming growth factor-β (TGFβ). These dampen the immune response and may induce clonal anergy or immunological unresponsiveness in TH1 cells.
Sensitization stimulates a population of memory T cells
MHC class I-restricted CD8+ T cells are important in contact hypersensitivity responses in humans and mice and are the major effector cells for many allergens. For example, lipid-soluble urushiol from poison ivy enters the cytoplasm of APCs and haptened cytoplasmic proteins are processed through the MHC class I pathway, leading to the activation of allergen-specific CD8+ T cells. Hapten-specific CD4 T cells are also activated hapten–peptide conjugates in association with MHC class II molecules and become effector/memory CD4+ T cells, which contribute to the skin inflammation, or regulatory CD4+ T cells (Fig. 26.4).
Q. What effect will loss of CCR7 and CD62L have on T cell function?
A. CD62L promotes adhesion of lymphocytes to high endothelial venules and CCR7 allows the cells to respond to CCL21 expressed in secondary lymphoid tissues (see Fig. 6.15). Hence cells lacking these receptors will lose their propensity to traffic into lymph tissues.
Elicitation involves recruitment of CD4+ and CD8+ lymphocytes and monocytes
The application of a contact allergen leads to:
• rapid expression of proinflammatory cytokines; and
• recruitment of effector T cells and monocytes to the site (Fig. 26.5).
TNF and IL-1 are potent inducers of endothelial cell adhesion molecules, including:
The earliest histological change, seen after 4–8 hours, is the appearance of mononuclear cells around blood vessels. Macrophages and lymphocytes invade the dermis and epidermis, peaking at 48–72 hours (Fig. 26.7).
Suppression of the inflammatory reaction is mediated by multiple mechanisms
The reaction to cutaneous application of sensitizer wanes after 48–72 hours. This is due to the removal of antigenic stimulus following degradation of the hapten–conjugate and a variety of inhibitory mechanisms (see Fig. 26.6) including:
• keratinocytes, dermal mast cells and macrophages secrete the anti-inflammatory cytokines, IL-10 and TGFβ, and the prostaglandin PGE, which inhibit T cell proliferation, cytokine production and inflammation;
• FoxP3+ CD4+ regulatory T cells and IL-10 secreting TH1 cells directly inhibit activation of effector T cells;
• external factors, such as UV light, also may inhibit the expression of contact sensitivity.
Tuberculin-type hypersensitivity
Soluble antigens from other organisms, including Mycobacterium leprae and Leishmania tropica, induce similar Tuberculin-type hypersensitivity reactions in sensitized people. The skin reaction is frequently used to test for T cell-mediated responses to the organisms following previous exposure (Fig. 26.8).
The tuberculin skin test reaction involves monocytes and lymphocytes
Monocytes constitute 80–90% of the total cellular infiltrate. Both infiltrating lymphocytes and macrophages express MHC class II molecules, and this increases the efficiency of activated macrophages as APCs. CD1+ DCs also are present at 24–48 hours. Overlying keratinocytes express HLA-DR molecules 48–96 hours after the appearance of the lymphocytic infiltrate. These events are summarized in Figure 26.9.
Granulomatous hypersensitivity
Epithelioid cells and giant cells are typical of granulomatous hypersensitivity
Epithelioid cells are large and flattened with increased endoplasmic reticulum (Fig. 26.10). They:
• are derived from activated macrophages under the chronic stimulation of cytokines;
• continue to secrete TNF and thus potentiate continuing inflammation.
Giant cells are formed when epithelioid cells fuse to form multinucleate giant cells (Fig. 26.11), sometimes referred to as Langhans’ giant cells (not to be confused with the Langerhans’ cell discussed earlier). Giant cells have several nuclei at the periphery of the cell. There is little endoplasmic reticulum, and the mitochondria and lysosomes appear to be undergoing degeneration. The giant cell may therefore be a terminal differentiation stage of the monocyte/macrophage line.
A granuloma contains epithelioid cells, macrophages, and lymphocytes
The macrophage/epithelioid core is surrounded by a cuff of lymphocytes, and there may also be considerable fibrosis (deposition of collagen fibers) caused by proliferation of fibroblasts and increased collagen synthesis. An example of a granulomatous reaction is the delayed Mitsuda reaction to dead M. leprae (see Fig. 26.11).
The three types of delayed hypersensitivity are summarized in Figure 26.1.
Cellular reactions in type IV hypersensitivity
T cells bearing αβ TCRs are essential
Sensitized αβ T cells, stimulated with the appropriate antigen and APCs, undergo lymphoblastoid transformation before cell division (Fig. 26.12). This forms the basis of the lymphocyte stimulation test as a measure of T cell function. Lymphocyte stimulation is accompanied by DNA synthesis and this can be measured by assaying the uptake of radiolabeled thymidine, a nucleoside required for DNA synthesis. Lymphocytes from a patient are stimulated in culture with the suspect antigen to determine whether it induces proliferation. It is important to stress that this is a test for T cell memory only, and does not necessarily imply the presence of protective immunity.
IFNγ is required for granuloma formation in humans
The role of individual cytokines can be analyzed in gene knockout mice deficient for a single cytokine. For example, IFNγ gene knockout mice are unable to activate macrophages and control infection with M. tuberculosis (Fig. 26.13).
TNF and lymphotoxin-α are essential for granuloma formation during mycobacterial infections
TNF and the related cytokine, lymphotoxin-α, are both essential for the formation of granulomas during mycobacterial infections (Fig. 26.14), and act in part through the regulation of chemokine production.
Both macrophage- and T cell-derived TNF contribute to this process, but within granulomas activated macrophages become the major source of TNF, driving the differentiation of macrophages into epithelioid cells and the fusion of epithelioid cells to form giant cells (Figs 26.14 and 26.15). The maintenance of granulomas is also dependent on TNF. Consequently, inhibition of TNF activity suppresses the granulomatous inflammation in Crohn’s disease and sarcoidosis.
Granulomatous reactions in chronic diseases
The immune response in leprosy varies greatly between individuals
• in tuberculoid leprosy, the skin may have a few well-defined hypopigmented patches, which show an intense lymphocytic and epithelioid cell infiltrate and no microorganisms;
• by contrast, the polar reaction of lepromatous leprosy shows multiple confluent skin lesions characterized by numerous bacilli, ‘foamy’ macrophages, and a paucity of lymphocytes;
• borderline leprosy has characteristics of both tuberculoid and lepromatous leprosy (Fig. 26.16).
The borderline leprosy reaction is a dramatic example of delayed hypersensitivity. Borderline reactions occur either spontaneously or following drug treatment. In these reactions, hypopigmented skin lesions containing M. leprae become swollen and inflamed (Fig. 26.17) because the patient is now able to mount a T cell response to the mycobacteria resulting in a delayed-type hypersensitivity reaction. The histological appearance shows a more tuberculoid pattern with an infiltrate of IFNγ-secreting lymphocytes. The process may occur in peripheral nerves, where Schwann cells contain M. leprae; this is the most important cause of nerve destruction in this disease. The lesion in borderline leprosy is typical of granulomatous hypersensitivity (see Fig. 26.17).
Granulomatous reactions are necessary to control tuberculosis
• controlling the bacterial growth; and
• causing tissue damage in infected organs. In those who progress to clinical tuberculosis, granulomatous reactions erode airways leading to cavitation in the lung and spread of bacteria. The reactions are frequently accompanied by extensive fibrosis and the lesions are visible in the chest radiographs of affected patients (Fig. 26.w1 ).
The histological appearance of the lesion is typical of a granulomatous reaction, with central caseous (cheesy) necrosis (Fig. 26.18). This is surrounded by an area of epithelioid cells with a few giant cells. Mononuclear cell infiltration occurs around the edge.
Granulomas surround the parasite ova in schistosomiasis
In schistosomiasis, which is caused by parasitic trematode worms (schistosomes), the host becomes sensitized to the eggs of the worms, leading to a typical granulomatous reaction in the parasitized tissue mediated essentially by TH2 cells (Fig. 26.19; see also Chapter 15). In this case the cytokines IL-5 and IL-13 are responsible for the recruitment of eosinophils and the formation of the granulomas around the ova. When the eggs have been deposited in the liver, the subsequent IL-13-dependent fibrosis causes hepatic scarring and portal hypertension.
The cause of sarcoidosis is unknown
Sarcoidosis is a chronic disease of unknown etiology in which activated macrophages and non-caseating granuloma accumulate in many tissues, frequently accompanied by fibrosis (Fig. 26.20). The disease particularly affects lymphoid tissue and the lungs, as well as bone, nervous tissue, and skin. Enlarged lymph nodes may be detected in chest radiographs of affected patients (Fig. 26.w2 ). No infectious agent has been isolated, though mycobacteria have been implicated because of the similarities in the pathology.
The cause of Crohn’s disease is unknown
Crohn’s disease is a chronic inflammatory disease of the ileum and colon, in which lymphocytes and macrophages accumulate in all layers of the bowel. The granulomatous reaction and fibrosis cause stricture of the bowel and penetrating fistulas into other organs (see Fig. 7.17). Although the antigens initiating this granulomatous reaction are unknown, there appears to be defect in inflammasome-mediated intracellular signaling responses to bacterial products in Crohn’s disease. This may result an excessive T cell-driven immune response to microbial antigens in genetically predisposed individuals.
Critical thinking: A hypersensitivity type IV reaction (see p. 444 for explanations)
1. What cell types make up the granulomas in the lymph node and what cytokines are involved in their formation?
2. What is the pathology at the site of the skin testing and how does it differ from that in the lymph node?
3. What type of lymphocyte is responsible for the skin test reactivity?
4. What other conditions cause granulomas in lymph nodes and how are they diagnosed?
5. When the family members are tested, the boy’s 5-year-old brother is found to have a positive tuberculin reaction (18 mm at 48 hours), but he is well with a normal chest radiograph. What does this result indicate about his immune responses and what is its significance?
Ananworanich J., Shearer W.T. Delayed-type hypersensitivity skin testing. In Manual of Clinical Laboratory Immunology, 6th edn, Washington: ASM Press; 2002:212–219.
Askenase P.W. Yes T cells, but three different T cells (αβ, γδ and NK T cells) and also B-1 cells mediate contact sensitivity. Clin Exp Immunol. 2001;125:345–350.
Baughman R.P., Lower E.E., du Bois R.M. Sarcoidosis. Lancet. 2003;361:1111–1118.
Bean A.G.D., Roach D.R., Briscoe H., et al. Structural deficiencies in granuloma formation in tumor necrosis factor gene-targeted mice underlie the heightened susceptibility to aerosol Mycobacterium tuberculosis infection which is not compensated for by lymphotoxin. J Immunol. 1999;162:3504–3511.
Brand S. Crohn’s disease: TH1, TH17 or both? The change of a paradigm: new immunological and genetic insights implicate TH17 cells in the pathogenesis of Crohn’s disease. Gut. 2009;58:1152–1167.
Britton W.J., Lockwood D.N. Leprosy. Lancet. 2004;363:1209–1219.
Casanova J.-L., Abel L. Genetic dissection of immunity to mycobacteria: the human model. Annu Rev Immunol. 2002;40:581–620.
Cavani A., De Luca A. Allergic contact dermatitis: novel mechanisms and therapeutic perspectives. Curr Drug Metabol. 2010;11:228–233.
Cho J.H. The genetics and immunopathogenesis of inflammatory bowel disease. Nat Rev Immunol. 2008;8:458–466.
Cooper A.M. Cell-mediated immune responses in tuberculosis. Ann Rev Immunol. 2009;27:393–422.
Daniel H., Present M.D., Rutgeerts P., et al. Infliximab for the treatment of fistulas in patients with Crohn’s disease. N Engl J Med. 1999;18:1398–1405.
Flynn J.L., Chan J., Triebold K.J., et al. An essential role for interferon-γ in resistance to Mycobacterium tuberculosis infection. J Exp Med. 1993;178:2249–2254.
Hagge D.A., Saunders B.M., Ebenezer G.J., et al. Lymphotoxin-alpha and TNF have essential but independent roles in the evolution of the granulomatous response in experimental leprosy. Am J Pathol. 2009;174:1379–1389.
Igyarto B.Z., Kaplan D.H. The evolving function of Langerhans cells in adaptive skin immunity. Immunol Cell Biol. 2010;88:361–365.
Kalish R.S., Wood J.A., LaPorte A. Processing of urushiol (poison ivy) hapten by both endogenous and exogenous pathways for presentation to T cells in vitro. J Clin Invest. 1994;93:2039–2047.
Kaplan D.H. In vivo function of Langerhans cells and dermal dendritic cells. Trends Immunol. 2010;27:446–451.
Kindler V., Sappino A.-P., Gran G.E., et al. The inducing role of tumour necrosis factor in the development of bactericidal granulomas during BCG infection. Cell. 1989;56:731–740.
Klimas N. Delayed hypersensitivity skin testing. In Manual of clinical laboratory immunology, 5th edn, Washington: ASM Press; 1997:276–280.
Martin S.F., Esser P.R., Schmucker S., et al. T-cell recognition of chemicals, protein allergens and drugs: towards the development of in vitro assays. Cell Mol Life Sci. 2010;67:4171–4184.
Roach D.R., Briscoe H., Saunders B., et al. Secreted lymphotoxin-alpha is essential for the control of intracellular bacterial infection. J Exp Med. 2001;193:239–246.
Roach D.R., Bean A.G.D., Demangel C., et al. Tumor necrosis factor regulates chemokine induction essential for cell recruitment, granuloma formation and clearance of mycobacterial infection. J Immunol. 2002;168:4620–4628.
Salgame P. Host innate and TH1 responses and the bacterial factors that contain Mycobacterium tuberculosis infection. Curr Opin Immunol. 2005;17:374–380.
Saunders B.M., Britton W.J. Life and death in the granuloma: immunopathology of tuberculosis. Immunol Cell Biol. 2007;85:103–111.
Vocanson M., Hennino A., Rozieres A., et al. Effector and regulatory mechanisms in allergic contact dermatitis. Allergy. 2009;64:1699–1714.
Von Andrian U.H., Mackay C.R. T cell function and migration: two sides of the same coin. N Engl J Med. 2000;343:1020–1034.
Wallis R.S., Broder M.S., Wong J.Y., et al. Granulomatous infectious disease associated with tumor necrosis factor. Clin Infect Dis. 2004;38:1261–1265.
Wynn T.A., Thompson R.W., Cheever A.W., Mentink-Kane M.M. Immunopathogenesis of schistosomiasis. Immunol Rev. 2004;201:156–167.
Yamamura M., Uyemura K., Deans R.J., et al. Defining protective immune responses to pathogens: cytokine profiles in leprosy lesions. Science. 1991;254:277–279.