Hypersensitivity Reactions
At the conclusion of this chapter, the reader should be able to:
• Define the terms hypersensitivity, allergy, sensitization, and immunization.
• Identify and explain the three categories of antigens.
• Compare the basic differences among and give examples of types I, II, III, and IV hypersensitivity reactions.
• Describe the etiology, immunologic activity, signs and symptoms, laboratory evaluation, and treatment of type I hypersensitivity reactions.
• Discuss examples of type II hypersensitivity reactions, including laboratory evaluation.
• Describe the mechanism of tissue injury, clinical manifestations, and laboratory testing for type III hypersensitivity reactions.
• Describe the characteristics and laboratory evaluation of type IV hypersensitivity reactions.
• Discuss the acquisition and consequences of latex sensitivity.
• Analyze case studies related to hypersensitivity reactions.
• Correctly answer case study related multiple choice questions.
• Be prepared to participate in a discussion of critical thinking questions.
• Describe the principle, clinical applications, or sources of error of a food allergy test, and the direct antiglobulin test.
Types of Antigens and Reactions
Food Allergies
The NIAID guidelines separate diseases defined as FA that include both IgE-mediated reactions to food (food allergies), non–IgE-mediated reactions to certain foods (e.g., celiac disease), and mixed IgE and non-IgE disorders (Table 26-1).
Table 26-1
Classification of Hypersensitivity Reactions
Parameter | Type of Reaction | |||
I | II | III | IV | |
Reaction | Anaphylactic | Cytotoxic | Immune complex | T cell–dependent |
Antibody | IgE∗ | IgG, possibly other immunoglobulins | Antigen-antibody complexes (IgG, IgM)∗ | None |
Complement involved | No | Yes∗ | Yes∗ | No |
Cells involved | Mast cells, basophils, granules (histamine)∗ | Effector cells (macrophages, polymorphonuclear leukocytes)∗ | Macrophages, mast cells | Antigen-specific T cells |
Cytokines involved | Yes∗ | No | Yes∗ | Yes (T cell cytokines)∗ |
Comparative description | Antibody mediated, immediate | Antibody dependent; complement or cell mediated | Immune complex mediated (immune complex disease) | T cell-mediated, delayed type |
Mechanism of tissue injury | Allergic and anaphylactic reactions | Target cell lysis; cell-mediated cytotoxicity | Immune complex deposition, inflammation | Inflammation, cellular infiltration |
Examples | Anaphylaxis Hay fever Asthma |
Transfusion reactions Hemolytic disease of newborn Thrombocytopenia |
Arthus reaction Serum sickness Systemic lupus erythematosus |
Allergy or infection Contact dermatitis |
Types of Hypersensitivity Reactions
The four types of hypersensitivity reaction (I to IV) are defined by the principal mechanism responsible for a specific cell or tissue injury that occurs during an immune response (Table 26-2). Types I, II, and III reactions are antibody dependent and type IV is cell mediated. Some overlapping occurs among the various types of hypersensitivity reactions, but there are major differences in how each type is diagnosed and treated.
Table 26-2
Mediator | Primary Action |
Histamine | Increases vascular permeability; promotes contraction of smooth muscle |
Leukotrienes | Alter bronchial smooth muscle and enhance effects of histamine on target organs |
Basophil kallikrein | Generates kinins |
Serotonin | Contracts smooth muscle |
Platelet-activating factor | Enhances the release of histamine and serotonin from platelets that affect smooth muscle tone and vascular permeability |
Eosinophil chemotactic factor of anaphylaxis | Attracts eosinophils to area of activity; these cells release secondary mediators that may limit the effects of primary mediators |
Prostaglandins | Affect smooth muscle tone and vascular permeability |
Type I Reactions
Etiology
Several groups of agents cause anaphylactic reactions. The two most common agents are drugs (e.g., systemic penicillin) and insect stings. Insects of the order Hymenoptera (e.g., common hornet, yellow jacket, yellow hornet, paper wasp) are examples of insects causing the most serious reactions. Immune-mediated IgE adverse food reactions (Box 26-1) can be fatal.
Immunologic Activity
Immediate hypersensitivity is the basis of acute allergic reactions caused by molecules released by mast cells when an allergen interacts with membrane-bound IgE (Fig. 26-1). Acute allergic reactions result from the release of preformed granule-associated mediators, membrane-derived lipids, cytokines, and chemokines when an allergen interacts with IgE that is bound to mast cells or basophils by the alpha chain of the high-affinity IgE receptor (FcεRI-α). This antigen receptor also occurs on antigen-presenting cells, where it can facilitate the IgE-dependent trapping and presentation of allergen to T cells.
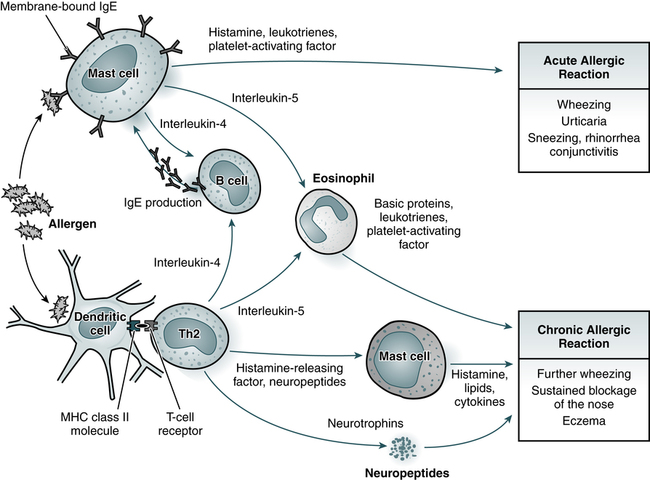
Acute allergic reactions are caused by the antigen-induced release of histamine and lipid mediators from mast cells. In the skin and upper airways, basophils (not shown) may also participate in allergic tissue reactions. Chronic allergic reactions, including the late-phase reaction, may depend on a combination of pathways, including recruitment of eosinophils, liberation of mast cell products by histamine-releasing factors, and neurogenic inflammation involving neurotrophins and neuropeptides. Th2, T-helper cell type 2; MHC, major histocompatibility complex. (Adapted from Kay AB: Allergy and allergic disease, N Engl J Med 344:30–38, 2001.)
Anaphylactic Reaction
1. The offending antigen attaches to the IgE antibody fixed to the surface membrane of mast cells and basophils. Cross-linking of two IgE molecules is necessary to initiate mediator release from mast cells.
2. Activated mast cells and basophils release various mediators.
3. The effects of mediator release produce vascular changes and activation of platelets, eosinophils, neutrophils, and the coagulation cascade.
Signs and Symptoms
Generalized Reaction
A generalized (anaphylactic) reaction is produced by mediators such as cytokines and vasoactive amines (e.g., histamine) from mast cells. Anaphylactic reactions are dramatic and rapid in onset. The physiologic effects of the primary and secondary mediators on the target organs, such as the cardiovascular or respiratory system, gastrointestinal (GI) tract, or the skin, define the signs and symptoms of anaphylaxis. Several important pharmacologically active compounds are discharged from mast cells and basophils during anaphylaxis (see Table 26-2).
Laboratory Evaluation of Allergic Reactions
ImmunoCAP
The U.S. Food and Drug Administration (FDA) has approved ImmunoCAP to provide an in vitro quantitative measurement of IgE in human serum (Fig. 26-2). It is considered to be the gold standard for the analysis of allergen-specific IgE. It is intended for in vitro use as an aid in the clinical diagnosis of IgE-mediated allergic disorders in conjunction with other clinical findings (Table 26-3) .
Table 26-3
Comparison of Tests for Specific IgE
Parameter | Skin Prick Testing | Intradermal Testing | Blood Testing (ImmunoCAP) |
Sensitivity (%) | 93.6 | 60.0 | 87.2 |
Specificity (%) | 80.1 | 32.3 | 90.5 |
Adapted from Choo-Kang LR: Specific IgE testing: objective laboratory evidence supports allergy diagnosis and treatment, Med Lab Observer MLO 38:10–14, 2006.