Host-Microorganism Interactions
1. List the various reservoirs (environments) that facilitate host-microorganism interactions.
2. Define direct versus indirect transmission and provide examples of each.
3. Define and differentiate the interactions between the host and microorganism, including colonization, infection, normal (resident) flora, pathogens, opportunistic pathogens, and nosocomial infection.
4. List and describe the components involved in specific versus nonspecific immune defenses, including inflammation, phagocytosis, antibody production, and cellular responses.
5. Identify elements involved in the two arms of the immune system: humoral and cell-mediated immunity.
6. Provide specific examples of disease prevention strategies, including preventing transmission, controlling reservoirs and minimizing risk of exposure.
7. Differentiate between bacterial endotoxins and exotoxins and provide examples of each.
8. Given a patient history of an infectious process, identify and differentiate a sign versus a symptom.
9. Define and differentiate between an acute infectious process and one that is chronic and/or latent.
Interactions between humans and microorganisms are exceedingly complex and far from being completely understood. What is known about the interactions between these two living entities plays an important role in the practice of diagnostic microbiology and in the management of infectious disease. Understanding these interactions is necessary for establishing methods to reliably isolate specific microorganisms from patient specimens and for developing effective treatment strategies. This chapter provides the framework for understanding the various aspects of host-microorganism interactions. Box 3-1 lists a variety of terms and definitions associated with host-microorganism interactions.
The complex relationships between human hosts and medically relevant microorganisms are best understood by considering the sequential steps in the development of microbial-host associations and the subsequent development of infection and disease. The stages of interaction (Figure 3-1) include (1) the physical encounter between host and microorganism; (2) colonization or survival of the microorganism on an internal (gastrointestinal, respiratory, or genitourinary tract) or external (skin) surface of the host; (3) microbial entry, invasion, and dissemination to deeper tissues and organs of the human body; and (4) resolution or outcome.
The Encounter Between Host and Microorganism
The Human Host’s Perspective
Microbial Reservoirs and Transmission
Humans encounter microorganisms when they enter or are exposed to the same environment in which the microbial agents live or when the infectious agents are brought to the human host by indirect means. The environment, or place of origin, of the infecting agent is referred to as the reservoir. As shown in Figure 3-2, microbial reservoirs include humans, animals, water, food, air, and soil. The human host may acquire microbial agents by various means referred to as the modes of transmission. The mode of transmission is direct when the host directly contacts the microbial reservoir and is indirect when the host encounters the microorganism by an intervening agent of transmission.
The agents of transmission that bring the microorganism from the reservoir to the host may be a living entity, such as an insect, in which case they are called vectors, or they may be a nonliving entity, referred to as a vehicle or fomite. Additionally, some microorganisms may have a single mode of transmission, whereas others may spread by various methods. From a diagnostic microbiology perspective, knowledge about an infectious agent’s mode of transmission is often important for determining optimum specimens for isolation of the organism and for implementing precautions that minimize the risk of laboratory-acquired infections (see Chapters 4 and 80 for more information regarding laboratory safety).
Microorganism Colonization of Host Surfaces
The Host’s Perspective
Once a microbe and the human host are brought into contact, the outcome of the encounter depends on what happens during each step of interaction (see Figure 3-1), beginning with colonization. The human host’s role in microbial colonization, defined as the persistent survival of microorganisms on a surface of the human body, is dictated by the defenses that protect vital internal tissues and organs against microbial invasion. The first defenses are the external and internal body surfaces that are in direct contact with the external environment and are the anatomical regions where the microorganisms will initially come in contact with the human host. These surfaces include:
• Skin (including conjunctival epithelium covering the eye)
• Mucous membranes lining the mouth or oral cavity, the respiratory tract, the gastrointestinal tract, and the genitourinary tract
Skin and Skin Structures
Skin serves as a physical and chemical barrier to microorganisms; its protective characteristics are summarized in Table 3-1 and Figure 3-3. The acellular, outermost layer of the skin, along with the tightly packed cellular layers underneath, provide an impenetrable physical barrier to all microorganisms, unless damaged. Additionally, these layers continuously shed, thus dislodging bacteria that have attached to the outer layers. The skin is also a dry and cool environment; this is incompatible with the growth requirements of many microorganisms, which thrive in a warm, moist environment.
TABLE 3-1
Protective Characteristics of the Skin and Skin Structures
Skin Structure | Protective Activity |
Outer (dermal) layers |
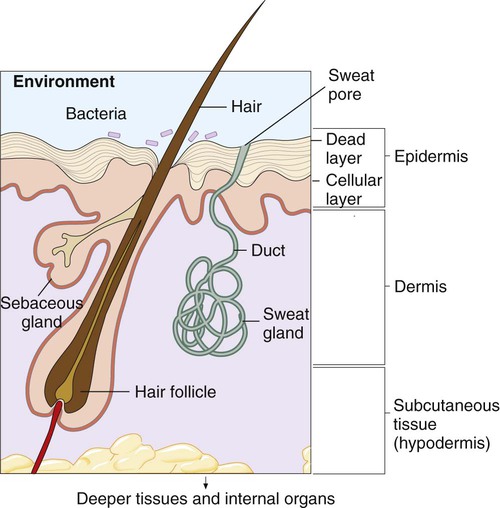
Mucous Membranes
Because cells that line the respiratory tract, gastrointestinal tract, and genitourinary tract are involved in numerous functions besides protection, they are not covered with a hardened, acellular layer as is the skin surface. However, the cells that compose these membranes still exhibit various protective characteristics (Table 3-2 and Figure 3-4).
TABLE 3-2
Protective Characteristics of Mucous Membranes
Mucous Membrane | Protective Activity |
Mucosal cells |
Specific Protective Characteristics.
Besides the general protective properties of mucosal cells, the mucosal linings throughout the body have characteristics specific to each anatomic site (Figure 3-5).
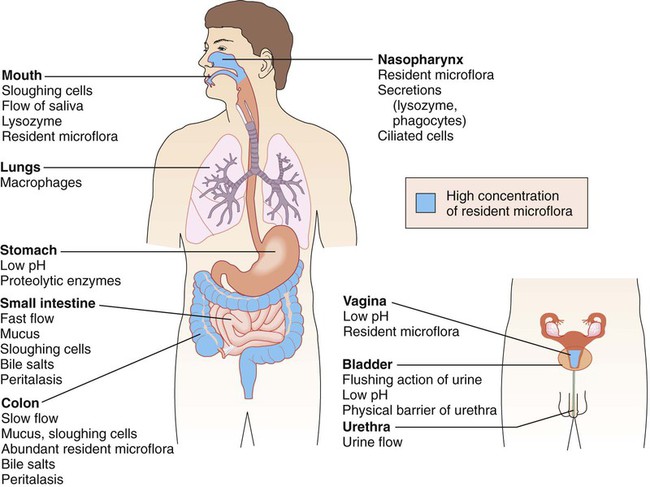
In the upper respiratory tract, nasal hairs keep out large airborne particles that may contain microorganisms. The cough-sneeze reflex significantly contributes to the removal of potentially infective agents. The cells lining the trachea contain cilia (hairlike cellular projections) that move microorganisms trapped in mucus upward and away from the delicate cells of the lungs (see Figure 3-4); this is referred to as the mucociliary escalator. These barriers are so effective that only inhalation of particles smaller than 2 to 3 µm have a chance of reaching the lungs.
The Microorganism’s Perspective
As previously discussed, microorganisms that inhabit many surfaces of the human body (see Figure 3-5) are referred to as colonizers, or normal flora (also referred to as normal microbiota). Some are transient colonizers, because they are able to survive, but do not multiply, on the surface and are frequently shed with the host cells. Others, called resident flora, not only survive but also thrive and multiply; their presence is more persistent.
Microbial Colonization
Colonization may be the last step in the establishment of a long-lasting, mutually beneficial (i.e., commensal), or harmless, relationship between a colonizer and the human host. Alternatively, colonization may be the first step in the process for the development of infection and disease. Whether colonization results in a harmless or damaging infection depends on the characteristics of the host and the microorganism. In either case, successful initial colonization depends on the microorganism’s ability to survive the conditions first encountered on the host surface (Box 3-2).
Besides surviving the host’s physical and chemical conditions, colonization also requires that microorganisms attach and adhere to host surfaces (see Box 3-2). This can be particularly challenging in places such as the mouth and bowel, in which the surfaces are frequently washed with passing fluids. Pili, the rodlike projections of bacterial envelopes, various molecules (e.g., adherence proteins and adhesins), and biochemical complexes (e.g., biofilm) work together to enhance attachment of microorganisms to the host cell surface. Biofilm is discussed in more detail later in this chapter. (For more information concerning the structure and functions of pili, see Chapter 2.)
Microorganism Entry, Invasion, and Dissemination
The Host’s Perspective
Disruption of Surface Barriers
Any situation that disrupts the physical barrier of the skin and mucosa, alters the environmental conditions (e.g., loss of stomach acidity or dryness of skin), changes the functioning of surface cells, or alters the normal flora population can facilitate the penetration of microorganisms past the barriers and into deeper host tissues. Disruptive factors may vary from accidental or intentional (medical) trauma that results in surface destruction to the use of antibiotics that remove normal, protective, colonizing microorganisms (Box 3-3). It is important to note that a number of these factors are related to medical interventions and procedures.
Responses to Microbial Invasion of Deeper Tissues
Nonspecific Responses.
Phagocytes.
Phagocytes are cells that ingest and destroy bacteria and other foreign particles. The two major types of phagocytes are polymorphonuclear leukocytes, also known as PMNs or neutrophils, and macrophages. Phagocytes ingest bacteria by a process known as endocytosis and engulf them in a membrane-lined structure called a phagosome (Figure 3-6). The phagosome is then fused with a second structure, the lysosome. When the lysosome, which contains toxic chemicals and destructive enzymes, combines with the phagosome, the bacteria trapped within the structure, referred to as a phagolysosome, are neutralized and destroyed. This destructive process must be carried out inside membrane-lined structures; otherwise the noxious substances contained within the phagolysosome would destroy the phagocyte itself. This is evident during the course of rampant infections when thousands of phagocytes exhibit “sloppy” ingestion of the microorganisms and toxic substances spill from the cells, damaging the surrounding host tissue. This process is referred to as phagocytosis.
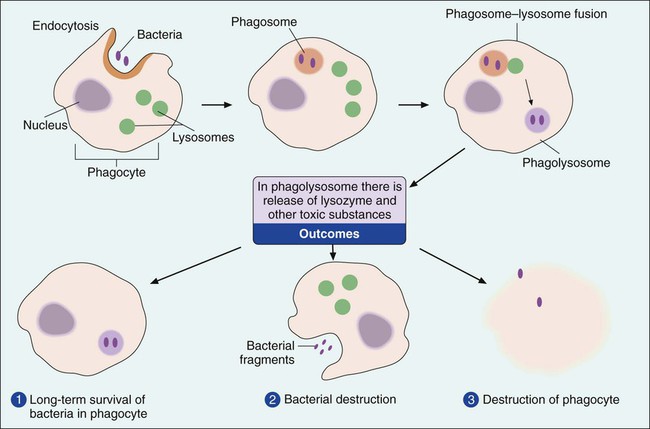
Although both PMNs and macrophages are phagocytes, these cell types differ. PMNs develop in the bone marrow and spend their short lives (usually a day or less) circulating in blood and tissues. Widely dispersed in the body, PMNs usually are the first cells on the scene of bacterial invasion. Macrophages also develop in the bone marrow but first go through a cellular phase in which they are called monocytes. Macrophages circulating in the bloodstream are called monocytes. When deposited in tissue or at a site of infection, monocytes transform into mature macrophages. In the absence of infection, macrophages usually reside in specific organs, such as the spleen, lymph nodes, liver, or lungs, where they live for days to several weeks, awaiting encounters with invading bacteria. In addition to the ingestion and destruction of bacteria, macrophages play an important role in mediating immune system defenses (see Specific Responses—The Immune System later in this chapter).
Inflammation.
Because microbes may survive initial encounters with phagocytes (see Figure 3-6), the inflammatory response plays an extremely important role as a primary mechanism against microbial survival and proliferation in tissues and organs. Inflammation has both cellular and biochemical components that interact in various complex ways (Table 3-3).
TABLE 3-3
Component | Functions |
Phagocytes (polymorphonuclear neutrophils [PMNs], dendritic cells, and macrophages) |
The manifestations of inflammation are evident and familiar to most of us and include the following:
• Swelling—caused by increased flow of fluid and cells to the affected body site
• Redness—results from vasodilation of blood vessels and increased blood flow at the infection site
• Heat—results from increased cellular metabolism and energy production in the affected area
• Pain—due to tissue damage and pressure on nerve endings from increased flow of fluid and cells
On a microscopic level, the presence of phagocytes at the infection site is an important observation in diagnostic microbiology. Microorganisms associated with these host cells are frequently identified as the cause of a particular infection. An overview of inflammation is depicted in Figure 3-7.
Specific Responses—the Immune System
Components of the Immune System
The central molecule of the immune response is the antibody. Antibodies, also referred to as immunoglobulins, are specific glycoproteins produced by plasma cells (activated B cells) in response to the presence of a molecule recognized as foreign to the host (referred to as an antigen). In the case of infectious diseases, antigens are chemicals or toxins secreted by the invading microorganism or components of the organism’s structure and are usually composed of proteins or polysaccharides. Antibodies circulate in the plasma or liquid portion of the host’s blood and are present in secretions such as saliva. These molecules have two active areas: the antigen binding site (Fab region) and the phagocyte and complement binding sites (Fc region) (Figure 3-8).
Five major classes of antibody have been identified: IgG, IgA, IgM, IgD, and IgE. Each class has distinctive molecular configurations. IgM is the largest and first antibody produced when an invading microorganism is initially encountered; production of the most abundant antibody, IgG, follows. IgA is secreted in various body fluids (e.g., saliva and tears) and primarily protects body surfaces lined with mucous membranes. Increased IgE is associated with various parasitic infections and various allergies. IgD is attached to the surface of specific immune system cells and is involved in the regulation of antibody production. As is discussed in Chapter 10, our ability to measure specific antibody production is a valuable tool for the laboratory diagnosis of infectious diseases.
Regarding the cellular components of the immune response, there are three major types of cells: B lymphocytes, T lymphocytes, and natural killer cells (Box 3-4). B lymphocytes originate from stem cells and develop into B cells in the bone marrow before being widely distributed to lymphoid tissues throughout the body. These cells primarily function as antibody producers (plasma cells). T lymphocytes also originate from bone marrow stem cells, but they mature in the thymus and either directly destroy infected cells (cytotoxic T cells) or work with B cells (helper T cells) to regulate antibody production. Natural killer cells are a subset of T cells. There are different types of natural killer cells, with the most prevalent referred to as invariant natural killer T cells (NKT). NKT cells develop in the thymus from the same precursor cells as other T lymphocytes. Each of the three cell types is strategically located in lymphoid tissue throughout the body to maximize the chances of encountering invading microorganisms that the lymphatic system drains from the site of infection.
Two Branches of the Immune System.
The immune system provides immunity that generally can be divided into two branches:
Antibody-mediated immunity is centered on the activities of B cells and the production of antibodies. When B cells encounter a microbial antigen, they become activated and a series of events is initiated. These events are mediated by the activities of helper T cells and the release of cytokines. Cytokines mediate clonal expansion, and the number of B cells capable of recognizing the antigen increases. Cytokines also activate the maturation of B cells into plasma cells that produce antibodies specific for the antigen. The process results in the production of B-memory cells (Figure 3-9). B-memory cells remain quiescent in the body until a second or subsequent exposure to the original antigen occurs. With secondary exposure, the B-memory cells are preprogrammed to produce specific antibodies immediately upon encountering the original antigen.
Antibodies protect the host in a number of ways:
• Helping phagocytes to ingest and kill microorganisms through a coating mechanism referred to as opsonization
• Neutralizing microbial toxins detrimental to host cells and tissues
• Promoting bacterial clumping (agglutination) that facilitates clearing from the infection site
• Inhibiting bacterial motility
• Viral neutralization; blocking the virus from entering the host cell
• Combining with microorganisms to activate the complement system and inflammatory response
Like B cells, T cells must be activated. Activation is accomplished by T-cell interactions with other cells that process microbial antigens and present them on their surface (e.g., macrophages, dendritic cells, and B cells). The responses of activated T cells are very different and depend on the subtype of T cell (Figure 3-10). Activated helper T cells work with B cells for antibody production (see Figure 3-9) and facilitate inflammation by releasing cytokines. Cytotoxic T cells directly interact with and destroy host cells containing microorganisms or other infectious agents, such as viruses. The activated T-cell subset, helper or cytotoxic cells, is controlled by an extremely complex series of biochemical pathways and genetic diversity within the major histocompatibility complex (MHC). MHC molecules are present on cells and form a complex with the antigen to present them to the T cells. The two primary classes of major histocompatibility molecules are MHC I and MHC II. MHC I molecules are located on every nucleated cell in the body and are predominantly responsible for the recognition of endogenous proteins expressed from within the cell. MHC II molecules are located on specialized cell types, including macrophages, dendritic cells, and B cells, for the presentation of extracellular molecules or exogenous proteins.
The Microorganism’s Perspective
Pathogens and Virulence
Because host factors play a role in the development of infectious diseases, the distinction between a pathogenic and nonpathogenic organism or colonizer is not always clear. For example, many organisms that colonize our skin usually do not cause disease (i.e., exhibit low pathogenicity) under normal circumstances. However, when damage to the skin occurs (see Box 3-3) or when the skin is disrupted in some other way, these organisms can gain access to deeper tissues and establish an infection.
Microbial Virulence Factors
Attachment.
Many of the microbial factors that facilitate attachment of pathogens are the same as those used by nonpathogenic colonizers (see Box 3-2). Most pathogenic organisms are not part of the normal microbial flora, and attachment to the host requires that they outcompete colonizers for a place on the body’s surface. Medical interventions, such as the overuse of antimicrobial agents, result in the destruction of the normal flora, creating a competitive advantage for the invading pathogenic organism.
Invasion.
Once surface attachment has been secured, microbial invasion into subsurface tissues and organs (i.e., infection) is accomplished by disruption of the skin and mucosal surfaces by several mechanisms (see Box 3-3) or by the direct action of an organism’s virulence factors. Some microorganisms produce factors that force mucosal surface phagocytes (M cells) to ingest them and then release them unharmed into the tissue below the surface. Other organisms, such as staphylococci and streptococci, are not so subtle. These organisms produce an array of enzymes (e.g., hyaluronidases, nucleases, collagenases) that hydrolyze host proteins and nucleic acids, destroying host cells and tissues. This destruction allows the pathogen to “burrow” through minor openings in the outer surface of the skin and into deeper tissues. Once a pathogen has penetrated the body, it uses a variety of strategies to survive attack by the host’s inflammatory and immune responses. Alternatively, some pathogens cause disease at the site of attachment without further penetration. For example, in diseases such as diphtheria and whooping cough, the bacteria produce toxic substances that destroy surrounding tissues. The organisms generally do not penetrate the mucosal surface they inhabit.
Survival Against Inflammation.
If a pathogen is to survive, the action of phagocytes and the complement components of inflammation must be avoided or controlled (Box 3-5). Some organisms, such as Streptococcus pneumoniae, a common cause of bacterial pneumonia and meningitis, avoid phagocytosis by producing a large capsule that inhibits the phagocytic process. Other pathogens may not be able to avoid phagocytosis but are not effectively destroyed once internalized and are able to survive within phagocytes. This is the case for Mycobacterium tuberculosis, the bacterium that causes tuberculosis. Still other pathogens use toxins and enzymes to attack and destroy phagocytes before the phagocytes attack and destroy them.
Survival Against the Immune System.
Microbial strategies to avoid the defenses of the immune system are outlined in Box 3-6. Again, a pathogen can use more than one strategy to avoid immune-mediated defenses, and microbial survival does not necessarily require devastation of the immune system. The pathogen may merely need to “buy” time to reach a safe area in the body or to be transferred to the next susceptible host. Also, microorganisms can avoid much of the immune response if they do not penetrate the surface layers of the body. This strategy is the hallmark of diseases caused by microbial toxins.
Microbial Toxins.
Endotoxin and exotoxin are the two general types of bacterial toxins (Box 3-7). Endotoxin is a component of the cellular structure of gram-negative bacteria and can have devastating effects on the body’s metabolism, the most serious being endotoxic shock, which often results in death. The effects of exotoxins produced by gram-positive bacteria tend to be more limited and specific than the effects of gram-negative endotoxin. The activities of exotoxins range from enzymes produced by many staphylococci and streptococci that augment bacterial invasion by damaging host tissues and cells to highly specific activities (e.g., diphtheria toxin inhibits protein synthesis, and cholera toxin interferes with host cell signals). Examples of other highly active and specific toxins are those that cause botulism and tetanus by interfering with neuromuscular functions.
Genetics of Virulence: Pathogenicity Islands
Many virulence factors are encoded in genomic regions of pathogens known as pathogenicity islands (PAIs). These are mobile genetic elements that contribute to the change and spread of virulence factors among bacterial populations of a variety of species. These genetic elements are thought to have evolved from lysogenic bacteriophages and plasmids and are spread by horizontal gene transfer (see Chapter 2 for information about bacterial genetics). PAIs are typically comprised of one or more virulence-associated genes and “mobility” genes (i.e., integrases and transposases) that mediate movement between various genetic elements (e.g., plasmids and chromosomes) and among different bacterial strains. In essence, PAIs facilitate the dissemination of virulence capabilities among bacteria in a manner similar to the mechanism diagrammed in Figure 2-10; this also facilitates dissemination of antimicrobial resistance genes (see Chapter 11). PAIs are widely disseminated among medically important bacteria. For example, PAIs have been identified as playing a role in virulence for each of the following organisms:
Escherichia coli (enteropathogenic, enterohemorrhagic or serotoxigenic, (verotoxigenic) uropathogenic, enterotoxigenic, enteroinvasive, enteroaggregative, meningitis-sepsis associated; see Chapter 20).
Biofilm Formation.
Microbial biofilm formation is important to many disciplines, including environmental science, industry, and public health. Biofilm formation affects the efficient treatment of wastewater; it is essential for the effective production of beer, which requires aggregation of yeast cells; and it affects bioremediation for toxic substances such as oil. It has been reported that approximately 65% of hospital-acquired infections are associated with biofilm formation. Box 3-8 provides an overview of pathogenic organisms associated with biofilm formation in human infections.
Outcome and Prevention of Infectious Diseases
Outcome of Infectious Diseases
Given the complexities of host defenses and microbial virulence, it is not surprising that the factors determining outcome between these two living entities are also complicated. Basically, outcome depends on the state of the host’s health, the virulence of the pathogen, and whether the host can clear the pathogen before infection and disease cause irreparable harm or death (Figure 3-11).
The time from exposure to an infectious agent and the development of a disease or infection depends on host and microbial factors. Infectious processes that develop quickly are referred to as acute infections, and those that develop and progress slowly, sometimes over a period of years, are known as chronic infections. Some pathogens, particularly certain viruses, can be clinically silent inside the body without any noticeable effect on the host before suddenly causing a severe and acute infection. During the silent phase, the infection is said to be latent. Again, depending on host and microbial factors, acute, chronic, or latent infections can result in any of the outcomes detailed in Figure 3-11.
Medical intervention can help the host fight the infection but usually is not instituted until after the host is aware that an infectious process is underway. The clues that an infection is occurring are known as the signs and symptoms of disease and result from host responses (e.g., inflammatory and immune responses) to the action of microbial virulence factors (Box 3-9). Signs are measurable indications or physical observations, such as an increase in body temperature (fever) or the development of a rash or swelling. Symptoms are indictors as described by the patient, such as headache, aches, fatigue, and nausea. The signs and symptoms reflect the stages of infection. In turn, the stages of infection generally reflect the stages in host-microorganism interactions (Figure 3-12).
• The severity of the infection, which is determined by host and microbial interactions already discussed
• Accuracy in diagnosing the pathogen or pathogens causing the infection
• Whether the patient receives appropriate treatment for the infection (which depends on accurate diagnosis)
Prevention of Infectious Diseases
The treatment of an infection is often difficult and not always successful. Because much of the damage may already have been done before appropriate medical intervention is provided, the microorganisms gain too much of a “head start.” Another strategy for combating infectious diseases is to stop infections before they start (i.e., disease prevention). As discussed at the beginning of this chapter, the first step in any host-microorganism relationship is the encounter and exposure to the infectious agent. Therefore, strategies to prevent disease involve interrupting or minimizing the risk of infection when exposures occur. As outlined in Box 3-10, interruption of encounters may be accomplished by preventing transmission of the infecting agents and by controlling or destroying reservoirs of human pathogens. Interestingly, most of these measures do not really involve medical practices but rather social practices and policies.
Epidemiology
To prevent infectious diseases, information is required regarding the sources of pathogens, the mode of transmission to and among humans, human risk factors for encountering the pathogen and developing infection, and factors that contribute to good and bad outcomes resulting from the exposure. Epidemiology is the science that characterizes these aspects of infectious diseases and monitors the effect diseases have on public health. Fully characterizing the circumstances associated with the acquisition and dissemination of infectious diseases gives researchers a better chance of preventing and eliminating these diseases. Additionally, many epidemiologic strategies developed for use in public health systems also apply in long-term care facilities (i.e., nursing homes, hospitals, assisted living centers) for the control of infections acquired within the facility (i.e., nosocomial infections; for more information on infection control, see Chapter 80).
The field of epidemiology is broad and complex. Diagnostic microbiology laboratory personnel and epidemiologists often work closely to investigate problems. Therefore, familiarity with certain epidemiologic terms and concepts is important (see Box 3-1).
1. An infection acquired from working with an animal reservoir is:
2. Which of the following is considered an indirect mode of transmission?
3. Nonspecific immunity includes all of the following except:
a. Is activated for all infectious agents
b. Is specific for any organism
6. A sign is different from a symptom in all of the following ways except:
a. It provides measurable data.
b. It is believed to be associated with the etiology of the disease.
d. It includes the temperature, respiratory rate, and pulse.
7. A short-lived infection that manifests with a short incubation period and serious illness is considered to be:
8. A microorganism that colonizes the skin but is capable of causing infection under the appropriate conditions is referred to as:
9. All of the following are involved in humoral immunity except:
a. injection of antigens or antibodies to provide immunity
b. inanimate source of infection
c. limited and specific effect
d. long-term health care−associated infection
e. characteristic of a disease-causing organism
f. serum proteins activated in the immune system
g. circulate in the blood before activation
11. Compare and contrast the components of the specific and nonspecific immune defenses, including the occurrence and process of inflammation; phagocytic cells; antibody production; cellular response; and natural physical or chemical properties of the human body.