Hematologic and hemostatic systems
Maternal physiologic adaptations
Antepartum period
Most hematologic parameters, including blood and plasma volume, cellular components, plasma constituents, and coagulation factors, are altered during pregnancy. These changes are reflected in progressive changes in many common hematologic laboratory values. As a result, it is essential to recognize the normal range of laboratory values and usual patterns of change during pregnancy and to evaluate findings in conjunction with clinical data and previous values in order to distinguish between normal adaptations and pathologic alterations (Table 8-1).
Table 8-1
Normal Laboratory Values in Nonpregnant and Pregnant Women
NONPREGNANT | PREGNANT | |
GENERAL SCREENING ASSAYS | ||
Hemoglobin | 12-16 g/dL (120.0-160.0 g/L) | 11-14 g/dL (110.0-140.0 g/L) |
Packed cell volume (PCV) | 37%-47% | 33%-44% |
Red blood cell count (RBC) | 4.2-5.4 million/mm3 | 3.8-4.4 million/mm3 |
Mean corpuscular volume (MCV) | 80-100 fl | 70-90 fl |
Mean corpuscular hemoglobin (MCH) | 27-34 fl | 23-31 fl |
Mean corpuscular hemoglobin concentration (MCHC) | 32-35 fl | 32-35 fl |
Reticulocyte count | 0.5%-1% | 1%-2% |
SPECIFIC DIAGNOSTIC TESTS | ||
Serum ferritin | 25-200 ng/mL (56.1-449.4 pmol/L) | 15-150 ng/mL (33.7-337.0 pmol/L) |
Serum iron | 135 mcg/dL (24.2 μmol/L) | 90 mcg/dL (16.1 μmol/L) |
Iron binding capacity | 250-460 mcg/dL (44.8-82.3 μmol/L) | 300-600 mcg/dL (53.7-107.4 μmol/L) |
Transferrin saturation | 25%-35% | 15%-30% |
Iron | 135 mcg/dL (24.2 μmol/L) | 90 mcg/dL (16.1 μmol/L) |
Red blood cell folate | 150-450 ng/mL cells (339.9-1019.7 nmol/L cells) | 100-400 ng/mL cells (226.6-906.4 nmol/L cells) |
Serum vitamin B12 | 70-85 ng/dL (51.7-62.7 pmol/L) | 70-500 ng/dL (51.7-369.0 pmol/L) |
Adapted from Morrison, J.C. & Pryor, J.A. (1990). Hematologic disorders. In R.D. Eden & F.H. Boehm (Eds.). Assessment and care of the fetus. Norwalk, CT: Appleton & Lange; Burrow, G.N., Duffy, T.P., & Copel, J.A. (2004). Medical complications during pregnancy (6th ed.). Philadelphia: Saunders.
Changes in blood and plasma volume
Among the most significant hematologic changes during pregnancy are increases in blood and plasma volume (Figure 8-1). These changes result in the hypervolemia of pregnancy, which is in turn responsible for many of the alterations in blood cellular components and plasma constituents. Circulating blood volume increases by 30% to 40% (approximately 1 ½ L), with a usual range of 30% to 45%.46,87,128,179 The increased blood volume is due to an increase in plasma volume that is followed by an increase in the total red blood cell (RBC) volume. Blood volume changes begin at 6 to 8 weeks, peak at 28 to 34 weeks at values about 1200 to 1600 mL higher than in nonpregnant women, then reach a plateau or decrease slightly to term.87,128
Plasma volume increases progressively from 6 to 8 weeks by approximately 45% to 50% (range, 40% to 60%) or about 1200 to 1600 mL above nonpregnant values.54,87,128,158,188 Plasma volume increases rapidly during the second trimester, followed by a slower but progressive increase that reaches its maximum of 4700 to 5200 mL around 32 weeks.87,128 The enlarged plasma volume is accommodated by the vasculature of the uterus, breasts, muscles, kidneys, and skin. The increased volume leads to hemodilution with a net decrease in RBC volume and total circulating plasma proteins.
Plasma volume, placental mass, and birth weight are positively correlated.54,73 Fetal growth correlates more closely with maternal plasma volume increases than with changes in RBC volume. Alterations in the usual increase in plasma volume are associated with pregnancy complications. A greater than normal increase in plasma volume has been observed in multiparous women (probably related to a tendency for higher weight infants) and with maternal obesity, large for gestational age infants, prolonged pregnancy, and multiple gestation.54
In twin pregnancies, plasma volume increases up to 70% over nonpregnant values, with further elevations seen in women with triplets and other multiple pregnancies.38,54 The lower than expected hematocrit seen in these women may be due to hemodilution from excessive plasma volume and may not indicate a problem with erythropoiesis per se. Preeclampsia is associated with a reduction in the expected increase in plasma volume in most studies (see Chapter 9).6,10,29,62,100,108,128 This may be due to vasoconstriction altering the intravascular compartment or a more “leaky” vasculature.10
The etiology of plasma volume changes in pregnancy is thought to be related to the effects of nitric oxide–mediated vasodilation on the renin-angiotensin-aldosterone system and subsequent sodium and water retention (see Chapter 11).32,128 These changes are also influenced by hormonal effects and are closely linked with the alterations seen in fluid balance and in the renal and cardiovascular systems. Hormonal influences, especially the effects of progesterone, on the vasculature of the venous system lead to decreased venous tone, increased capacity of the veins and venules, and decreased vascular resistance. These changes allow the vasculature to accommodate the increased blood volume. Estrogen and progesterone influence plasma renin activity and aldosterone levels, resulting in retention of sodium and an increase in total body water.73 Most of this extra water is extracellular and available to contribute to the increased plasma volume. Changes in plasma volume have also been linked to a mechanical effect, with the low-resistance uteroplacental circulation acting as an arteriovenous shunt. This shunt provides physical space to accommodate the increased cardiac output and corresponding change in plasma volume.38,73
Increased plasma volume and hypervolemia reduce blood viscosity. Hypervolemia also leads to hemodilution and changes in plasma protein and blood cellular components, which further reduce viscosity. Blood viscosity decreases approximately 20% during the first two trimesters. During the third trimester, viscosity may increase slightly. The decreased viscosity reduces resistance to flow and the cardiac effort needed, thus conserving maternal energy resources.38
Changes in blood cellular components
The principal change in blood cellular components during pregnancy is an increase in RBC volume (Table 8-2). This alteration, in conjunction with changes in plasma volume, is reflected in changes in the hemoglobin and hematocrit.
Table 8-2
Changes in Blood Cellular Components During Pregnancy
COMPONENT | CHANGE | PATTERN OF CHANGE | BASIS FOR CHANGE | INTRAPARTUM CHANGES | POSTPARTUM CHANGES |
Red blood cells (RBCs) | Increases 20%-30% (250-450 mL) | Slow, continuous increase beginning in first trimester; may accelerate slightly in third trimester | Erythropoietin stimulated by human placental lactogen, progesterone, and prolactin | Slight increase due to slight hemoconcentration; 50% of increased RBCs lost at delivery | RBC production ceases temporarily; remainder of increased RBCs lost via normal catabolism |
Hematocrit | Decreases 3%-5% to 33.8% at term (range, 33%-39%) | Decreases from second trimester as plasma volume peaks | Hemodilution | Returns to nonpregnant levels by 4-6 weeks as a result of RBC catabolism | |
Hemoglobin | Decreases 2%-10% to 12.1-12.5 g/dL (range, 11-13 g/dL) at term | If iron and folate are adequate, little change to 16 weeks; lowest values at 16-22 weeks; slowly increases to term | Hemodilution; total body hemoglobin increases by 65-150 g | Slight increase as a result of stress and dehydration | Initial decrease; stabilizes at 2-4 days; nonpregnant values by 4-6 weeks |
Reticulocytes | Increase 1%-2% | Gradual increase to third trimester | Increased RBC production | Increases slightly; nonpregnant values by 4-6 weeks | |
White blood cells | Increase 8% to 5000-12,000/mm3 (up to 15,000/mm3 seen) | Begins in second month; increase involves primarily neutrophils | Estrogen | Increase to 25,000-30,000/mm3 | Decrease to 6000-10,000/mm3; normal values by 4-7 days |
Eosinophils Basophils | Probably increases slightly Decreases slightly | Variable | Hemodilution | Disappear from peripheral blood | By 3 days return to peripheral blood |
Platelets | May decrease slightly but within normal adult ranges; usual range 150,000-400,000/mm3 | Variable | 20% decrease with placental separation | Increase by 3-5 days with gradual return to nonpregnant levels | |
Erythrocyte sedimentation rate | Increases | Progressive | Increased plasma globulin and fibrinogen | Increases | Initially 55-80 mm/hr; peaks 1-2 days postpartum |
Changes in red blood cells.
The total RBC volume increases by 20% to 30% (250 to 450 mL) during pregnancy.54,128,158 Changes in RBC volume are due to increased circulating erythropoietin (Epo), which stimulates erythropoiesis and accelerated RBC production. The rise in Epo in the last two trimesters is due to progesterone, possibly prolactin, and human placental lactogen rather than to a decrease in oxygen-carrying capacity, which is the usual stimulus for Epo production.54,128 The magnitude of change in RBC volume varies and is influenced by the woman’s iron stores.38 The increase in RBCs reflects the increase in oxygen demands (which rise 15%) during pregnancy.178,179
The increase in erythropoiesis and total RBC volume begins during the first trimester.54,87 The increase occurs at a relatively constant rate, but slower than changes in plasma volume, and may accelerate slightly during the third trimester.54,73 Hemodilution is maximal at 28 to 34 weeks and leads to a lower hemoglobin, hematocrit and RBC count.87 The increased RBC production results in a moderate erythroid hyperplasia of the bone marrow and an increase in the reticulocyte count.
RBC 2,3-diphosphoglycerate (2,3-DPG) rises beginning early in pregnancy and leads to a gradual shift to the right of the maternal oxygen-hemoglobin dissociation curve (see Chapter 10). This reduces the affinity of maternal hemoglobin for oxygen (see box on p. 301) and favors release of oxygen in the peripheral tissues, including the intervillous space, which facilitates oxygen transfer from mother to fetus and fetal growth.54,73,128 Alterations in placental function that occur with maternal disorders such as preeclampsia, chronic renal disease, diabetes, and severe anemia can decrease oxygen transfer across the placenta. The fetus may develop chronic hypoxia, with stimulation of erythropoietin (Epo) production, increased erythropoiesis, polycythemia, and increased neonatal morbidity.
The mean cell diameter and thickness of the RBCs also change, resulting in a cell that is more spherical in shape. Because the increase in plasma volume is three times greater than the RBC volume increase, the net result is a decrease in the total RBC count, hemoglobin, and hematocrit (see Table 8-2). Changes in the mean corpuscular volume (MCV) and mean corpuscular hemoglobin volume (MCHV) are related to iron status. In women with adequate iron, the MCV and MCHV are relatively stable; in iron-deficient women, these values may decrease.87,179
The hemoglobin and hematocrit decrease from the second trimester on as plasma volume peaks. Thus although total body hemoglobin increases 85 to 150 g in pregnancy, net hemoglobin decreases. Even with adequate iron supplementation, the hemoglobin decreases about 2 g/dL (20 g/L) to a mean of about 11.6 g/dL (116 g/L) in the second trimester as a result of hemodilution.74 At term the hemoglobin averages 12.1 to 12.5 g/dL (121 to 125 g/L), with a range of 11 to 13 g/dL (110 to 130 g/L) versus a mean of 14 ± 2 (140 ± 20 g/L) for nonpregnant females. The Centers for Disease Control and Prevention (CDC) suggests values of 11 g/dL (110 g/L) (first and second trimesters) and 10.5 g/dL (105 g/L) (third trimester) as the lowest acceptable values for screening pregnant women.36,54 The mean hematocrit is 33.8% (range, 33% to 39%) at term.54,87 The fall in the hematocrit may help protect the woman from thromboembolism by decreasing blood viscosity and enhancing perfusion.128 A high hematocrit in a pregnant woman may indicate a low plasma volume and a relative hypovolemia. Changes in the hemoglobin and hematocrit in pregnant women are illustrated in Figure 8-2.
Changes in white blood cells.
Total white blood cell (WBC) volume increases slightly beginning in the second month and levels off during the second and third trimesters (see Table 8-2). The total WBC count in pregnancy varies with individual women, ranging from 5000 to 12,000/mm3, with values as high as 15,000/mm3 reported.87,128 The increased WBC count is due to a neutrophilia with an elevation in mature leukocyte forms. A slight shift to the left may occur with occasional myelocytes and metamyelocytes seen on the peripheral smear.87 Changes in other WBC forms are minimal (see Table 8-2), with a possible slight increase in eosinophils and slight decrease in basophils and no systematic changes in monocytes.87 Leukocyte alkaline phosphatase activity rises during pregnancy, falling several days before to delivery. Changes in leukocytes accompanying pregnancy are similar to changes that occur with physiologic stress, such as vigorous exercise, with return to the circulation of mature leukocytes that were previously shunted out of the circulatory system.46 The basis for these changes is unclear but is probably related to hormonal changes.54,87 The neutrophil count normally increases slightly with the estrogen peak during the menstrual cycle. In women who become pregnant, the neutrophils continue to increase after fertilization, peaking around 30 weeks then remaining stable to term. The total lymphocyte count is unchanged, as are numbers of circulating B and T lymphocytes (see Chapter 13).
Changes in platelets.
In general, platelet values do not change significantly during pregnancy.71,87 A slight decrease in platelet count, probably due to hemodilution, and an increase in platelet aggregation during the last 8 weeks of pregnancy has been reported, suggesting a low-grade activation and consumption of platelets.69,71,87 In healthy pregnant women, platelet counts have generally not been reported at values below lower limits for normal nonpregnant women.87,117 Mild to moderate thrombocytopenia (less than 150,000/mm3) has been reported in late pregnancy in 7% of healthy pregnant women.26,87,181 This may be due to hemodilution, decreased platelet production, or increased turnover.24 The acceptable range for platelet values in pregnancy is 150,000 to 400,000/mm3.
Changes in plasma components
Many components of plasma—including plasma proteins, electrolytes, serum iron, lipids, and enzymes—change during pregnancy (Table 8-3). Total plasma proteins decrease 10% to 14%, with much of the change occurring in the first trimester. Although there is an absolute increase in albumin concentration during the first trimester, there is a relative decrease due to increased blood volume and hemodilution. Decreased albumin leads to a net decrease in colloid osmotic (oncotic) pressure, reducing the normal forces counteracting edema formation. Although edema formation in pregnancy is primarily due to alterations in venous hydrostatic pressure, decreased oncotic pressure from the relative decrease in albumin is an important contributory factor.15
Table 8-3
Changes in Plasma Components During Pregnancy
COMPONENT | CHANGE | TIMING | BASIS | SIGNIFICANCE |
Total plasma proteins | ↓10%-14% | First trimester | Estrogen/progesterone | ↓ Colloid osmotic pressure (edema formation)Altered protein binding of calcium, drugs, and so on |
Albumin | Total: 144 gSerum: 3.5 g | First trimester | Estrogen/progesterone | See above |
Fibrinogen | ↑50%-80% | First to third trimesters | Hemodilution | Alterations in hemostasis ↓ Erythrocyte sedimentation rate |
Globulin | ↑ | First to third trimesters | Estrogen/progesterone | ↓ Erythrocyte sedimentation rate |
α- and β-globulin | ↓ | Progressive throughout pregnancy | Estrogen/progesterone | See individual globulinsFacilitate transport of carbohydrates and lipids to placenta and fetus |
γ-Globulin | ↓ | Third trimester | Transplacental passage of IgG | |
Thyroxin-binding globulin | ↑ | First trimester | ↑Plasma T3 and T4 | |
α1-Antitrypsin | Doubles | Protects lungs from deported trophoblast tissue | ||
α2-Macroglobulin | ↑20% | Antiplasmin effect, which may predispose to DIC | ||
Total serum lipids | ↑40%-60% | Continuous to term | Human placental lactogen and altered metabolism | |
Cholesterol | ↑40% | Continuous to term | Essential precursor for steroid hormones (e.g., estrogen, progesterone) | |
Phospholipids | ↑37% | Continuous to term | Major component of cell membranes needed for maternal and fetal growth | |
β-Lipoprotein | ↑Up to 180% | Possible ↑ risk of thrombosis | ||
Serum electrolytes | ↓ 5-10 mg/L | First trimester | ↓ Plasma osmolarity | |
Serum ferritin | ↓ 30% | To 28 weeks (with adequate iron) or 30-32 (without) | Hemoglobin synthesis (early) Fetal uptake (late) | Reflects decreasing iron stores |
Transferrin | ↑ 70% | Linear rise | Altered liver function | Facilitates Fe absorption and transport |
Iron-binding capacity | ↓ 15% |
DIC, Disseminated intravascular coagulation; Fe, iron; IgG, immunoglobulin G.
Globulin concentration demonstrates both absolute and relative increases, leading to progressive falls in the albumin-to-globulin ratio. Both α- and β-globulin increase progressively during pregnancy; γ-globulin decreases slightly. Fibrinogen also demonstrates both absolute and relative increases of 50% to 80%.15,46 The erythrocyte sedimentation rate (ESR) increases progressively during pregnancy, probably due to the elevation in plasma globulin and fibrinogen levels. Alterations in other plasma proteins are summarized in Table 8-3.
The alterations in plasma proteins alter protein binding of substances such as calcium, drugs, and anesthetic agents. Because many drugs are transported in the blood bound to albumin, doses of some drugs may need to be altered during pregnancy (see Chapter 7). Increased binding of substances such as calcium reduces the level of free calcium in the maternal plasma. As a result, calcium must be actively transported across the placenta to the fetus (see Chapter 17).
Decreases in serum electrolytes (anions, cations, and buffer base) reduce plasma osmolarity by 8 to 10 mOsm/L during the first trimester.15 These changes are due to both hypervolemia and the effects of respiratory system alterations, particularly hyperventilation with increased CO2 loss (see Chapter 10).38
Serum iron decreases during pregnancy, especially after 28 weeks and in women without adequate iron stores. Iron needs during pregnancy are summarized in Table 8-4. Serum ferritin is a more precise indicator of reticuloendothelial iron stores. 1 mcg/L (2.5 pmol/L) serum ferritin is equal to 8 mg of stored iron in the adult. Serum ferritin levels in pregnancy are 15 to 150 ng/mL (33.7 to 337 pmol/L).54 In women without adequate iron, serum ferritin levels fall until 30 to 32 weeks and then stabilize. The greatest decrease in serum ferritin is between 12 and 25 weeks due to the rapid expansion of maternal RBC volume during this time (see Figure 8-2).189 With adequate iron, serum ferritin levels stabilize by 28 weeks or slightly earlier, and may even rise near term.15,54 Decreases in serum ferritin in early pregnancy are due to mobilization of iron stores for maternal hemoglobin synthesis; later decreases are due to increased fetal iron uptake. In multiparous women the decrease in serum ferritin occurs earlier and may be greater.
Table 8-4
Iron Requirements for Pregnancy
REQUIRED FOR | AVERAGE (mg) | RANGE (mg) |
External iron loss | 170 | 150-200 |
Expansion of red blood cell mass | 450 | 200-600 |
Fetal iron | 270 | 200-370 |
Iron in placenta and cord | 90 | 30-170 |
Blood loss at delivery | 150 | 90-310 |
Total requirement | 980 | 580-1340 |
Requirement less red blood cell expansion | 840 | 440-1050 |
From Kilpatrick, S.J. & Laros, R.K. (2004). Maternal hematologic disorders. In R.K. Creasy, R. Resnik, & J.D. Iams (Eds.). Maternal-fetal medicine: Principles and practice (5th ed.). Philadelphia: Saunders.
Levels of serum lipids rise with marked elevations in cholesterol and phospholipids. Cholesterol is an essential precursor for steroid hormone production by the placenta; phospholipids are major components of cell membranes. The rise in serum lipids begins in the first trimester, increasing to 40% to 60% at term (see Table 8-3). Increases in serum alkaline phosphatase are due to increased placental production. As a result, alkaline phosphatase levels are not useful in evaluating liver disorders during pregnancy. Serum cholinesterase activity decreases by 30%. Increased cholinesterase activity may lead to longer periods of paralysis if substances such as succinylcholine are used during surgical procedures.15
Changes on coagulation factors and hemostasis
Pregnancy has been called an acquired hypercoagulable state, reflecting an increased risk for thrombosis and consumptive coagulopathies such as disseminated intravascular coagulation (DIC). Pregnancy is associated with increased clotting potential, decreased anticoagulants, and decreased fibrinolysis.7,103 Hemostatic changes during pregnancy are thought to result in an ongoing low-grade activation of the coagulation system in the uteroplacental circulation, beginning as early as 11 to 15 weeks. This state of compensated intravascular coagulation is characterized by thrombin formation and local consumption of clotting factors in which component synthesis equals or exceeds consumption.69,169 Figures 8-3 to 8-5 summarize coagulation and fibrinolysis.
Intravascular and extravascular fibrin deposits are found in the uteroplacental circulation, intervillous spaces, and placental bed. Tissue factor is found in amniotic fluid, placenta, decidua, and endometrial stroma.93,103 Tissue factor (previously called thromboplastin) may play a nonhemostatic role in angiogenesis, cell signaling and embryogenesis.63 Circulating high-molecular-weight, soluble fibrin-fibrinogen complexes—which are indicative of uteroplacental fibrin formation—also increase. During pregnancy, smooth muscle and elastic tissue within the uterine spiral arteries are replaced by a fibrin matrix (see Chapter 3). These changes allow for expansion of the vessels to accommodate increased blood flow to the placenta and to facilitate collapse of the terminal portion of the vessel with placental separation.69,129,133,178 Elevated levels of plasminogen-activated inhibitors may assist with the deposition of fibrin in the maternal blood vessels.65 Increased fibrinogen, thrombin generation, and inhibition of fibrinolysis during pregnancy may interact to ensure integrity of uteroplacental vessels.103 During late pregnancy, accumulation of mural thrombi in the vessel walls decreases the diameter of the lumen, reducing blood flow, which may result in the placental infarcts and small areas of ischemia often seen at term.
Alterations are seen in coagulation (procoagulant) factors, coagulation inhibitors, and fibrinolysis. Contact factors involved in initiation of the clotting cascade and many coagulation factors are elevated in pregnancy.69,103,129 Factors I (fibrinogen), VII, VIII, IX, XII, and X; as well as von Willebrand factor (vWF), which is important in platelet adhesion, are markedly increased.26,42,71,103,129,179 Factors II and V are generally unchanged.103 Factor XIII increases initially then decreases to about 50% of nonpregnant values in late pregnancy.26,65 Factor XI decreases to term.103 These changes increase thrombin generating potential.103 A concurrent increase in fibrinogen and decrease in factor XIII (fibrin stabilizing factor) alters the process of clot stabilization and subsequent lysis during pregnancy.69 Thrombin-antithrombin complexes double by the third trimester, indicating increased thrombin generation.26,133
Changes in coagulation factors during pregnancy are reflected in the activated partial thromboplastin time (aPTT) and prothrombin time (PT), which decrease slightly from midpregnancy to term.26 D-dimer concentrations increase progressively, which is consistent with the hypercoagulable state of pregnancy.42,133 Bleeding times are normally unchanged.71
Coagulation inhibitors form an endogenous anticoagulant system that inhibits hemostasis (see Figure 8-4). Absolute levels of antithrombin are generally unchanged during pregnancy or may be slightly decreased by term.71 Both free and total protein are decreased.103,128 Free protein S decreases by 60% to 70%, with the lowest levels seen at delivery.103 This decrease is due to an increase in its carrier protein (complement 4β-binding protein).103 Activated protein S may be decreased by up to 40% during pregnancy.65,103 Levels of protein C, which is complexed to protein S, do not change; however, resistance to activated protein C increases, particularly in the second and third trimesters, due to the changes in protein S.103 Thrombomodulin, a marker of endothelial damage, which activates protein C, is increased.27,102 Tissue factor pathway inhibitor increases while heparin cofactor II and α1-macroglobulin are generally unchanged.26,71
Fibrinolytic activity (see Figure 8-5) increases early in pregnancy, then decreases to term.42,71,133 Type 1 and type 2 plasminogen activator inhibitors (PAI-1 and PAI-2) inhibit fibrinolysis. PAI-1 is produced by the decidua and increases 3 to 5 fold during pregnancy.103,115 PAI-2 is produced by the placenta and increases to term impairing maternal fibrinolytic activity.27,65,103,115 PAIs also regulate local fibrin deposition in the uteroplacental vessels and have been reported to be further increased with preeclampsia.102 Circulating fibrin degradation products are increased, possibly due to increased fibrin generation and degradation in the placenta.42 Since D-dimer values, a marker of fibrinolysis, are increased, this laboratory test must be used with caution for clinical evaluation in pregnancy.65,133
The net effect of changes in hemostasis during pregnancy is increased activity of most procoagulant proteins and a lowering of factors that inhibit coagulation, resulting in increased thrombin generation. The result is a hypercoagulable state that promotes clot formation, extension, and stability.103,129 The hypercoagulable state of pregnancy is balanced to some extent by changes in plasminogen. Plasminogen is elevated and tissue plasmin inhibitors are decreased, which helps retain the dynamic equilibrium between clotting and clot lysis and thus overall hemostatic balance during pregnancy.65,69 The increased tendency toward coagulation during pregnancy may also be partly balanced by pregnancy-specific proteins, which act similarly to heparin to facilitate neutralization of thrombin. Many clotting factors are synthesized by the liver and influenced by estrogen, and many of the changes seen in pregnancy are similar to those seen with oral contraceptives.42 Estrogen may also alter uptake of coagulation proteins by the uterus and increase uterine tissue factor production in preparation for rapid onset of coagulation after placental separation.18,42,165
Intrapartum period
Changes in the hematologic system and hemostasis during pregnancy are crucial in preparing the woman to tolerate the normal blood loss at delivery and prevent significant bleeding with placental separation. The amount of blood loss with delivery is up to 500 mL (vaginal delivery) or 1000 mL (cesarean section or a vaginal delivery of twins) and occurs with minimal changes in blood pressure or pulse.15,46,54,128 This loss at delivery may be underestimated by up to 50%.107 Blood loss at delivery and in lochia over the first few postpartum days account for about half of the increased RBC volume acquired during pregnancy.46
Changes in hematologic parameters
Hemoglobin levels tend to increase slightly during labor due to hemoconcentration. The degree of hemoconcentration is related to increases in erythropoiesis (as a stress response), muscular activity, and dehydration.46 Leukocyte alkaline phosphatase activity increases with the onset of labor. Increases in this enzyme are often associated with inflammatory responses. The WBC count increases during labor and immediately postpartum to values up to 25,000 to 30,000/mm3. This increase is primarily due to an increase in neutrophils and may represent a response to stress.149 Circulating eosinophils decrease and may disappear.179 Intrapartum changes in hematologic parameters are summarized in Table 8-2.
Changes in hemostasis
The coagulation system undergoes further activation during the intrapartum period both before and after placental separation. The placenta and decidua are rich in tissue factor, the primary initiator of coagulation. Tissue factor is a membrane-bound protein expressed on the surface of cells that are normally not in direct contact with plasma.133 With cellular injury, such as with placental separation, tissue factor is released and activates coagulation (see Figure 8-3). Concentrations of clotting factors increase during labor. PT shortens significantly, especially during the third stage of labor with clotting at the placental site. Levels of fibrinogen and plasminogen may also decrease as a result of their increased utilization after placental separation.69 Factor VIII complex increases during labor and delivery. Factor V increases after placental separation, which contributes to activation of clotting.
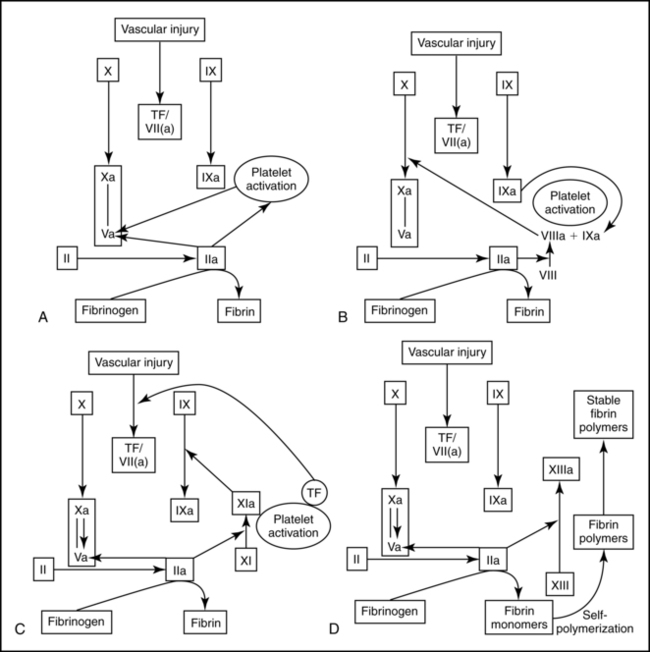
Fibrinolytic activity decreases further during labor and delivery, enhancing formation of clots at the placental site following separation.15,69,94 This promotes development of a hemostatic endometrial fibrin mesh over the wound. About 5% to 10% of the total body fibrin is deposited at this site.69
Fibrin levels and fibrin-fibrinogen degradation products (FDP) reach their nadir with placental separation.42 FDP and d-dimer both increase after delivery.42,71,133 This change may increase the risk of coagulation disorders in the immediate postpartum period by interfering with formation of firm fibrin clots.15,69 The number of platelets falls about 20% with placental separation due to clotting at the placental site. Platelet activation and fibrin formation are maximal at delivery (Figure 8-6). Thus the hypercoagulable state of pregnancy is further magnified during the intrapartum period. This state protects the woman from hemorrhage and excessive blood loss at delivery by providing for rapid hemostasis following removal of the placenta.
Postpartum period
Changes in blood volume
The decrease in blood and plasma volume during the immediate postpartum period corresponds to the amount of blood loss with delivery. This loss usually accounts for over half of the RBC volume accumulated during pregnancy.38 During the first few days after delivery, plasma volume decreases further as a result of diuresis. After 3 to 4 days, mobilization of interstitial fluid leads to a slight increase in plasma volume. This hemodilution decreases hemoglobin, hematocrit, and plasma protein by the end of the first postpartum week.38 The volume change may contribute to circulatory embarrassment in women with cardiac problems (see Chapter 9). Plasma volume continues to decrease after the first week, reaching nonpregnant values by 6 to 8 weeks or earlier.
Accurate and consistent assessment of blood loss is essential at delivery and postpartum. Blood losses during these periods are reported to be both underestimated and overestimated. Unit and product-specific standards for estimating losses increase accuracy of these assessments.155
Changes in hematologic parameters
Increased RBC production ceases early in the postpartum period due to suppression of Epo.38,157 Mean hemoglobin levels decrease slightly in the first 24 hours after delivery, then plateau for 4 days, followed by a slow rise to day 14.54,87 Hematocrit values follow a similar pattern. A 500-mL blood loss such as may occur with vaginal delivery will usually result in a 1-g reduction in hemoglobin. The hematocrit returns to nonpregnant levels by 4 to 6 weeks with the normal catabolism of RBCs. The reticulocyte count is increased by the end of pregnancy, with a further increase during the first 1 to 2 postpartum days, followed by a rapid decrease. The initial increase may be a result of Epo stimulation because of decreased RBC oxygen-carrying capacity from blood loss at delivery.54,87
Excess circulating hemoglobin is catabolized, and the iron released is stored in the reticuloendothelial system. During the first 2 weeks, there is little change in serum ferritin levels, with a decrease in transferrin and increase in serum iron, and marked decrease in C-reactive protein.54,87 Serum ferritin levels increase by 5 to 8 weeks to levels seen early in pregnancy. This increase occurs regardless of whether or not the woman received supplemental iron during pregnancy and is more marked after vaginal delivery.54,74
The WBC count, which increases in labor and immediately postpartum, falls to 6000 to 10,000/mm3, then returns to normal values by 6 days.87,170 Eosinophils, which tend to disappear from the peripheral blood during delivery, return by 3 days postpartum; basophils and monocytes remain unchanged.178,179 The number of platelets, which falls following placental separation, increases beginning 3 to 4 days postpartum and gradually returns to nonpregnant levels. It is unclear whether this change is due to stimulation of platelet production or is secondary to changes in blood volume.54,87 Postpartum changes are summarized in Table 8-2.
Changes in hemostasis
Fibrin degradation products increase after placental delivery.42 Fibrinolytic activity returns to normal ranges as early as 1 hour after delivery and fibrinolysis to nonpregnant levels by 24 to 48 hours.26,71 Return to normal activity reflects removal of the fibrinolytic inhibitors produced by the placenta. PAI-1 and PAI-2 decrease after separation of the placenta. PAI-1 reaches non-pregnant values by 5 weeks.26 PAI-2 that was originally produced by the placenta can be detected for up to 8 weeks.71 Secondary increases in fibrinogen, factor V, and factor VIII complex occur during the first week, followed by a return to predelivery levels by 1 to 7 days, and a slow decrease to nonpregnant levels.42 Clotting factors, which increase during labor, slowly decrease, reaching their lowest levels by 7 to 10 days. Factors VII and X return to normal levels by 2 weeks. The hemostatic system usually returns to nonpregnant status at 4 to 6 weeks postpartum, although levels of free protein S may remain low for up to 8 weeks.71 Activated protein C resistance decreases to nonpregnant values by 1 to 7 days.42 Changes in the flow velocity and diameter of the deep veins may take up to 6 weeks to return to prepregnant levels.115 Thus the postpartum period continues to be a time of increased risk for thromboembolism.
Clinical implications for the pregnant woman and her fetus
The increased blood and plasma volume during pregnancy results in a physiologic hypervolemia that allows the woman to tolerate many of the changes associated with pregnancy and delivery and helps to (1) meet the demands of an enlarged uterus and hypertrophied vascular system while maintaining normal systemic blood pressure; (2) allow the woman to tolerate blood loss at delivery; (3) protect the woman from impaired venous return and hypotension with position changes late in the third trimester (when a significant proportion of her fluid volume may be sequestered within the venous system of the lower extremities) and thus reduce the risk of supine hypotension; (4) enhance maternal-fetal exchange of gases and nutrients; and (5) increase cutaneous blood flow by fourfold to sevenfold, thus assisting with heat dissipation via the skin.15,38,46,54
The increased red blood cell (RBC) volume helps offset hemodilution and maintain blood oxygen-carrying capacity and availability of oxygen for the fetus. The increased volume allows for adequate blood flow within the expanding uteroplacental circulation, thus ensuring adequate nutrient availability for the fetus throughout gestation. These changes alter evaluation of hematologic variables in the pregnant woman (see Table 8-1).
Iron requirements during pregnancy
Iron stores in nonpregnant women are generally marginal due to menstrual blood, and thus iron, loss. The usual menses loss is 12 to 15 mg of elemental iron (1 mL blood is equal to 0.5 mg of iron).87 Most women have a daily iron intake of about 9 mg/day, below the recommended 12 to 18 mg.128 Worldwide, an estimated 20% of fertile women have iron reserves greater than 500 mg, the minimum iron requirement in pregnancy; 40% have reserves between 100 and 500 mg; and 40% have reserves less than 100 mg.128 In the United States, pregnant women have an average of 300 mg of iron stored at the beginning of pregnancy. So, to meet the iron demands of pregnancy, women must absorb an additional 500 to 700 mg (or 2 to 8 mg/day more than normal).109 The World Health Organization estimates that the prevalence of anemia in pregnant women worldwide is 41.8%.203,204 However, the prevalence varies by geographic area with estimates of 55.8% of pregnant women in Africa, 41.6% in Asia, 31.1% in Latin America and the Caribbean, 30.4% in Oceania, 18.7% in Europe, and 6.1% in North America.203
Iron requirements increase during pregnancy by about 1 g over the usual body iron stores of 2 to 2.5 g in adult women.109 Table 8-4 summarizes how this additional iron is used during pregnancy. The need for iron begins early in the second trimester and peaks in the second half of pregnancy (increasing from an additional 0.8 mg/day in early pregnancy to 7.5 mg/day by term).109,128
The amount of iron needed by the fetus and placenta and to replace usual maternal losses is an obligatory requirement that is met regardless of the cost to maternal iron stores. Even if the mother is has low iron levels and is anemic, the fetus will usually not suffer, because the placenta continues to transport iron to meet fetal needs. If adequate iron is not available for synthesis of additional maternal RBCs, her RBC volume will not increase to the usual levels and the hematocrit and hemoglobin will decrease further as plasma volume increases.46,87
Low iron levels in low income countries have been associated with an increase in maternal and neonatal morbidity and mortality.181 Iron-folic acid supplementation during pregnancy has been reported to increase hemoglobin levels by 1.17 g/dL in high income countries and 1.13 g/dL in low income countries.160 In low income countries, use of these supplements were estimated to decrease maternal anemia by ⅓ to ½ over 10 years.160 However, routine use of prophylactic iron supplementation in pregnancy continues to be controversial in the United States with little evidence documenting significant improvements in perinatal outcomes.5,75,87,145,189 A Cochrane meta-analysis of 49 trials (n = 23,300 pregnant women) found that daily iron or iron plus folate supplementation increased hemoglobin levels before birth and decreased the risk of anemia at term, however no significant reduction in low birth weight infants, delayed development, preterm birth, infection, or postpartum hemorrhage was found.145 A beneficial effect on neonatal iron stores and a risk of hemoconcentration (hemoglobin levels >130 g/dL) with daily supplementation were suggested.145
Although intestinal iron absorption increases in the second half of pregnancy (probably as a compensatory mechanism in response to increased iron demand), dietary sources and maternal stores alone may not be adequate to meet the increased demands of pregnancy. Iron stores affect iron absorption. Women who have good iron stores have minimal increases in absorption during the first trimester, then increase absorption during the second trimester. By later pregnancy, iron stores may be exhausted and iron needs met primarily from absorption.109 Thus if a woman has good iron stores before pregnancy and a diet high in bioavailable iron, most of these women will not develop anemia if they are not supplemented.87 However, iron stores in young, healthy nonpregnant women may be marginal to nonexistent.34
Iron supplementation increases iron reserves, hemoglobin levels, and decreases the risk of iron deficiency anemia during pregnancy and postpartum, even in women with good iron stores at the beginning of pregnancy.128 Even with adequate nutrition, 10% to 20% of pregnant women will develop an iron deficiency.74,75 General recommendations made by the Institute of Medicine (IOM) for iron supplementation during pregnancy are 30 mg ferrous iron (which is provided by 150 mg ferrous sulfate, 300 mg ferrous gluconate, or 100 mg ferrous fumarate) daily beginning at 12 weeks’ gestation along with a well-balanced diet.75 The need for iron can also be met with supplementation of 60 to 85 mg/day of elemental iron beginning at 20 to 28 weeks.75,87 Although the IOM recommended supplementation only if serum ferritin levels were <20 μg/L, the high cost of this screening has limited applicability.75,87 Iron supplementation does not prevent or correct the normal decline in hemoglobin seen in pregnancy but it can prevent depletion of stores, the first stage of iron deficiency anemia.74
Severe anemia and pregnancy
Anemia during pregnancy is defined as a hemoglobin <11 g/dL by the World Health Organization and <10.5 g/dL in the second trimester and <11.1 g/dL in the third trimester by the Center for Disease Control.188 The most common anemias encountered in pregnancy are iron deficiency anemia, megaloblastic anemia of pregnancy (folic acid deficiency), sickle cell disorders, and α- and β-thalassemia.54 Changes in the hematologic system may influence the course of these disorders during pregnancy and alter fetal outcome. Anemia caused by iron or folate deficiency is due to underproduction of RBCs and is associated with decreased reticulocytes. The normal hematologic changes along with altered nutritional needs during pregnancy increase the risk for these nutritional anemias.
In women with severe anemia (hemoglobin less than 6 to 8 mg/dL [60 to 80 g/L]), maternal arterial oxygen content and oxygen delivery to the fetus are decreased. The fetus attempts to adapt through increased placental blood flow, redistribution of blood within the fetal organs, increased RBC production (to increase the total oxygen-carrying capacity), and a decrease in the diffusing distance for oxygen across the placenta. Because fetuses have predominantly fetal hemoglobin, they cannot readily increase the availability of oxygen to the tissues by further altering the affinity of hemoglobin for oxygen. Although the fetus adapts, the cost may be high, with decreased growth and an increased mortality due to the lack of an adequate oxygen supply and nutrients. Several meta-analyses have found significant relationships between maternal anemia (hemoglobin less than 8.5 g/dL [85 g/L]) in early pregnancy and preterm birth, but not with small-for-gestational-age infants.165 Conversely, an elevated hemoglobin (greater than 14.5 [145 g/L]) in early pregnancy is associated with stillbirth and small-for-gestational-age infants, perhaps because the increased hemoglobin is due to a reduction in the usual increase in plasma volume and thus reduced blood flow to the intervillous space.179
Iron deficiency anemia
The most common cause of anemia during pregnancy is iron deficiency anemia.36,87 Generally this form of anemia is preventable or easily treated with iron supplements. Hematologic changes in pregnancy can make diagnosis of iron deficiency difficult.54 Total iron binding capacity and serum iron often fall during pregnancy, as well as with iron deficiency anemia. A useful test for iron deficiency in the pregnant woman is measurement of serum ferritin levels, which correlate well with iron stores during pregnancy.54 Serum ferritin levels lower than 12 mcg/L with a low hemoglobin indicate iron deficiency, which can be treated with ferrous sulfate until the hemoglobin returns to levels normal for the stage of gestation.54,74 Because ferritin is an acute phase reactant, falsely high levels may occur with infection. Therefore serum ferritin levels less than 60 to 100 mcg/L may indicate deficiency if infection is present.54
In general, even with significant maternal iron deficiency, the fetus will often be protected and receive adequate stores at cost to the mother. However, if the mother is severely iron deficient and anemic, the fetus may have decreased RBC volume, hemoglobin, iron stores, and cord ferritin levels and an increased risk of iron deficiency during infancy.38,54,87 Iron deficiency anemia before midpregnancy is associated with an increased risk of low birth weight, preterm birth, and perinatal mortality.54,74,128
Megaloblastic anemia
Megaloblastic anemia in the nonpregnant woman is usually due to folic acid or vitamin B12 deficiency. Folic acid deficiency is the most common cause of megaloblastic anemia encountered during pregnancy.54 Vitamin B12 deficiency with pregnancy is less common because (1) stores of vitamin B12 are normally large (most women have a 2- to 3-year store in their liver), so deficient states take years to develop; (2) vitamin B12 is used for DNA replication, so a severe deficiency usually leads to infertility; and (3) vitamin B12 deficiency is usually due to pernicious anemia, a disorder seen primarily in older women.54,87 Vitamin B12 dietary deficiency in pregnancy most often occurs with a vegan diet. Vitamin B12 deficiency may be misdiagnosed in a pregnant woman, because serum vitamin B12 levels fall with the expanded plasma volume and consequent hemodilution.54
Folate demands increase threefold during pregnancy from 50 μg/day to 300 to 500 μg/day during pregnancy.87 Because folic acid is essential for DNA synthesis and cell duplication, folate is needed for growth of the fetus and placenta as well as for maternal RBC production. Folate requirements increase throughout pregnancy and are higher in multiple pregnancy.54 Maternal serum folate levels fall during pregnancy, and women with an inadequate dietary intake need supplements. Prenatal vitamins generally contain 1 g of folate; increased levels are needed for individuals with hematolytic disorders. The changes in folate metabolism during pregnancy are due to decreased serum folate and RBCs, increased plasma clearance, increased urinary excretion, and altered histidine metabolism.121
Severe folic acid deficiency has been associated with fetal malformations, preeclampsia, abruptio placenta, prematurity, and low birth weight.54 Maternal folate deficiency increases the risk of neural tube defects (NTDs) and has been associated with a increased risk of cleft lip and palate and preterm birth.35,54,87,114,121 A recent Cochrane meta-analysis (5 trials; n = 6105) confirmed that daily folic acid supplementation reduced the risk of neural tube defects, but did not find evidence for a role in preventing cleft lip and palate, congenital heart disease, miscarriage, or other defects.52 Supplementation is recommended for all women of childbearing age to reduce the risk of neural tube defects. Supplementation should begin before pregnancy because NTDs occur early in the first trimester (see Chapter 15).35 During pregnancy, folate is included in prenatal vitamins.111 In later pregnancy, the fetus has higher levels of folate and elevated folate-binding protein, which protects against fetal folate deficiency; even with low maternal folate levels, neonatal cord levels are usually within normal limits.
Sickle cell disease
Sickle cell disease is a group of disorders that involve mutations in the genes that determine hemoglobin β-chain structure. In women with sickle cell anemia, tissue deoxygenation or acidosis triggers structural changes in the sickle hemoglobin (HbS), so the RBCs take on a half-moon or sickle appearance. The sickled cells can obstruct blood flow in the microvasculature. The areas most susceptible to obstruction are those characterized by slow flow and high oxygen extraction such as the spleen, bone marrow, and placenta. Obstruction leads to venous stasis, further deoxygenation, platelet aggregation, hypoxia, acidosis, further sickling, and eventually infarction.54,87
A woman with sickle cell anemia normally has a lower hemoglobin level (7 to 8 g/dL [70 to 80 g/L]) and oxygen-carrying capacities, to which her system has adjusted. Pregnancy places both the woman and her infant at greater risk for complications due in part to the effects of hematologic, cardiovascular, renal, and respiratory changes during pregnancy.158 As plasma volume increases during pregnancy, the woman may become slightly more anemic. In addition, she may experience an increased risk of sickling attacks. Sickle cell crises are triggered by physical or emotional stress, which may be caused by infection, trauma, hypoxia, and pregnancy. Crises in pregnancy and postpartum may be related to the hypercoagulable state, increased susceptibility to infection, or vascular stress.158 The rapid hemodynamic changes postpartum often precipitate crises, especially if associated with a long or difficult labor and delivery.87 Fetal and neonatal complications such as prematurity and fetal growth restriction may arise due to placental infarction and fetal hypoxia. Fetal hypoxia results from decreased oxygen transport due to the abnormal biochemistry of the maternal hemoglobin and the loss of functional placental tissue for gas and nutrient exchange caused by the infarctions.54,87
Thalassemias
Thalassemia is a disorder in the synthesis of either the α or β peptide chains of the hemoglobin molecule. This leads to alterations in the RBC membrane and decreased RBC life span. α-Thalessemia is an alteration in the production of α chains, in which one or more of the four genes on chromosome 16 that are involved in α-chain production are altered or deleted. Phenotype and severity depend on how many genes are involved and whether the genes are deleted (nonfunctional) or present but with altered function.194 Most pregnant women with α-thalessemia have one or two genes affected and have either a silent presentation or mild anemia.87,194 If all four α-chain genes are missing, the fetus cannot synthesize either normal fetal hemoglobin (composed of two α- and two γ-chains) nor adult hemoglobin (composed of two α- and two β-chains). Infants develop high output cardiac failure, hydrops fetalis, and are often stillborn or die shortly after birth. Intrauterine transfusions are associated with decreased perinatal mortality and morbidity. These infants currently require lifelong transfusion therapy; however, stem cell transplants have been reported to be effective in treating this disorder.194
β-Thalassemia minor is a frequently encountered β-thalassemia during pregnancy. Many females with thalassemia major (Cooley anemia) die in childhood or adolescence; those who survive are often amenorrheic and infertile.54,87 Some pregnancies have occurred in these women, with an increased risk of fetal loss.54 Women with β-thalassemia minor have a mild hypochromic, microcytic anemia, but are usually healthy otherwise and generally do not have increased maternal or infant morbidity if their condition is stable.87 With the plasma volume expansion that accompanies pregnancy, they may develop a more pronounced anemia, but they generally require only supportive care.188 Women with β-thalassemia intermedia may develop a more severe anemia during pregnancy, especially in the second and third trimesters, and may require transfusion therapy.25
Thromboembolism and pregnancy
The hypercoagulable state of pregnancy is crucial in protecting the mother against excessive blood loss with delivery and placental separation. However, the hypercoagulable state is also a disadvantage because it significantly increases the risk of thromboembolic disorders during pregnancy and postpartum. Venous thromboembolism (VTE) is a leading cause of maternal mortality in the United States.102
The risk of VTE increases up to sixfold during pregnancy (0.76 to 1.723 per 1000 pregnant women).115 The risk increases with parity and age, and is nine times higher among women with cesarean deliveries than among women who give birth vaginally.102,196 VTE includes both deep vein thrombosis (DVT) and pulmonary emboli.102 Pulmonary embolism occurs in 1 in 2000 pregnancies and is a major cause of maternal mortality.69,102 Pulmonary emboli usually result from dislodged deep venous thrombi in the lower extremities.
The three factors (Virchow triad) that predispose to thromboembolic disorders (stasis, altered coagulation, and vascular damage) are all present or potentially present during pregnancy.102 Ninety-eight percent of DVTs arise in the lower extremities.102 During pregnancy, increased venous capacitance (due to the effects of progesterone and local endothelial production of prostacyclin [PGI2] and nitric oxide) leads to increased distensibility, decreased flow in the lower extremities, and venous stasis. By late pregnancy, the velocity of venous blood flow in the lower extremities has been reduced by half, and venous pressure has risen an average of 10 mmHg (1.33 kPa).69,102,115 The blood flow velocity decreases by early in the second trimester, with a nadir from 34 weeks to term that does not return to prepregnant values until 6 or more weeks after delivery. In addition, the diameter of the major leg veins increases, more so on the left than the right. During pregnancy, greater than 80% of DVTs occur on the left (versus 55% in nonpregnant women).102,133,156 This is because of compression of the left iliac vein by the right iliac and ovarian veins. About three fourths of DTVs occur during the antepartum period, and one fourth occur postpartum.102,156,196 Most pulmonary emboli develop postpartum and are associated with cesarean section.102
The hypercoagulable state during pregnancy increases the risk of clot formation. The increased fibrinogen, coagulation factors, and decreased protein S and resistance to activated protein C increased thrombin generation, decrease endogenous anticoagulants and impair fibrinolysis. If a clot develops, the decreased fibrinolytic activity and increased PAI-1 and PAI-2 (which inhibit clot lysis) impedes fibrin removal and clot lysis.102 An increase in the incidence of VTEs is seen during the third trimester when fibrinolytic activity decreases. Finally, the potential for localized vascular damage and release of tissue factor exists with delivery and particularly with cesarean section. Further decreases in protein S after cesarean delivery or with infection may increase the risk of pulmonary emboli postpartum.102 VTE is also increased with obesity, increased parity, maternal age, prolonged bed rest, and in women with thrombophilia, antiphospholipid syndrome, anemia, artificial heart valves, or preeclampsia (due to exaggeration of the hypercoagulable state).102,115,125 Once a thrombus develops, it is more likely that it will extend if the predisposing factors persist over time, as occurs with pregnancy. Women with a history of VTE either before or during pregnancy, especially if associated with thrombophilia, have an increased risk of developing a similar disorder in subsequent pregnancies. Ambulation soon after delivery decreases venous stasis and the risk of VTE.
In pregnant women with VTEs the anticoagulant therapy of choice is usually either unfractionated or low molecular weight heparin. Heparin is considered the drug of choice for thromboembolic disorders during pregnancy because the molecular weight of both of these forms of heparin prevents placental transfer.58,102,119 Heparin doses may need to be increased as pregnancy progresses, particularly during the third trimester. Changes in heparin requirements have been related to increasing plasma volume and renal clearance and to the presence of a placental heparinase enzyme. Heparin doses may need to be decreased in women who develop significant alterations in renal function or in whom plasma volume changes are reduced.102,119 Coumarin derivatives (warfarin sulfate) inhibit vitamin K–dependent coagulation factors. These agents cross the placenta, whereas vitamin K–dependent coagulation factors do not, impairing fetal coagulation and increasing the risk of fetal and neonatal hemorrhage.119 The risk of fetal bleeding and intracranial hemorrhage is especially high during labor. Warfarin has also been associated with an increased risk of abortion and with a specific syndrome of fetal anomalies involving the face, eyes, bones, and central nervous system, if given in the first 11 to 13 weeks of gestation.119,122 These abnormalities may be due to inhibition of vitamin K–dependent proteins (osteocalcins) involved in bone development.
Coagulation disorders during pregnancy
Thrombophilias are being increasingly regarded as having potential roles in the pathophysiology of preeclampsia, fetal growth restriction, and miscarriage due to the effects of thrombotic changes in the placental bed.65,70 Changes in pregnancy may exacerbate the effects of inherited thrombophilias such as the factor V Leiden and prothrombin gene variants and deficiencies of protein C and S and increase the risk of pregnancy complications.103 Women with these disorders may develop clinical signs for the first time during pregnancy.65 The most common acquired thrombophilic disorder is antiphospholipid syndrome (APS), an autoimmune disorder seen in 0.2% to 2% of pregnant women.103,159 In this disorder, antibodies are produced that promote thrombosis. APS involves activation of endothelial cells, monocytes, and platelets by the APL antibodies.159 Women with APS have an increased risk of arterial and venous thrombosis, autoimmune thrombocytopenia, miscarriage, and fetal loss.96,159 Pregnancy or use of estrogen-containing birth control pills increase the risk of thrombosis in women with APS, who are treated with heparin with or without low-dose aspirin.159
Antiphospholipid antibodies such as lupus anticoagulant and anticardiolipin are also seen in pregnancies complicated by preeclampsia, fetal growth restriction, placental insufficiency, placental abruption, and preterm birth.65,103 The increase in fetal loss with antiphospholipid antibodies is thought to be due to an increase in the thromboxane to prostacyclin ratio in the decidua and placenta, leading to increased platelet activation, vasoconstriction, placental thrombosis and infarct, and subsequent fetal death.103
Pregnancy is characterized by increases in fibrinolytic activity, plasminogen and plasminogen activators in the uterus, and an ongoing low-grade activation of the coagulation system within the uteroplacental circulation. As a result, events such as extravasation of blood into the myometrium or rupture of blood vessels in the area can activate the fibrinolytic system and lead to a consumptive coagulopathy.15 The risk of coagulopathies such as disseminated intravascular coagulation (DIC) is higher during pregnancy, particularly in association with placental abruption, severe preeclampsia, eclampsia, intrauterine fetal death, amniotic fluid embolism, or septic abortion.87 These events result in one or more of the processes commonly associated with intravascular coagulation: release of tissue factor and activation clotting (preeclampsia, eclampsia), endothelial injury with activation of coagulation (placental abruption, fetal death), or shock and stasis (amniotic fluid embolism, gram-negative sepsis).87,191 Many of these complications trigger the formation of tissue factor or endotoxins, thus inducing thrombin formation. The resulting activation of the coagulation pathway (see Figure 8-3) leads to depletion of fibrinogen and increased fibrinolytic activity.69
DIC arises from inappropriate activation of normal clotting processes within the circulation with intravascular consumption of procoagulant proteins, clotting factors and platelets, formation of fibrin clots within the vascular bed, production of anticoagulants, and activation of fibrinolysis. As a result, the normal balance between the coagulation and fibrinolytic systems is disrupted. Consumption of the clotting factors can lead to hemorrhage and shock. As clots are formed and fibrin is deposited in the microcirculation, further cell (tissue) injury occurs, triggering further coagulation and eventual depletion of plasma clotting factors. These fibrin clots may also cause intravascular obstruction and infarction. Activation of clotting also activates the fibrinolytic system, which leads to formation of fibrin-fibrinogen degradation products or fibrin split products. These fibrin degradation products further inhibit coagulation and decrease platelet function.202
Summary
The hematologic and hemostatic systems undergo significant alterations during pregnancy that promote maternal adaptation but also influence interpretation of laboratory values and increase the risk of thromboembolic insults and coagulopathies. Because of the significant risks associated with these events, appropriate measurement, observation, data gathering, and evaluation are essential. Changes in the hematologic system during pregnancy are also critical for fetal homeostasis. Maternal hypervolemia promotes delivery of oxygen and nutrients to the fetus; changes in serum albumin concentration may influence the availability of both nutrients and potentially harmful substances. The fetus can, in turn, affect maternal status, as is the case with iron metabolism and needs. Clinical recommendations for nurses working with pregnant women based on changes in the hematologic system are summarized in Table 8-5.
Table 8-5
Recommendations for Clinical Practice Related to Changes in the Hematologic System of Pregnant Women
Recognize usual hematologic values and patterns of change during pregnancy and postpartum (pp. 216-225 and Tables 8-1 to 8-3).
Recognize that isolated laboratory values must be evaluated in light of clinical findings and previous values (pp 216-225).
Assess maternal nutritional status in relation to iron, folate, and vitamins, and provide nutritional counselling (pp. 225-227).
Monitor hematocrit and hemoglobin values throughout pregnancy (pp. 218-219, 225-227).
Know the patterns of change in plasma and blood volume during pregnancy and the postpartum period (pp. 216-217, 223-224).
Monitor and counsel women with cardiac problems, paying particular attention to periods when blood and plasma volume increases significantly (pp. 216-217, 223-224 and Chapter 9).
Monitor for and teach pregnant woman to recognize the signs of thromboembolism and consumptive coagulopathy (pp. 228-229).
Recognize risk factors for the development of thromboembolism (p. 228).
Encourage ambulation soon after delivery to reduce the risk of thromboembolism (p. 228).
Recognize risk factors for development of consumptive coagulopathies (p. 229).
Evaluate maternal responses to prescribed drugs for signs of subtherapeutic levels or side effects (p. 220 and Chapter 7).
Counsel women regarding the effects of plasma volume increases and decreased plasma proteins on drug levels during pregnancy (pp. 216-217, 220 and Chapter 7).
Recognize factors that may alter signs of infection during the intrapartum and early postpartum period (pp. 223-224).
Note the timing of cord clamping (pp. 234-235).
Development of the hematologic system in the fetus
The hematologic system arises early in gestation and along with the primitive cardiovascular system is one of the earliest systems to achieve some functional capacity. The hematologic system is critical for the well-being of the fetus through transport of nutrients and oxygen and removal of waste products. Fetal red blood cells (RBCs), white blood cells (WBCs), and platelets are often found in the maternal circulation. Fetomaternal transfusion occurs in an estimated 50% of pregnancies, although this generally involves a small volume. RBCs can pass as early as 4 to 6 weeks. Later, antigens on some of these cells may stimulate maternal antibody production against the fetal cells, which can lead to fetal anemia, neutropenia, or thrombocytopenia.30 Fetal iron requirements were discussed in the previous section.
Formation of blood cells
Blood cells first appear in the yolk sac and can be found as early as 2 weeks, gestation.106 By about 6 weeks’ gestation, hematopoietic stem cells are found in the developing liver and migrate from there to the bone marrow.209 Hematopoiesis in the fetus can be divided into three periods: mesoblastic, hepatic, and myeloid. In all sites, cells arise from stem cells derived from mesoderm tissues. Primitive cells arise first, followed by definitive cells. Embryonic and fetal hematopoietic stem cells are pluripotent cells that can reproduce and repopulate in adults better than adult stem cells. Thus these fetal cells have greater potential for transplant with lower risk of graft failure.33,47,209 Embryonic and fetal stem cells also have the ability to differentiate into cells of different tissues types, not just hematopoietic cells, depending on environment.141
During the mesoblastic period (from 14 to 19 days to a peak at 6 weeks’ gestation), blood cells are formed in blood islands in the secondary yolk sac. The secondary yolk sac arises at 12 to 15 days and is a site of early protein synthesis, nutrient transfer, and hematopoiesis. Peripheral cells in these islands form primitive blood vessels; central cells develop into hematoblasts (primitive RBCs).33,148 Although only RBCs and macrophages are produced in the yolk sac, the yolk sac stem cells are all multipotential.67,81 Blood formation in the yolk sac is maximal from 2 to 10 weeks and disappears by the third month.3,48 Another site of early blood formation during this period is the ventral aspect of the aorta in the periumbilical area. Hematopoiesis occurs in this area until 28 to 40 days’ gestation.33,81,187
The hepatic period begins during the fifth to sixth week, shortly after the onset of circulation during weeks 4 to 5. This period peaks from 6 to 18 weeks. Thus from 3 to 5 months the liver is the major source of fetal blood cells, although some blood formation continues in this site through the first week after birth. Liver mass increases 40-fold after liver hematopoiesis begins, and at 11 to 12 weeks’ gestation, hematopoietic cells make up 60% of the liver.47,81 The predominant cells produced by the liver are normoblastic erythrocytes, although megakaryocytes, granulocytes, and lymphocytes are also produced.148 Hematopoiesis can be detected in the spleen, omentum, and thymus during the third month and shortly thereafter in the lymph nodes. Blood formation in the spleen, initially producing erythrocytes and later lymphocytes, declines after 4 to 5 months’ gestation.207
Extramedullary erythropoiesis may continue in the liver and spleen and develop in other organs, including the adrenal glands, pancreas, thyroid, endocardium, skin, and brain, after bone marrow is established.81 This may occur with any event resulting in reduced bone marrow function, as can occur with rubella or parvovirus B19 infection. When this occurs in the skin, it can been seen as the “blueberry muffin” rash.81
The thymus and lymph nodes become seeded with stem cells by the fourth month. These structures are primarily involved in lymphopoiesis but are also involved in maturation and activation of the immature WBCs that develop from myeloid stem cells.33,140
The myeloid period begins at about 18 weeks, with eventual production of all types of blood cells.3,47,81 The bone marrow is the major site for blood production after 30 weeks’ gestation.3,81 Centers for blood formation arise in mesenchymal tissues and invade cavities produced during bone formation (see Chapter 17). Initially bone marrow produces granulocytes and megakaryocytes. After liver erythropoiesis declines, bone marrow erythropoiesis increases. The major area of blood production is the fatty marrow in the core of the long bones. Large fat cells are not found in fetal marrow as they are in adults, suggesting that fetal marrow is functioning at full hematopoietic capacity under homeostatic conditions.
The long bones of the fetus and newborn are cartilaginous, and their relative marrow volume is smaller than in older individuals. As a result, the only way the fetus or newborn can significantly increase production of blood cells is by either reactivation or persistence of extramedullary hematopoiesis in the abdominal viscera, such as the liver and spleen. This extramedullary erythropoiesis is responsible for much of the hepatosplenomegaly seen in infants with erythroblastosis fetalis.53
All blood cells arise from pluripotent stem cells under the influence of various cytokines and growth factors for specific cell lineages (Figure 8-7). Fetal and post birth hematopoiesis are mediated by hematopoietic growth factors and cytokines, including stem cell factors, interleukin(IL)-1, IL-4, IL-6, IL-9, erythropoietin (Epo), macrophage colony-stimulating factor (M-CSF), granulocyte colony-stimulating factor (G-CSF), thrombopoietin, and possibly insulin and insulin-like growth factors.31,47,81,106,148 Hematopoietic growth factors are either non–cell-lineage– or cell-lineage–specific. Non–cell-lineage–specific growth factors—such as IL-3 and granulocyte-macrophage colony-stimulating factor (GM-CSF)—stimulate a variety of progenitor cells. Cell-lineage–specific growth factors stimulate differential maturation of granulocytes (G-CSF), monocytes (M-CSF), or erythrocytes.81,106 Epo and other hematotpoietic growth factors also appear to be important in development of other systems (including cardiovascular, gastrointestinal, and brain) both before and after birth.67,79,80,92,186,205
Development of red blood cells
Fetal RBC production is independent of the mother. The initial RBCs are primitive nucleated megaloblasts that appear at 3 to 4 weeks’ gestation, followed by normative megaloblastic erythropoiesis at 6 weeks. The primitive cells contain embryonic hemoglobin and are not dependent on Epo. The definitive fetal RBC, produced initially in the liver, contains primarily fetal hemoglobin (HbF) and is regulated by Epo.47,148 By 10 weeks, the latter cells constitute 90% of the RBC volume. The early cells are large nucleated cells with increased deformability. With increasing gestation, hemoglobin, hematocrit, and total RBC count increases, whereas numbers of nucleated RBCs, mean corpuscular volume, cell diameter, mean corpuscular hemoglobin volume, reticulocytes, and proportion of immature cell forms decrease.81,140 Yolk sac cells differentiate intravascularly, while erythoblasts in the liver and bone marrow mature attached to macrophages in blood islands.142
Epo does not cross the placenta, so fetal erythropoiesis is endogenously controlled.33,81 Fetal Epo increases from 19 weeks to term and is produced primarily in the liver, and also in the spleen, rather than in the kidneys. Increasing amounts of Epo are produced in the kidneys from 20 weeks, and especially from 30 weeks to term as the kidneys mature.47,81 The shift from liver to renal Epo production after 30 weeks is thought to be due to increased Epo expression in interstitial cells along with renal growth and maturation. Epo inhibits the death of erythroid precursors and stimulates development and differentiation of these precursors. Epo levels are elevated with fetal hypoxia, anemia, and placental insufficiency and in infants of insulin-dependent diabetic mothers.47
Development of white blood cells and platelets
Formation of WBCs begins in the liver at 5 weeks, although a few macrophages are produced in the yolk sac, followed by the thymus (8 to 9 weeks), spleen (11 weeks), and lymph nodes (12 weeks).81 Significant numbers are not produced until the myeloid period. Initially, erythropoiesis is greater than granulopoiesis; however, by 10 to 12 weeks, granulopoiesis predominates, and by 21 weeks the adult ratio of granulopoiesis to erythropoiesis is seen.208 Circulating granulocytes increase rapidly during the third trimester. The neutrophil count doubles from 14 to 18 weeks, gestation and increases four-fold from 24 to 32 weeks.110 At birth, numbers of WBCs are equal to or greater than those found in adults. Eosinophils appear by 10 weeks, increasing to 5% of total marrow cells by 21 weeks. Basophils also appear by 10 weeks, but levels remain low. Monocytes are found in the yolk sac by 3 to 4 weeks and initially are the most prominent cells in liver hematopoietic tissue, then decrease to birth. Circulating monocytes are seen at 5 to 6 months, gestation.89 Macrophage levels peak at 10 to 16 weeks; monocyte levels peak by 12 to 16 weeks.208
Lymphocytes are formed in the fetal liver and lymphoid plexuses initially, then in the thymus (7 to 10 weeks), and spleen and bone marrow (10 to 12 weeks).81 The numbers of circulating lymphocytes increase rapidly to peak at 20 weeks (10,000 mm3), then decline to 3000/mm3 by term.140 Megakaryocytes are found at 5 to 6 weeks in the yolk sac, by 8 to 9 weeks in the circulation, in the liver and spleen by 10 weeks, and reach adult levels by 18 weeks.148,177 Platelets increase from a mean of 187,000/mm3 at 15 weeks to a mean of 274,000 by term.143,175 Thrombopoietin, produced primarily in the liver and kidneys, is the major factor controlling platelet production.81
Formation of hemoglobin
Hemoglobin synthesis begins around 14 days’ gestation. Several forms of hemoglobin (Hb) are found in the embryo and fetus (Figure 8-8). Two pairs of polypeptide chains form each of the different hemoglobin types (Table 8-6). All forms have two α or α-like globulin chains (controlled by genes on chromosome 16). The primitive embryonic varieties formed in the yolk sac are Gower 1 (seen at less than 5 weeks’ gestation), followed by Gower 2 and Hb Portland (seen between 5 and 10 to 12 weeks’ gestation).47,148 Two points of hemoglobin switching occur during development, the first is the switch from embryonic hemoglobin to fetal hemoglobin (HbF); the second is the switch from HbF to adult hemoglobin (HbA).16
Table 8-6
Human Hemoglobins Expressed During Development
HEMOGLOBIN (Hb) | GLOBULIN CHAIN COMPOSITION | STAGE OF EXPRESSION | PRIMARY SITE OF PRODUCTION |
Hb Gower 1 | Zeta2 epsilon2 (ζ2ε2) | Embryonic | Yolk sac |
Hb Gower 2 | Alpha1 epsilon2 (α1ε2) | Embryonic | Yolk sac |
Hb Portland | Zeta2 gamma2 (ζ2γ2) | Embryonic | Yolk sac |
HbF | Alpha2 A gamma2 or alpha2G gamma2 | Fetal | Liver |
HbA2 | Alpha2 delta2 (α2δ2) | Adult | Bone marrow |
HbA | Alpha2 beta2 (α2β2) | Adult | Bone marrow |
From Luchtman-Jones, L., Schwartz, A.L., & Wilson, D.B. (2006). Hematological problems in the fetus and neonate. In R.J. Martin, A.A. Fanaroff, & M.C. Walsh (Eds.). Fanaroff & Martin’s Neonatal-perinatal medicine: Diseases of the fetus and infant (8th ed.). Philadelphia: Mosby.
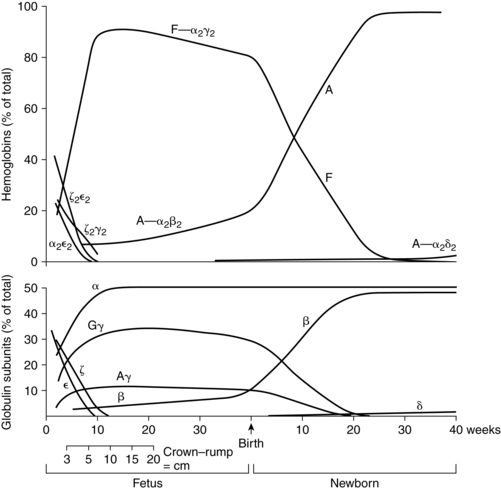
The predominant hemoglobin from 10 to 12 weeks to term is HbF, which consists of a pair of α chains and a pair of γ chains. HbA, which consists of pairs of α and β chains (controlled by genes on chromosome 11), appears after 6 to 8 weeks and increases rapidly after 16 to 20 weeks’ gestation.140 By 30 to 32 weeks, 90% to 95% of the hemoglobin is HbF. After this point, the amount of HbF begins to slowly decline to 84% at 34 weeks and 60% to 80% by term.146 Simultaneously, levels of HbA increase along with the total body hemoglobin mass.140 The switch from fetal to adult hemoglobin synthesis is related to postmenstrual, not post birth, age. Synthesis of HbA and HbF is not significantly affected by intrauterine transfusions or exchange transfusions after birth.
All forms of hemoglobin have similar functions but vary in their oxygen affinity, with earlier forms having greater affinity.14,47 HbF has a greater affinity for oxygen because HbF does not bind 2,3-diphosphoglycerate (2,3-DPG) as effectively as does HbA. Increased affinity facilitates oxygen transfer across the placenta but reduces oxygen release to the tissues. Fetal hemoglobin has several other unique properties. HbF is more resistant to acid elution and can be oxidized to methemoglobin more readily, increasing the susceptibility of newborns to methemoglobinemia.
The resistance of fetal hemoglobin to acid elution is the basis for tests such as the Kleihauer-Betke techniques used to detect fetal cells in maternal blood. These tests may be unreliable as a measure of fetal cells if maternal HbF is elevated or with ABO incompatibility. In the presence of ABO incompatibility, fetal cells may be destroyed by maternal antibody and cleared from the mother’s circulation. Altered levels of HbF are seen in women with sickle cell anemia, thalassemia minor, hydatidiform mole, and leukemia and in a pregnancy-induced elevation of HbF. Fetal cells may still be identified, because with elevated maternal HbF there tend to be many cells with varying amounts of HbF and the fewer fetal cells have consistently high HbF concentrations.53
Fetal iron requirements
Fetal iron content is 75 mg/kg by the third trimester.153 The majority (75%) of fetal iron is found in hemoglobin, with about 7 mg/kg in tissues and 10 mg/kg stored in the liver and spleen.152 The stored iron doubles during the last few weeks of gestation. Iron passes rapidly against a concentration gradient from mother to fetus via transferrin. Maternal transferrin releases its iron in the intervillous space. The iron is taken up by transferrin receptors located on the surface of syncytiotrophoblast cells and is actively transported across the syncytiotrophoblast and cytotrophoblast by a series of iron transporters.87,152,210 Iron transported across the placenta enters fetal blood and attaches to fetal serum transferrin. Fetal transferrin is synthesized by the fetal liver beginning about 29 to 30 days’ gestation.140
By the end of pregnancy, 90% of the mother’s transferrin-bound iron is delivered to the placenta.152 Placental transferrin receptor density is thought to be the major determinant of placental iron transfer.210 Increased expression of placental transferrin receptors is seen with low maternal stores, low fetal stores, and with adolescents (who have increased iron needs due to their own growth and development).210 This compensatory mechanism enhances uptake of iron by the placenta. Other mechanisms to decrease the risk of fetal iron deficiency are increased maternal intestinal iron absorption and increased placental iron transport.87
With fetal growth the rate of iron transfer across the placenta increases. Thus transport of iron from mother to fetus is greatest during the last few months of gestation (up to 4 mg/day at term).152 Serum iron and ferritin levels in cord blood are higher than in maternal blood and have been related to maternal hemoglobin or ferritin in most but not all studies. Cord blood serum ferritin levels are lower (but usually still within normal limits) in infants of iron-deficient mothers, reflecting lower fetal iron stores. These infants are reported to have reduced iron stores and an increased risk of anemia in the first year.152
Development of the hemostatic system
The hemostatic and fibrinolytic systems develop simultaneously. Most coagulation proteins are present in measurable quantities by 5 to 10 weeks’ gestation.56,91 Fetal blood demonstrates clotting ability by 11 to 12 weeks.69 Fibrinogen synthesis in the liver begins at 5 weeks. Fibrinogen can be found in the plasma by 12 to 13 weeks, reaching adult values by 30 weeks.180 The placenta is rich in tissue factor (TF), which also plays a role in angiogenesis, cell signaling, and embryogenesis. Thus the placenta is able to respond rapidly to insults with initiation of the clotting cascade.18,63,69,166 This helps protect the fetus from significant blood loss. Vitamin K levels are 30% of adult values by the end of the second trimester and 50% by term.180
Fibrinolytic activity can be demonstrated at 12 to 13 weeks’ gestation. The whole blood clotting time in the fetus is relatively short, and the fetus is in a hypercoagulable state during the first and second trimesters. Fibrinolytic activity in the fetus is increased even though levels of blood plasminogen are low, due to increased tissue plasminogen activator (tPA) or decreased inhibitor or both.69 The elevated fibrinolytic activity may help protect and maintain the extensive fetal capillary circulation within the placental villi.