Head injury
Clinical aspects
Assessment and management
The best indicator of head injury severity is impairment of consciousness. This either reflects brain stem dysfunction or diffuse hemispheric damage. Level of consciousness is assessed using the Glasgow Coma Scale (GCS) which quantifies responses to verbal and painful stimuli in terms of eye opening, speech and movement (Fig. 9.1). The aggregate score ranges from a maximum of 15 (alert and orientated) to a minimum of 3 (comatose or dead).
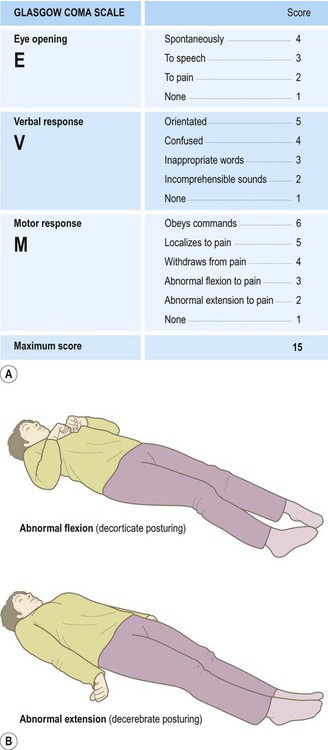
(A) Response to verbal and painful stimuli is assessed in terms of eye opening, verbal response and motor response. The minimum score is 3 (even in death) and the maximum score is 15 (alert and orientated); (B) Abnormal flexion to pain (an ‘M’ score of 3 out of 5) is also known as decorticate posturing, whereas abnormal extension to pain (an ‘M’ score of 2 out of 5) is called decerebrate posturing. From Teasdale, G and Jennett, B: LANCET (ii) 81-83: (1974) with permission.
A GCS score of 13–15 corresponds to mild head injury, accounting for the majority of cases. A total score of 9–12 represents moderate head injury, whilst a score of 3–8 signifies severe head injury. In addition to providing an initial assessment of severity, any reduction in the GCS score is a sensitive indicator that the clinical state has deteriorated. This may signify development of a secondary complication requiring urgent intervention. The role of imaging in the assessment of head injury is discussed in Clinical Box 9.1.
Outcome following head injury
Factors that predict a less favourable outcome include increasing age (>60 years), an initial GCS score below 5, a fixed and dilated pupil, a prolonged period of hypotension/hypoxia or a haemorrhage requiring surgical decompression. Late complications may include hydrocephalus due to obstruction of CSF drainage pathways (see Ch. 2, Clinical Box 2.2) or seizures (Clinical Box 9.2).
Pathology of head injury
Cerebral contusions
Cerebral contusions (bruising) and lacerations (tears) are common in traumatic brain injury, occurring in more than 90% of fatal cases. Contusions are most pronounced at the crests of gyri in the frontal and temporal lobes, particularly in places where the brain comes into contact with the irregular contours of the skull base (Fig. 9.2). Cerebral contusions may be associated with haemorrhage into the overlying subarachnoid or subdural spaces and if blood continues to accumulate it will begin to act as an intracranial mass lesion. The combination of a cerebral contusion with an overlying subdural haemorrhage is called a burst lobe. This most often occurs at the frontal and temporal poles.
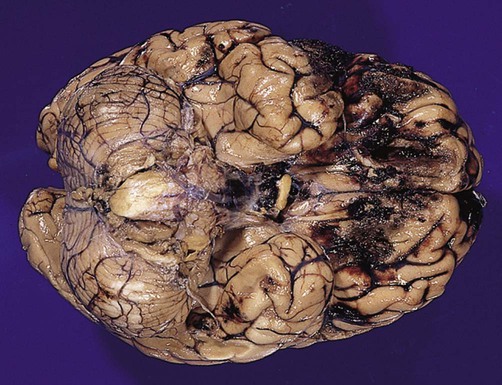
They are particularly marked in the orbital cortex and frontal/temporal polar regions. From Prayson, R: Neuropathology 1e (Churchill Livingstone 2005) with permission.
Intracranial haemorrhage
Extradural haemorrhage
This typically occurs when the middle meningeal artery is torn by a skull fracture in the region of the pterion, where the bone is thin and is closely related to the underlying vessel (Fig. 9.4). Arterial blood escapes into the extradural space (between dura and bone) and strips the tightly adherent dura from the inner table of the cranial vault (Fig. 9.5).
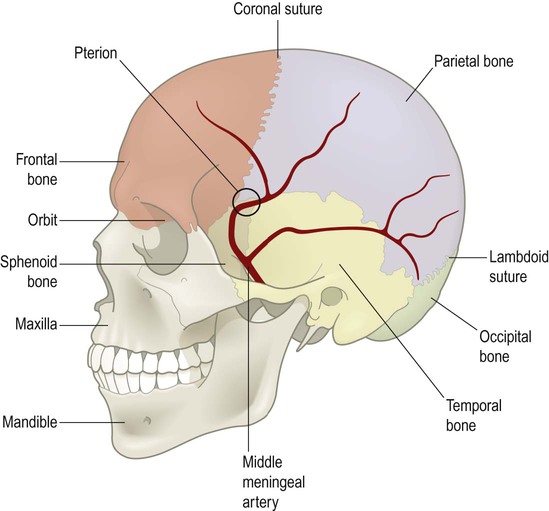
The underlying vessel is therefore vulnerable to damage in association with a skull fracture.
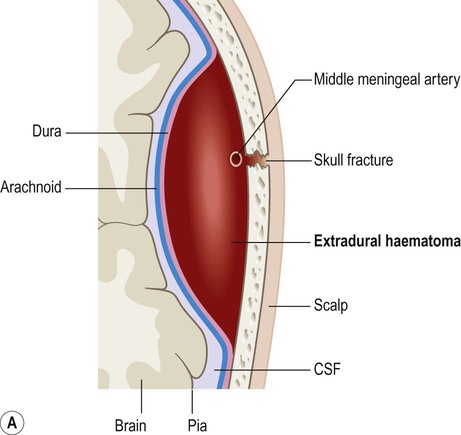
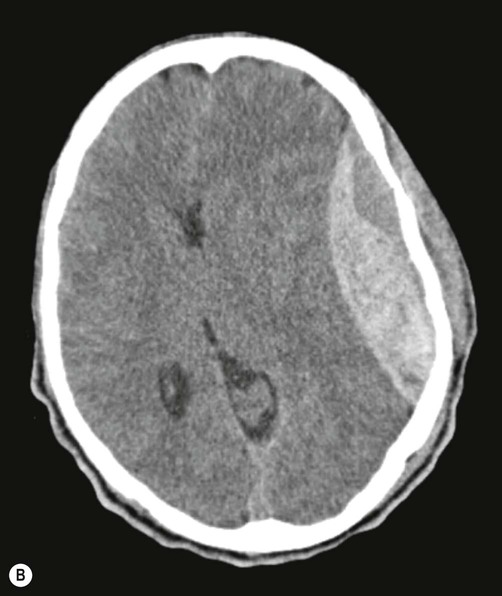
On brain imaging, the typical biconvex shape is due to the fact that the dura is firmly anchored to the overlying skull and the accumulating blood is not able to cross suture lines (between adjacent cranial bones). Note the considerable ‘midline shift’ caused by the expanding mass lesion. Courtesy of Dr Andrew MacKinnon.
Subdural haemorrhage
Subdural haemorrhage is caused by tearing of bridging veins that pass between the cerebral cortex and dural venous sinuses (see Ch. 1). These vessels pass through a potential plane between the dura and arachnoid membranes. A subdural haematoma results from the accumulation of venous blood in this potential space, which is easily expanded by blood under venous pressure because the dura and arachnoid are only loosely attached to each other (Fig. 9.6).
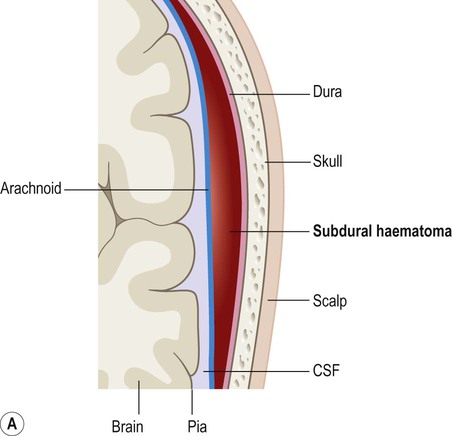
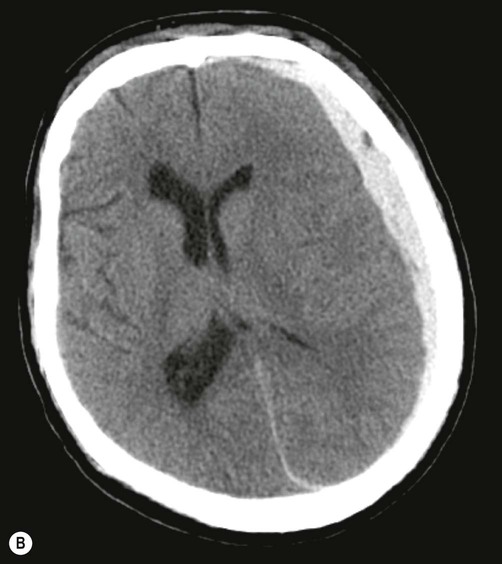
On brain imaging, subdural haematomas spread further over the surface of the brain and cause less mass effect. This is because the blood is able to track freely between the arachnoid and dura (within the subdural space). Courtesy of Dr Andrew MacKinnon.
Diffuse axonal injury
Microscopic appearances
Diffuse axonal injury can only be detected reliably by microscopic examination of the brain after death. This shows axonal swellings throughout the cerebral hemispheres and brain stem (Fig. 9.7). Large white matter bundles such as the corpus callosum and internal capsule are particularly vulnerable, together with the dorsolateral sector of the upper brain stem.
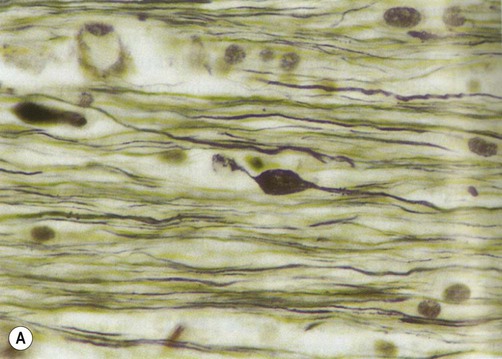
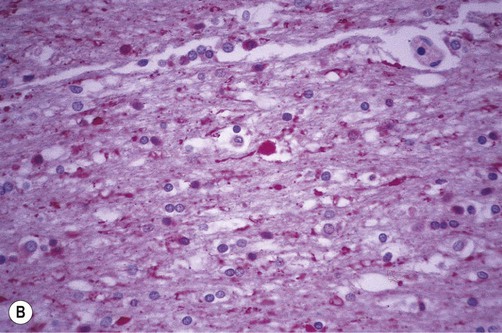
(A) Axon swellings, demonstrated using silver staining. From Stevens and Lowe: Pathology 2e (Mosby 2000) with permission; (B) Antibody-labelling (immunohistochemistry) for beta-APP. From Prayson, R: Neuropathology 1e (Churchill Livingstone 2005) with permission.
Axonal swellings can be highlighted by silver stain preparations (Fig. 9.7A). They can also be demonstrated by immunohistochemistry (antibody-labelling) of proteins that are normally transported along axons, including beta amyloid precursor protein (β-APP). Positive labelling for β-APP can be seen within two hours of head injury (Fig. 9.7B).
In the most severe cases, axonal transection is accompanied by tears in small blood vessels, leading to microhaemorrhages. These form small haematomas at the junction between grey and white matter (Fig. 9.8). Confusingly, these small haemorrhages are known as gliding contusions.
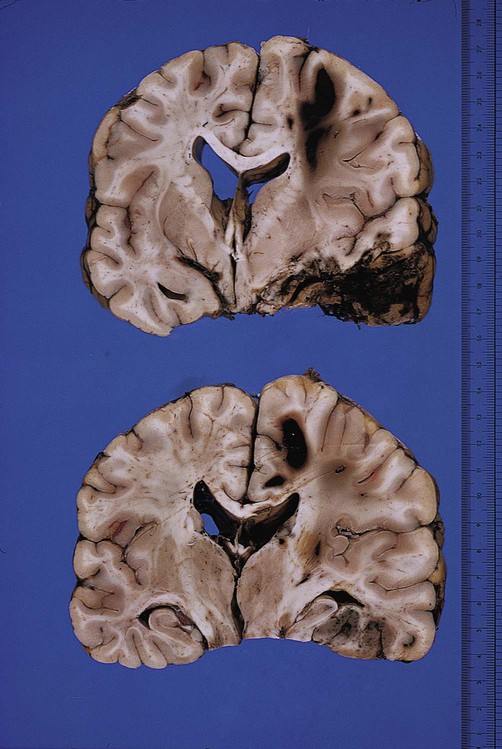
Post-mortem photograph in a case of traumatic head injury. The upper and lower images show large gliding contusions in the parasagittal white matter, close to the overlying cortex. There is also severe contusion of the temporal lobe. From Ellison and Love: Neuropathology 2e (Mosby 2003) with permission.
Brain swelling and intracranial pressure
Raised intracranial pressure
The cranium is a rigid structure with non-compressible contents (Fig. 9.10A). Any increase in the total volume of the cranial contents will therefore lead to a steep rise in intracranial pressure, but this is initially prevented by compensatory mechanisms which modify the volume–pressure curve (Fig. 9.10B). These include redistribution of CSF from the ventricles to the lumbar subarachnoid space and compression of the dural venous sinuses (thereby reducing the total intracranial blood volume).
Brain shift and herniation
The cranial cavity is incompletely divided into compartments by two dural partitions: the falx cerebri (in the midline) and the tentorium cerebelli (forming a roof over the posterior fossa) (see Ch. 1). A pressure gradient may be created by an expanding mass lesion, displacing part of the brain from one intracranial compartment to another. This is termed brain shift (or internal herniation).
Types of herniation (Fig. 9.11)
Displacement of the cingulate gyrus under the free edge of the falx cerebri is termed subfalcine herniation and usually results from a focal lesion in one cerebral hemisphere (see Figs 9.11a and 9.12a).
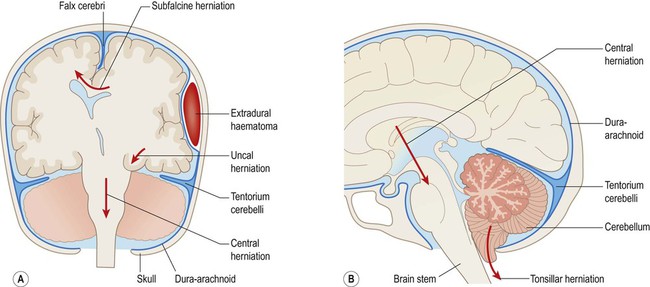
(A) Coronal section illustrating the main types of internal herniation; (B) Sagittal section of the posterior fossa showing tonsillar and central (diencephalic) herniation.
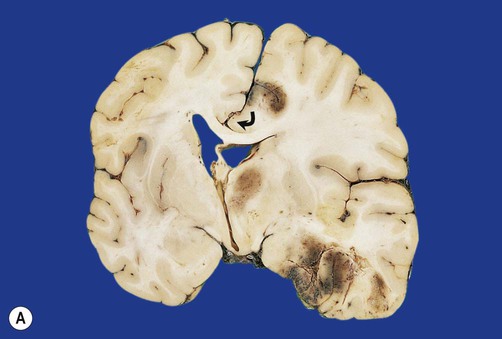
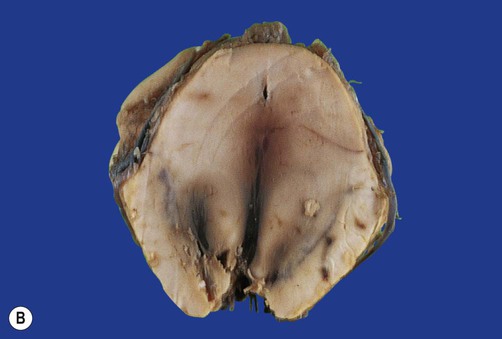
(A) Coronal section showing subfalcine herniation of the right cingulate gyrus (arrow) with marked midline shift. There is also haemorrhage in the medial temporal region due to transtentorial herniation; (B) Displacement of the brain stem may lead to a fatal midline haemorrhages (Duret or ‘flame’ haemorrhages), shown here in the midbrain. From Ellison and Love: Neuropathology 2e (Mosby 2003) with permission.
Downward displacement of the medial temporal lobe (the parahippocampal gyrus and uncus) through the tentorial hiatus is referred to as transtentorial (or uncal) herniation (Fig. 9.11A). This may cause pupil changes due to compression of the oculomotor nerve (Clinical Box 9.3).
Diffuse swelling of both cerebral hemispheres is likely to produce downward displacement of the entire diencephalon (thalamic region). This is called diencephalic (or central) herniation and may be associated with tearing of brain stem blood vessels, causing fatal haemorrhage (Fig. 9.12B).
Secondary infarction
In addition to the direct effect of cerebral herniation, which damages the compressed brain tissue, obstruction of cerebral blood vessels may lead to secondary infarction (tissue death due to impaired blood flow; see Ch. 10). For instance, subfalcine herniation may be associated with compression of the anterior cerebral artery with consequent infarction of the medial frontal lobe, whereas transtentorial herniation may obstruct the posterior cerebral artery, with infarction of the occipital and inferior temporal lobes.
Brain blood flow and ICP
The main factors determining cerebral blood flow (CBF) are illustrated in Figure 9.13. Blood flow to the brain is proportional to the cerebral perfusion pressure (CPP) and inversely proportional to the cerebral vascular resistance (CVR). The cerebral perfusion pressure can be thought of as the ‘driving force’ for cerebral blood flow.
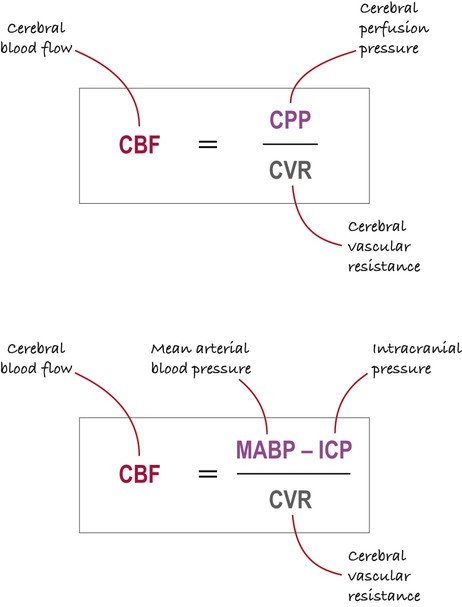
NB: The upper and lower expressions are the same, but the lower part of the figure shows the main determinants of the cerebral perfusion pressure (coloured purple).
Regulation of brain blood flow
The cerebral blood flow is normally maintained at a constant level in a process termed autoregulation. With normal intracranial pressure, flow depends mainly upon the mean arterial blood pressure, which is approximately equal to the diastolic blood pressure plus one third of the pulse pressure (the ‘pulse pressure’ is the difference between systolic and diastolic pressures). The brain compensates for fluctuations in the arterial perfusion pressure by altering the vascular resistance of the brain, achieved by contraction and relaxation of smooth muscle in cerebral arterioles. Autoregulation is effective over a very wide range of mean arterial blood pressures (see Fig. 9.14). Following head injury the normal autoregulatory mechanisms may not be effective, so that relatively small falls in perfusion pressure (caused by low blood pressure or high intracranial pressure) may be associated with precipitous drops in cerebral blood flow. Management of raised ICP is discussed in Clinical Box 9.4.
Minor head injury
Concussion
Concussion is thought to be caused by acceleration–deceleration injury leading to shearing forces and diffuse axonal dysfunction. Pathological studies show that concussion may be associated with lasting changes in the brain and these may be responsible for the long-term alterations in mood, memory and concentration that occur in some people (Clinical Box 9.5).
Repeated minor head injury
There is increased risk of cumulative mild traumatic brain injury in certain sporting activities. It is well known in boxing as the ‘punch drunk’ syndrome, but may also occur in other sports such as football or horse racing. Examination of the brain in such cases often reveals signs of old contusions (especially in the frontal and temporal lobes) together with generalized cerebral atrophy, enlargement of the ventricles and tearing of the septum pellucidum. Microscopic examination may show neuronal loss in the cerebral cortex and substantia nigra of the midbrain. Surviving neurons often contain neurofibrillary tangles similar to those found in Alzheimer’s disease (Ch. 12); and selective loss of neurons in the substantia nigra may lead to features mimicking Parkinson’s disease (Ch. 13).