17 Head and Neck
Diagnostic Imaging Techniques
Computed Tomography
CT makes use of ionizing radiation to generate cross-sectional images based on differences in x-ray attenuation of various tissues. Modern scanners are typically helical, meaning that x-ray source rotation and patient translation occur simultaneously, resulting in the acquisition of a volume of data that is then partitioned and reconstructed into individual slices.1 Helical scanning allows for rapid data acquisition to diminish artifacts related to motion (breathing, swallowing, gross patient motion). The rapid data acquisition also allows for more and thinner slices to be obtained, which facilitates diagnosis by decreasing partial volume averaging effects. It also allows for improved quality of multiplanar reconstructions. The most recent advance in CT imaging has been the introduction of the multidetector or multislice scanners.2,3 Multidetector scanners have a variable number of parallel arcs of detectors that are capable of simultaneously acquiring volumes of data. The increased speed that results from multislice sampling can be traded for improved longitudinal resolution, increased volume of coverage, and improved signal-to-noise ratio.
CT of the head and neck should be performed with thin sections, generally ≤3 mm, in the axial plane (Fig. 17-1A). Direct coronal imaging may be performed in some situations (such as paranasal sinus imaging), but multidetector methods allow for high-quality coronal and sagittal reformations (Fig. 17-1B to Fig. 17-1E); reformatted images improve depiction of anatomical relationships and can improve diagnostic accuracy. They also spare the patient from additional radiation. Additional angled views can also be useful in specific situations (e.g., to throw artifacts related to dental amalgam off of vital structures) (Fig. 17-1F to Fig. 17-1H). Unless one is primarily interested in bony structures, CT of the head and neck is usually performed following injection of iodinated contrast material. The opacification of vessels helps to separate them from other structures such as lymph nodes and also helps to delineate pathologic conditions. Iodinated contrast material carries a small risk of minor or significant allergic reaction and a risk of nephrotoxicity in patients who already have some degree of renal dysfunction or are at risk of this (e.g., diabetics). These factors should be considered when a contrast-enhanced CT scan is requested.
FIGURE 17-1 • A, Axial 2.5-mm-thick slice from a contrast-enhanced computed tomography (CT) scan of the neck. Note the excellent depiction of the normal larynx, with the true vocal cords (V), cricoid cartilage (C), arytenoid cartilage (black arrow), and thyroid cartilage (white arrow) indicated. Also shown are the common carotid artery (cc) and internal jugular vein (ijv). B, Coronal reformation of the axial CT data demonstrates the true vocal cord (V), false vocal cord (*), and laryngeal ventricle (arrow). C, A more posterior coronal reformation demonstrates the epiglottis (white arrow), pyriform sinus (P), sphenoid sinus (SS), and nasopharyngeal airway (NP). D, An off-midline sagittal reformation shows the sternocleidomastoid muscle (SCM) and subcutaneous fat (FAT) in the posterior cervical space as well as small, normal-appearing lymph nodes (arrows). E, A midline sagittal reformation shows the nasopharynx (NP), epiglottis (white arrow), fat-filled pre-epiglottic space (PES, white arrow), and cervical segment of the trachea (T) and air in the cervicothoracic segment of the esophagus (E). F, An axial contrast-enhanced CT scan at the C1-2 level from a patient with left otalgia shows extensive streak artifact from dental amalgam and no convincing abnormality. G, An axial contrast-enhanced CT scan from the same patient as FIGURE 17-1F in which the slices have been angled to avoid the oral cavity. A mass (white arrows) arising from the deep lobe of the left parotid (P) is now easily appreciated. The right parotid (P) is also indicated. H, Scout view from the patient in FIGURE 17-1F and FIGURE 17-1G shows the angled image stack (red arrows) as well as the standard axial acquisition.
Magnetic Resonance Imaging
MRI exploits differences in tissue relaxation characteristics and spin density to produce an image that is exquisitely sensitive to soft-tissue contrast.4,5 Depending on the parameters that one selects, variable tissue characteristics and contrast will be produced. Multiple types of sequences in multiple planes are generally necessary to fully characterize lesions of the head and neck. Slice thickness should be no more than 5 mm, and a gadolinium-based contrast agent is generally used to enhance detection of pathologic conditions and improve tissue characterization and differential diagnosis. In some circumstances, thinner sections covering a smaller anatomic area may be necessary for more precise depiction and diagnosis.
In the head and neck, we typically obtain the following imaging sequences:
On a T1-weighted image, fat is bright and fluid (such as cerebrospinal fluid) is relatively dark. Muscle and most pathologic conditions are of intermediate signal intensity. The large amount of fat in the head and neck provides intrinsic tissue contrast, which makes the T1-weighted image very sensitive to infiltrative processes that obliterate tissue planes or replace marrow fat (Fig. 17-2A). Some hemorrhagic or proteinaceous lesions may result in intrinsic T1-shortening such that they appear bright on a T1-weighted image. On a T2-weighted image, fluid is very bright and most pathologic conditions are relatively bright, whereas muscle is quite dark. The FSE technique is useful in limiting artifacts related to motion and magnetic susceptibility as compared with a conventional spin-echo T2-weighted image. Because fat remains bright on a FSE image, however, fat saturation should ideally be applied. In the nasal cavity and paranasal sinuses, T2-weighted images are particularly useful in distinguishing neoplastic masses from polyps, thickened mucosa, and retained secretions (Fig. 17-2B). Gadolinium is useful for demonstrating pathologic conditions and tailoring a differential diagnosis based on enhancement characteristics. Postgadolinium imaging is also useful in assessing perineural spread of tumor, cavernous sinus invasion, and meningeal or brain infiltration (Fig. 17-2C and Fig. 17-2D). Fat saturation should ideally be applied on a postgadolinium image; otherwise, the contrast between an enhancing lesion and surrounding fat may actually be reduced as compared with the pregadolinium image. As low-field scanners often do not have fat saturation available, high-field (≥1.5 T) imaging is generally preferred for patients with head and neck cancer. If a patient is severely claustrophobic, then sedation may be necessary to accomplish the scan.
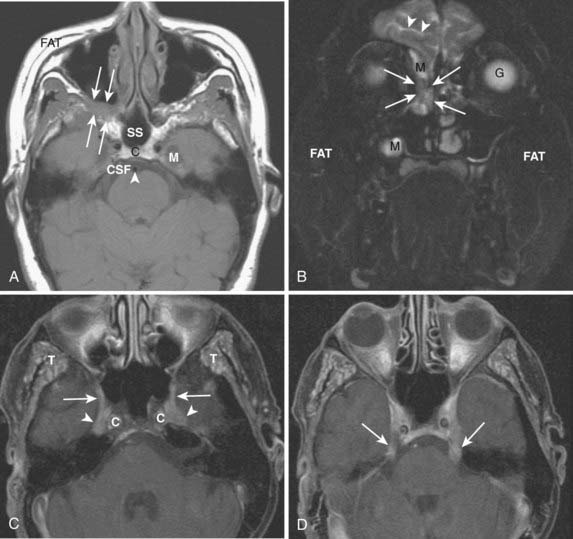
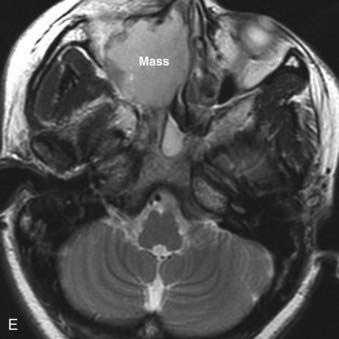
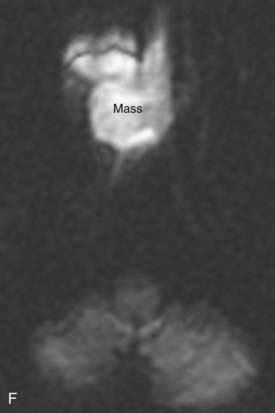
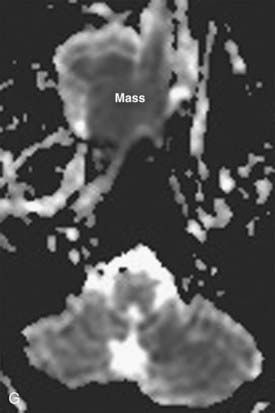
FIGURE 17-2 • A, An axial T1-weighted image is shown. Note that fat (as seen in the subcutaneous region, subcutaneous fat [FAT], and also in the marrow of the clivus [C]) is extremely bright, whereas fluid (as seen in cerebrospinal fluid (CSF) in the prepontine cistern and Meckel cave (M) is dark. Soft tissue such as muscle and brain parenchyma are intermediate in signal intensity, whereas air (as in the sphenoid sinus [SS]) is black. The white arrowhead indicates the flow void of the basilar artery. White arrows indicate nasopharyngeal carcinoma infiltrating the fat of the right pterygopalatine fossa, which appears expanded and of lower signal intensity than the normal, mostly fatty pterygopalatine fossa on the left. B, A coronal fast spin-echo (FSE) T2-weighted image with fat saturation is shown. Note that the signal from subcutaneous fat (FAT) is completely suppressed. Fluid appears bright, as seen with CSF in a cerebral sulcus (arrowheads) and the vitreous humor within the ocular globe (G). Mucoid material (M) within the maxillary, frontal, and ethmoid sinuses is extremely bright. In contrast, a highly cellular inverting papilloma (white arrows) is of intermediate signal intensity and shows clearly in contrast to thickened mucosa and sinonasal secretions. C, A postgadolinium axial T1-weighted image with fat saturation is shown. Subcutaneous fat appears dark because its signal has been suppressed. Gadolinium administration can be confirmed by looking at the sinonasal mucosa, which enhances brightly postgadolinium. Orbital fat appears bright because of local failure of fat saturation resulting from inhomogeneity of the local magnetic field caused by the proximity to the air-filled maxillary sinuses. Abnormal enhancement is seen along foramen rotundum (white arrows) and in the Meckel caves (white arrowheads) because of lymphomatous infiltration of the trigeminal nerves. Increased enhancement of the temporalis muscles bilaterally (T) is related to denervation change from involvement of V3. D, A slightly more superior postgadolinium axial T1-weighted image with fat saturation demonstrates abnormal thickening and enhancement of the cisternal segments of the trigeminal nerves bilaterally, also caused by lymphomatous infiltration. E, An axial fast spin-echo T2-weighted image demonstrates a large mass involving the right maxillary sinus, right nasal cavity, and right ethmoid air cells. Fat suppression was not used in this case. F, An axial diffusion-weighted image (same patient as in Fig. 17-2E) shows marked hyperintensity of the lesion. This image contains both T2-weighted and diffusion-weighted information. G, The apparent diffusion coefficient map at the same level as Fig. 17-2F represents the diffusion-weighted image with the T2 contribution removed and it therefore represents “true” diffusion information, with reduced diffusion demonstrated as low signal intensity in the mass. This is consistent with high cellularity, as is often seen in small, round, blue-cell tumors. In this case, biopsy confirmed sinonasal rhabdomyosarcoma.
Additional planes may be useful in some circumstances (e.g., coronal FSE T2-weighted images with fat saturation for paranasal sinus pathologic conditions). Additional sequences such as magnetic resonance angiography may also be useful in certain circumstances (e.g., paragangliomas), but are not necessary for evaluation of most neoplasms of the head and neck. Modalities in widespread use in the brain (magnetic resonance spectroscopy, diffusion-weighted imaging, functional MRI) have not yet found a place in routine head and neck imaging. There is growing interest in diffusion-weighted imaging in the head and neck, however, because the relative reduction in water diffusion that is seen in highly cellular neoplasms can be useful in the differential diagnosis and staging of primary tumors and the detection of residual or recurrent disease following primary therapy (Fig. 17-2E to Fig. 17-2G).6
As mentioned earlier, in many cases MRI is preferred to CT because of its superior soft-tissue contrast and increased sensitivity to processes such as perineural spread of tumor. It is important for the clinician to be aware of MRI safety considerations, however, and relative and absolute contraindications to MRI.7,8 It is also important to be aware of the entity of nephrogenic systemic fibrosis (NSF), a scleroderma-like condition that primarily involves the skin but may also affect organs such as the liver, lung, heart, and muscle. Current thinking suggests an association and a potential causal link between the use of gadolinium-based contrast agents and the development of NSF among patients with advanced kidney disease; hence it is important to screen for kidney disease prior to administration of gadolinium-based contrast agents for MR scanning.9
Positron Emission Tomography
PET offers information regarding tissue metabolism rather than simply demonstrating anatomy. The most common radiopharmaceutical in use for cancer imaging at present is 18F-fluorodeoxyglucose (FDG). This is taken up into tissues in proportion to the glycolytic rate, which is generally increased in neoplastic processes. FDG does accumulate in some normal structures to a variable degree (sublingual glands, lymphoid tissue of Waldeyer ring) (Fig. 17-3),10 and may also accumulate in active muscles (e.g., the larynx if a patient has been talking) and brown fat (which is often present in the supraclavicular fossae). The current widespread availability of PET-CT scanning allows far more precise localization of FDG activity and more precise interpretation than was previously possible with FDG PET scanning alone, and allows pitfalls (such as interpreting vascular, muscular, or brown fat activity as neoplasia) to be avoided.11,12 Situations in which FDG PET-CT scanning is particularly helpful include the search for an unknown primary lesion in a patient presenting with metastatic neck disease (Fig. 17-4A), the assessment of residual or recurrent disease following primary therapy, and the search for synchronous or metachronous primary lesions or distant metastases (Fig. 17-4B and Fig. 17-4C).13,14 FDG PET-CT scanning is also useful for staging the neck. There may be a significant number of false-negative studies in patients with clinically N0 necks, however, as small tumor deposits (on the order of 1 to 4 mm) may not be detectable on FDG PET-CT scan, but will be found if a neck dissection is performed. It must also be remembered that FDG is a nonspecific tracer that is concentrated in areas of inflammation as well as neoplasia. An FDG PET-CT scan must therefore always be interpreted in appropriate clinical context and must be supplemented with tissue sampling and follow-up imaging as appropriate.
FIGURE 17-4 • A, Axial fused image from an 18F-fluorodeoxyglucose (FDG) positron emission tomography–computed tomography (PET-CT) scan in a patient who presented with a left neck mass concerning for metastatic cervical lymphadenopathy, and fine-needle aspiration positive for squamous cell carcinoma (SCC). Initial head and neck evaluation was unremarkable other than the left level-II node. The fused PET-CT image in the axial plane demonstrates expected activity in the left level-II node (slightly concave arrow), as well as subtly asymmetrical activity in the left base of tongue (deeply concave arrow). Mild normal activity is shown in the right base of tongue (concave arrowhead) for comparison. Endoscopy revealed a very subtle lesion in the left base of tongue, and a biopsy was positive for SCC. B, A whole-body projection image from an 18F-FDG PET-CT study performed to stage an oropharyngeal SCC in a different patient. The large primary tumor is seen (white arrow), as is a right level-II lymph node (upper black arrow). A large focus of activitiy in the mediastinum (lower black arrow) was unexpected. C, A coronal fused PET-CT image (same patient as in FIGURE 17-4B) localizes activity to the thoracic esophagus. This lesion proved to be an unexpected and asymptomatic SCC of the esophagus.
Imaging the Head and Neck
The anatomy of the head and neck is extremely complex. To facilitate communication and differential diagnosis, the supra- and infrahyoid neck are usually discussed as a series of spaces.15–17 We discuss primarily the pharyngeal mucosal space, from which squamous cell carcinomas (SCCs), lymphomas, and minor salivary gland tumors may arise. In this discussion, we refer to the traditional divisions of this space into the nasopharynx, oropharynx, oral cavity, and hypopharynx. The larynx, located in the infrahyoid visceral space, is also discussed. The deeper spaces of the head and neck may be involved by deep extension of primarily mucosal lesions or may be the site of origin of soft-tissue sarcomas and other nonsquamous neoplasms.
Spatial Anatomy of the Head and Neck
The spaces of the suprahyoid neck are defined by the three layers of the deep cervical fascia (the superficial, middle, and deep layers). The spaces so defined include the pharyngeal mucosal space, parapharyngeal space, masticator space, parotid space, carotid space, retropharyngeal space, and perivertebral space. These spaces are demonstrated in Fig. 17-5. In a discussion of head and neck cancer, however, which is dominated by SCC of the mucosa of the upper aerodigestive tract, we discuss lesions arising in the nasopharynx, oropharynx, oral cavity, hypopharynx, and larynx. The infrahyoid neck has traditionally been taught from the point of view of surgical triangles, but can also be described as a series of spaces, which facilitates understanding and interpretation of cross-sectional imaging modalities such as CT and MRI. The spaces of the infrahyoid neck are also defined by the three layers of the deep cervical fascia and include the superficial space (external to the superficial layer of the deep cervical fascia), visceral space (including the thyroid gland, larynx, and esophagus), carotid space, retropharyngeal space, and prevertebral space. These spaces are demonstrated in Fig. 17-6.
Nasopharynx
Normal Anatomy
The nasopharynx is bounded anteriorly by the posterior nasal cavity at the level of the choana; superiorly by the sphenoid sinus; posterosuperiorly by the clivus, upper cervical spine, and prevertebral muscles; and inferiorly by the soft palate. Its contents include squamous mucosa, lymphoid tissue (adenoids), pharyngeal constrictor muscles, the levator palatini muscle, and the torus tubarius (the projecting posterior lip of the pharyngeal opening of the eustachian tube). Because of the location of the pharyngeal opening of the eustachian tube, nasopharyngeal masses often obstruct the eustachian tube, causing dysfunction and serous otitis. The Rosenmüller fossa represents the uppermost aspect of the lateral recess of the nasopharynx and is a common site of origin of nasopharyngeal cancers. The normal nasopharynx is shown in Fig. 17-7.
Pathologic Conditions
Nasopharyngeal carcinoma (NPC) is the most common malignant lesion of the nasopharynx. NPC may be small and confined to the Rosenmüller fossa or large with extension to the skull base, intracranial structures, and deep spaces of the head and neck.18 Lymphadenopathy is often a prominent feature of even early NPC. NPCs most commonly metastasize to retropharyngeal, level-II, and level-V nodes. Contralateral nodes are also at significant risk because of rich bilateral lymphatic drainage, and nodal imaging should extend from the high retropharyngeal level to the supraclavicular fossa. Note that the imaging appearance of normal and abnormal lymph nodes and the classification of lymph nodes based on anatomic “levels” is discussed later in the “Lymph Nodes” section of this chapter.
NPC generally presents as an asymmetric, infiltrative mass with a variably exophytic component that is intermediate in signal intensity on both T1– and T2-weighted images and shows homogeneous enhancement postgadolinium (Fig. 17-8). Small lesions are typically limited by the surrounding pharyngobasilar fascia; once this barrier is breached, the tumor may directly invade the adjacent fatty parapharyngeal space. NPC may also extend directly to the skull base: superiorly or superolaterally to the floor of the sphenoid sinus, pterygoid process, or foramen lacerum, or posteriorly to the clivus. Skull-base involvement is best detected on T1-weighted images, which show loss of the normal bright signal of marrow fat (Fig. 17-9). The sinus of Morgagni, which allows passage of the cartilaginous portion of the eustachian tube and the levator veli palatini muscle from the skull base to the pharynx, is a natural defect that may allow extension of NPC laterally into the parapharyngeal space and carotid space. NPC may then involve V3 and extend intracranially via the foramen ovale to the Meckel cave. Cavernous sinus extension and perineural spread of disease (usually along V3) are often best demonstrated on postgadolinium T1-weighted images with fat saturation (Fig. 17-10).
Oropharynx
Normal Anatomy
The oropharynx is bounded anteriorly by the circumvallate papillae of the tongue and the anterior tonsillar pillars; these structures separate the oropharynx from the oral cavity.19 Posteriorly it is bounded by the pharyngeal constrictor muscles and superiorly by the soft palate. Inferiorly it is separated from the larynx by the epiglottis and glossoepiglottic fold and from the hypopharynx by the pharyngoepiglottic fold. Its contents include the tongue base, palatine tonsils, soft palate, and oropharyngeal mucosa and constrictor muscles from the level of the palate to the hyoid bone. The normal oropharynx is shown in Fig. 17-11.
Pathologic Conditions
SCC accounts for most tumors of the oropharynx, followed by lymphoma, minor salivary gland lesions, and mesenchymal tumors.20 The appearance of SCC varies somewhat with the specific subsite involved, and the tumor may be variably exophytic or deeply infiltrative. Tumors of the tonsillar fossa may be occult when small and may present with cervical nodal disease. Tonsil cancers most commonly metastasize to level-II lymph nodes. Larger tumors of the tonsillar fossa may spread anteriorly or posteriorly to involve the tonsillar pillars or anteriorly to invade the tongue. They may extend deeply to invade the constrictor muscles and then gain access to the parapharyngeal space and skull base.
Tumors of the base of the tongue, when small, may be difficult to separate from normal lingual tonsillar tissue. Malignancy of the tongue base is usually asymmetrical, crossing midline only when large. Patterns of spread include posterolaterally to the tonsillar pillar and pharyngeal wall, submucosally into the supraglottic larynx, or anteriorly into the floor of the mouth. Nodal metastases are common with base-of-tongue tumors, with level-II nodes most commonly involved, followed by level-III nodes. Contralateral level-II nodes are also commonly involved. The imaging features of lymphomas and minor salivary tumors are similar to SCC, and clinical examination and tissue sampling are necessary to make a specific diagnosis in most cases. Representative tonsillar fossa and base of tongue lesions are shown in Fig. 17-12 and Fig. 17-13.