Chapter 39 Hamstring Tendon Interference Screw Fixation
Biomechanical and Biological Considerations
The concept of interference screw fixation involves the parallel insertion of the screw to the graft in the tunnel, which allows compression of the graft to the bony tunnel wall and biological graft incorporation. Graft fixation using interference screws presents the “gold standard” when using a bone–patellar tendon–bone (BPTB) graft. This type of fixation combines high initial construct stiffness and early bony graft incorporation.1 These factors are generally required to allow for an accelerated rehabilitation, which has been demonstrated to improve clinical outcome.2 Soft tissue grafts, however, do not provide attached bone blocks for interference fit fixation. Additionally, tendon–bone healing generally takes longer for bony graft incorporation compared with bone–bone healing (4 to 6 weeks versus 6 to 12 weeks).3–7 Thus fixation of soft tissue grafts requires improved mechanical and biological boundary conditions for graft fixation compared with grafts with attached bone blocks.
Biomechanical Boundary Conditions
Ideally, a graft fixation construct should be similar in strength and stiffness to the native human anterior cruciate ligament (ACL). Current fixation techniques demonstrate a wide range of in vitro measured graft fixation loads (200N to 1200N). However, today evidence is still limited regarding real in vivo requirements for initial construct strength.8 Noyes et al calculated that the ACL is loaded up to 454N during activities of daily living,9 but other authors reported a high amount of good and excellent results with fixation types whose failure load is far below that anticipated value.10–12 For soft tissue graft fixation using biodegradable interference screws, failure loads of 250N to 800N have been demonstrated depending on screw length and insertion torque.13–15
Graft fixation generally is divided into anatomical (aperture or joint line fixation), nonanatomical, and semi-anatomical (Fig. 39-1), according to the location of fixation in relation to the joint line. Graft fixation directly at the joint line (site of the native ACL insertion) is called anatomical, whereas an extracortical fixation (e.g., staples, fixation buttons) is called nonanatomical. For example, transfixation devices or tibial interference screws that are not deeply inserted provide an intraosseous and thus a semi-anatomical mounting of the graft.
This classification is important because the site of graft fixation determines the length of the complete graft fixation construct. The length of the native ACL is between 2.8 and 3.7 cm. Reconstruction of the anteromedial bundle of the ACL results in an intraarticular graft length of only approximately 2.5 to 3.2 cm. Using femoral and tibial nonanatomical fixation devices, the length of the graft fixation construct can easily reach 10 to 15 cm (see Fig. 39-1). In correlation to the distance between the fixation devices, reversible elastic longitudinal deformations of the graft have been demonstrated. This phenomenon also is known as the “bungee-cord effect.” Furthermore, sagittal intratunnel graft motion (reversible) might occur due to anteroposterior translation of the graft during knee flexion and extension using a nonanatomical or semianatomical graft fixation technique (“windshield-wiper effect”).15,16 Thus a long distance between the femoral and tibial fixation device results in low construct stiffness and graft–tunnel motion.
Additionally, indirect and direct graft fixation should be distinguished (see Fig. 39-1). The concept of indirect graft fixation implicates the use of linkage material between graft and fixation device (e.g., suture loop of Endobutton fixation), whereas direct fixation means anchoring of the graft without any additional material except the fixation device itself (e.g., interference screws, staples, transfixation devices). Longitudinal irreversible graft deformation might occur using indirect fixation techniques due to stretch-out of linkage material (e.g., suture loop of Endobutton fixation) or suture attachment at the graft tissue.13,17,18
Longitudinal and sagittal graft–tunnel motion inhibits constant graft–tunnel contact at the tunnel entry site and thus compromises bony graft incorporation at the site of the native ACL insertion.5,19 Furthermore, graft–tunnel motion might lead to graft laceration at the tunnel entry site during dynamic loading20 and is a factor responsible for the development of tunnel enlargement.16 Although current literature is not consistent concerning the correlation between tunnel enlargement and postoperative knee stability16,21,22 in revision ACL reconstruction, tunnel enlargement is of clinical importance and should therefore be prevented.
According to these considerations, interference screw fixation offers high construct stiffness and prevents intratunnel graft motion because it allows for direct and anatomical graft fixation at the level of the joint line combined with adequate initial fixation strength.5,23 Recent clinical data support the belief that clinical outcome can be improved with anatomical joint line fixation using interference screws for hamstring tendon grafts.24
Biological Boundary Conditions
In addition to correct tunnel placement, graft incorporation represents the main factor for long-term survival of an ACL reconstruction and is mainly influenced by the type of fixation. The surgical target is to create biological (and biomechanical) boundary conditions that allow for the restitution of a native ACL insertion site anatomy.5 The normal ACL insertion site consists of four zones (so-called direct ligament insertion). The first zone comprises the ligament, the second is characterized by fibrocartilage, the third zone consists of a mineralized cartilage tidemark, and the fourth is where the mineralized cartilage tidemark inserts into the subchondral bone plate.5 The design of this complex insertion anatomy allows distribution of longitudinal and shear forces from the ligament into the subchondral bone plate. The development of a direct ligament insertion at the level of the joint line has been demonstrated histologically in an animal model using anatomical soft tissue graft fixation with compression at the tunnel entry site (interference screws).25 In contrast, the use of a nonanatomical fixation technique in the same model showed the development of an indirect type of ligament insertion or only a delayed formation of a direct type of insertion.25 In indirect insertions, the surface of the ligament connects with the periosteum whereas the deeper layers connect to bone via Sharpey fibers (e.g., medial collateral ligament). This is of inferior mechanical competence compared with the direct ligament insertion.5
Thus it is reasonable to assume that neutralization of graft-tunnel motions using anatomical and direct interference screw fixation obviously improves osseous graft incorporation by means of the development of a native ACL insertion anatomy.25
Interference Screws
Metallic interference screws initially were developed for fixation of grafts with attached bone blocks. These screws are threaded sharply to achieve good starting conditions for screw insertion and secure graft fixation. The use of this type of interference screw for soft tissue graft fixation might lead to laceration of the graft tissue during screw insertion, especially if high insertion torque is generated. Thus different round-threaded interference screws have been developed for soft tissue graft fixation.26,27 The first round-threaded metallic interference screw for direct fixation of soft tissue grafts was developed by L. Pinczewski, the round-headed cannulated interference screw (RCI)10,26 (Fig. 39-2). More recently, biodegradable interference screws have been developed and biomechanically as well as clinically tested for fixation of BPTB and soft tissue grafts.27,28 Soft tissue graft fixation using biodegradable interference screws was first described by Stähelin and Weiler.29
Biodegradable interference screws have been demonstrated to be advantageous compared with metallic screws by means of undistorted radiological imaging, uncompromised revision surgery, and minimized risk of graft laceration. However, one might consider that most currently available biodegradable interference screws do not show complete degradation and subsequent osseous replacement of the former implant site because they consist of slow degrading and high-molecular poly-L-lactide.27 Thus the use of intermediate degrading stereo-co-polymeric materials such as poly-(L-co-D,L-lactide) are preferable.27,30
The newest screw generations are sharply threaded just at the tip for easy starting conditions of the screw, followed by a blunt threading to prevent tissue laceration (see Fig. 39-2). Biodegradable as well as metallic interference screws are distributed in different sizes (diameter and length). Thus precise matching of graft and tunnel diameters can easily be performed.
Mechanical studies have shown that a tight fit among the screw, the graft, and the tunnel is essential for sufficient fixation strength.13,31–33 When deciding to oversize a screw to improve fixation strength (particularly at the tibial site), an increased length has been demonstrated to be superior to an increased diameter.13,34 Furthermore, an oversized screw diameter increases the insertion torque in contrast to a longer screw. We therefore recommend increasing screw length instead of using massively oversized screws in diameter (e.g., Delta screws) to avoid violating the tendon tissue.
In summary, hamstring tendon interference screw fixation offers the following advantages:
Technical Considerations
Graft Preparation
Because preservation of the gracilis tendon has been demonstrated to be beneficial35,36 and due to the fact that anatomical joint line fixation requires only a short graft (at least 7 cm) (Fig. 39-3), the use of a four-stranded semitendinosus tendon graft should be routinely achieved. To gain a sufficient length of the semitendinosus tendon, the tendon can be harvested including the periosteal distal insertion of the tendon. In most cases this results in a tendon length of at least 28 cm. If the semitendinosus tendon is very short (less than 26 cm) or thin, one can additionally harvest the gracilis tendon to create a four- or five-stranded semitendinosus/gracilis tendon graft.
The four-stranded graft is prepared with the help of a suture board while arthroscopic preparation of the knee is performed. The proximal and distal endings of the semitendinosus tendon are armed with #2 polyester sutures in a whipstitch fashion (Fig. 39-4). Care has to be taken to pull all slack out of each suture pass. Then the construct should be manually tensioned to allow potential slippage to be taken out of the construct (Fig. 39-5). The tendon is then looped over itself using the so-called “W-technique,” and a polyester passing suture is brought through each loop (Fig. 39-6). The looped tendons are then pulled through a graft sizer (Fig. 39-7). The resulting diameter of the graft is usually between 7 and 9 mm. A tight fit of the graft in the tunnel generally is required to improve fixation strength and graft incorporation. The diameter of the graft is a given value, which needs to be known before tunnel creation. When hybrid fixation (see later discussion) is used, sizing of the graft in increments of 1 mm is sufficient; if interference screw fixation is used solely, we recommend sizing in increments of 0.5 mm33 to allow for the required tight fit. A marking suture using #0 absorbable suture has to be set 2 cm from the femoral end of the graft to show the surgeon if the graft is inserted deep enough into the femoral tunnel. The side effect of the suture is that good passage of the graft in the tunnel is ensured, and twisting of the graft around the screw during its insertion is prevented (see later discussion; see also Fig. 39-3).
If femoral hybrid fixation using the EndoPearl (Linvatec, Largo, FL) device is desired, the pearl is tied to the femoral end of the graft (see Fig. 39-3). It is important that the knot fixing the pearl to the graft is not placed at the side of the screw, which would result in an increased graft diameter, possibly leading to problems during graft insertion. The tibial end of the graft is sutured in a baseball-stitch technique using #0 absorbable sutures to ease the insertion of the tibial screw and to increase initial tibial graft fixation strength (see Fig. 39-3).
Femoral Interference Screw Fixation
Tunnel Preparation
According to the current literature the femoral tunnel should be drilled in the ten-o’clock position for right knees or in the two–o’clock position for left knees.37–39 Arnold et al demonstrated that this position cannot or can hardly be achieved when using the conventional transtibial techniques (single incision).40 Thus we routinely use the anteromedial portal technique in all ACL reconstruction procedures because it allows for an anatomical lateral tunnel placement.
In the anteromedial tunnel technique, we create the femoral tunnel first in approximately 120 to 130 degrees of flexion (Fig. 39-8). Thus tunnel direction is directed more to the center of the bone and away from the posterior femoral cortex, which prevents posterior breakage of the tunnel wall. As this amount of knee flexion only hardly can be achieved when using a standard leg holder, we routinely use a lateral support at the level of the thigh and put the leg on the operating table in maximum knee flexion (Fig. 39-9).
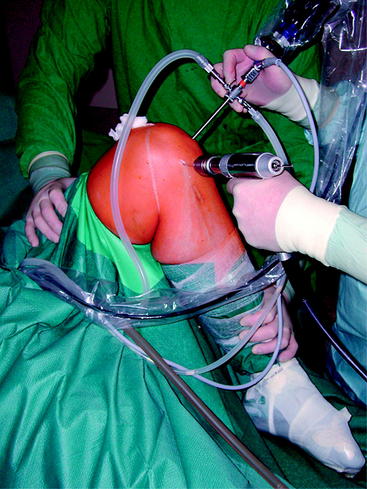
Fig. 39-9 Knee held in maximum flexion during creation of the femoral tunnel via the anteromedial portal.
The standard sizing when using interference screw fixation at the femoral site alone is:
The length of the screw should be 23 to 25 mm to achieve sufficient initial fixation strength.
Screw Insertion
Interference Screw Position
Problems in femoral hamstring tendon interference screw fixation might occur if the graft tends to rotate around the screw during its insertion; this might lead to an undesired position of the graft (Fig. 39-12). When using a standard right-threaded screw in right knees, the graft might rotate toward an anterolateral position, resulting in too-anterior graft placement and lateral wall impingement. Thus Pinczewski et al could show a difference of clinical outcome between right and left knees after hamstring tendon interference fit fixation.41 Subsequently, a reversed-threaded interference screw was developed for use in right knees.41 However, in a left knee with a conventional screw or in a right knee with a reversed screw, the graft might rotate toward the 12-o’clock position, which might lead to compromised control of knee rotation.40,42 We therefore recommend preventing screw rotation completely.
Possible Problems During Screw Insertion
Femoral Hybrid Fixation
An important method that further minimizes graft–tunnel motions and improves initial fixation strength as well as construct stiffness is the so-called hybrid fixation.31,43 The principle of hybrid fixation is to combine two fixation techniques at one site.31 At least one of the devices used should be able to achieve sufficient fixation strength alone. This device is combined with a second technique in order to neutralize its possible biomechanical and biological disadvantages (e.g., graft–tunnel motion) (Table 39-1).
Type of Fixation | Possible Methods |
---|---|
Femoral hybrid fixation | Interference screw and EndoPearl Suture button and interference screw Suture button and cancellous bone plug Transfixation and interference screw Transfixation and cancellous bone plug |
Tibial hybrid fixation | Interference screw and suture to bony bridge Interference screw and suture button Interference screw and staples Cancellous bone plug and suture button Cancellous bone plug and suture to bony bridge Cancellous bone plug and tying of sutures over screw |
On the femoral site, graft fixation generally is more forgiving compared with the tibia from a mechanical point of view. However, disturbed biological incorporation is a problem of femoral fixation due to the higher graft–tunnel motions.25 We therefore recommend aperture fixation on the femur to optimize biological boundary conditions. In order to minimize possible problems of interference screw fixation, such as tissue laceration and graft rotation, femoral hybrid fixation allows for the use of an undersized screw to solve these problems.
For femoral hybrid fixation with interference screws, a biodegradable spherical device, the EndoPearl, has been developed (see Fig. 39-3). The EndoPearl is tied to the femoral end of the graft and achieves an internal locking between the graft and the tip of the interference screw (Fig. 39-15). Thus it increases initial fixation strength, especially in cases of tunnel enlargement (revision reconstruction) or low bone density. Clinically it has been shown to improve knee stability compared with interference screw fixation alone.44 If this type of femoral hybrid fixation is used, the femoral tunnel should be created 1 cm deeper than normally recommended (35 mm instead of 25 mm). Additionally the chosen diameter of the screw can be 1 to 2 mm less than the tunnel diameter without compromising fixation strength. This is advantageous because it decreases the risk of tissue laceration (insertion torque) and reduces screw rotation without compromising initial fixation strength.
Thus the standard sizing when using femoral hybrid fixation with the EndoPearl is:
As an alternative, tunnel diameter can be increased and screw size chosen according to the graft diameter when hybrid fixation is used. This allows for hamstring tendon interference screw fixation in revision cases as well. As another alternative, a cortical bone plug can be tied to the femoral end of the graft.45
Tibial Interference Screw Fixation
Tunnel Preparation
On the tibial site, screw position is routinely chosen to be posterior to the graft (see Fig. 39-15). This position prevents the screw from anterior graft impingement if it is accidentally inserted too deep. The tibial screw can be positioned anterior to the graft if the tibial tunnel is placed slightly too far anterior to push the graft posteriorly and to prevent a notch impingement.
Matching of Tibial Tunnel and Screw Diameter
Therefore, when using tibial hybrid fixation with interference screws, the standard procedure is:
If one prefers using interference screw fixation alone, the following sizing is recommended:
Possible Problems during Screw Insertion
A specific problem at the tibial site is the “blind” insertion of the screw. Thus the risk of inserting the screw not parallel to the graft is increased compared with the femoral site. Furthermore, it might easily happen that the screw is not inserted between the graft and the tunnel wall but instead pushes the graft forward during its insertion. To prevent this accidental graft dislocation, the surgeon should hold the holding sutures of the graft tightly during screw insertion. After tibial screw insertion we recommend controlling graft and screw placement by an intratunnel view using the arthroscope (Fig. 39-16).
Tibial Hybrid Fixation
When interference screw fixation is used for soft tissue grafts, some authors are concerned about a possible fixation slippage at the tibial fixation site even though fixation strength at the femoral site is considered sufficient.13,18,32,46 The risk of graft slippage on the tibial site can be nicely compensated with oversized screws, or better, by using hybrid fixation on the tibia, especially in females.34,47
An easy and safe method of tibial hybrid fixation is to tie the holding sutures of the graft over a bony bridge after the tibial screw is inserted (Fig. 39-17). For this a monocortical drill hole is created 2 cm distally of the tibial tunnel exit site. Then one strand of each suture is passed through the hole and tied over the created bony bridge.48
In cases with a low insertion torque of the interference screw, the use of a suture button instead of the suture over bony bridge might be beneficial (Fig. 39-18). Manual rotation of the button tightens the linkage material, thus safely preventing graft slippage.
1 Kurosaka M, Yoshiya S, Andrish J. A biomechanical comparison of different surgical techniques of graft fixation in anterior cruciate ligament reconstruction. Am J Sports Med. 1987;15:225.
2 Shelbourne KD, Nitz P. Accelerated rehabilitation after anterior cruciate ligament reconstruction. Am J Sports Med. 1990;18:292-299.
3 Grana WA, Egle DM, Mahnken R, et al. An analysis of autograft fixation after anterior cruciate ligament reconstruction in a rabbit model. Am J Sports Med. 1994;22:344-351.
4 Rodeo SA, Arnoczky SP, Torzilli PA, et al. Tendon-healing in a bone tunnel. A biomechanical and histological study in the dog. J Bone Joint Surg. 1993;75A:1795-1803.
5 Weiler A, Scheffler S, Apraleva M. Healing of ligament and tendon to bone. In: Walsh W, editor. Repair and regeneration of ligaments, tendons and capsule. Totawa, New Jersey: The Humana Press; 2005:201-231.
6 Weiler A, Hoffmann RF, Bail HJ, et al. Tendon healing in a bone tunnel. Part II: histologic analysis after biodegradable interference fit fixation in a model of anterior cruciate ligament reconstruction in sheep. Arthroscopy. 2002;18:124-135.
7 Weiler A, Peine R, Pashmineh-Azar A, et al. Tendon healing in a bone tunnel. Part I: biomechanical results after biodegradable interference fit fixation in a model of anterior cruciate ligament reconstruction in sheep. Arthroscopy. 2002;18:113-123.
8 Rupp S, Hopf T, Hess T, et al. Resulting tensile forces in the human bone-patellar tendon-bone graft: direct force measurements in vitro. Arthroscopy. 1999;15:179-184.
9 Noyes FR, Butler DL, Grood ES, et al. Biomechanical analysis of human ligament grafts used in knee-ligament repairs and reconstructions. J Bone Joint Surg. 1984;66A:344-352.
10 Pinczewski LA, Deehan DJ, Salmon LJ, et al. A five-year comparison of patellar tendon versus four-strand hamstring tendon autograft for arthroscopic reconstruction of the anterior cruciate ligament. Am J Sports Med. 2002;30:523-536.
11 Roe J, Pinczewski LA, Russell VJ, et al. A 7-year follow-up of patellar tendon and hamstring tendon grafts for arthroscopic anterior cruciate ligament reconstruction: differences and similarities. Am J Sports Med. 2005;33:1337-1345.
12 Shelbourne KD, Patel DV. ACL reconstruction using the autogenous bone-patellar tendon-bone graft: open two-incision technique. Instr Course Lect. 1996;45:245-252.
13 Scheffler SU, Sudkamp NP, Gockenjan A, et al. Biomechanical comparison of hamstring and patellar tendon graft anterior cruciate ligament reconstruction techniques: the impact of fixation level and fixation method under cyclic loading. Arthroscopy. 2002;18:304-315.
14 Selby JB, Johnson DL, Hester P, et al. Effect of screw length on bioabsorbable interference screw fixation in a tibial bone tunnel. Am J Sports Med. 2001;29:614-619.
15 Weiler A, Scheffler SU, Sudkamp NP. [Current aspects of anchoring hamstring tendon transplants in cruciate ligament surgery.]. Chirurg. 2000;71:1034-1044.
16 Höher J, Möller H, Fu F. Bone tunnel enlargement after anterior cruciate ligament reconstruction: fact or fiction. Knee Surg Sports Traumatol Arthrosc. 1998;6:231-240.
17 Höher J, Scheffler SU, Withrow JD, et al. Mechanical behavior of two hamstring graft constructs for reconstruction of the anterior cruciate ligament. J Orthop Res. 2000;18:456-461.
18 Magen H, Howell S, Hull M. Structural properties of six tibial fixation methods for anterior cruciate ligament soft tissue grafts. Am J Sports Med. 1999;27:35-43.
19 Natsu-Ume T, Shino K, Nakata K, et al. Endoscopic reconstruction of the anterior cruciate ligament with quadrupled hamstring tendons: a correlation between MRI changes and restored stability of the knee. J Bone Joint Surg. 2001;83B:837-840.
20 Toritsuka Y, Shino K, Horibe S, et al. Second-look arthroscopy of anterior cruciate ligament grafts with multistranded hamstring tendons. Arthroscopy. 2004;20:287-293.
21 Buelow JU, Siebold R, Ellermann A. A prospective evaluation of tunnel enlargement in anterior cruciate ligament reconstruction with hamstrings: extracortical versus anatomical fixation. Knee Surg Sports Traumatol Arthrosc. 2002;10:80-85.
22 Stange R, Russel V, Salmon L, et al. Tibial tunnel widening after ACL reconstruction: a 2 and 5 year comparison of patellar tendon autograft and 4-strand hamstring tendon autograft. Arthroscopy Assoc N Am. 2001. 20th Annual Meeting:67
23 Weiler A, Hoffmann RF, Stahelin AC, et al. Hamstring tendon fixation using interference screws: a biomechanical study in calf tibial bone. Arthroscopy. 1998;14:29-37.
24 Wagner M, Kaab MJ, Schallock J, et al. Hamstring tendon versus patellar tendon anterior cruciate ligament reconstruction using biodegradable interference fit fixation: a prospective matched-group analysis. Am J Sports Med. 2005;33:1327-1336.
25 Weiler A, Unterhauser F, Faensen B, et al. Comparison of tendon-to-bone healing using extracortical and anatomic interference fit fixation of soft tissue grafts in a sheep model of ACL reconstruction. ns Orthop Res Soc. 2002;48:173.
26 Scranton P, Pinczewski L, Auld M, et al. Outpatient endoscopic quadruple hamstring anterior cruciate ligament reconstruction. Operative Tech Orthop. 1996;6:177-180.
27 Weiler A, Hoffmann R, Stähelin A, et al. Current concepts: biodegradable implants in sports medicine—the biological base. Arthroscopy. 2000;16:305-321.
28 Weiler A, Windhagen HJ, Raschke MJ, et al. Biodegradable interference screw fixation exhibits pull-out force and stiffness similar to titanium screws. Am J Sports Med. 1998;26:119-126.
29 Stähelin A, Weiler A. All-inside anterior cruciate ligament reconstruction using semitendinosus tendon and soft threaded biodegradable interference screw fixation. Arthroscopy. 1997;13:773-779.
30 Hunt P, Unterhauser FN, Strobel MJ, et al. Development of a perforated biodegradable interference screw. Arthroscopy. 2005;21:258-265.
31 Brand J, Weiler A, Caborn D, et al. Graft fixation in cruciate ligament surgery: current concepts. Am J Sports Med. 2000;28:761-774.
32 Brand JCJr, Pienkowski D, Steenlage E, et al. Interference screw fixation strength of a quadrupled hamstring tendon graft is directly related to bone mineral density and insertion torque. Am J Sports Med. 2000;28:705-710.
33 Steenlage E, Brand JCJr, Johnson DL, et al. Correlation of bone tunnel diameter with quadrupled hamstring graft fixation strength using a biodegradable interference screw. Arthroscopy. 2002;18:901-907.
34 Weiler A, Hoffmann R, Siepe C, et al. The influence of screw geometry on hamstring tendon interference fit fixation. Am J Sports Med. 2000;;28:356-359.
35 Gobbi A, Domzalski M, Pascual J, et al. Hamstring anterior cruciate ligament reconstruction: is it necessary to sacrifice the gracilis? Arthroscopy. 2005;21:275-280.
36 Tashiro T, Kurosawa H, Kawakami A, et al. Influence of medial hamstring tendon harvest on knee flexor strength after anterior cruciate ligament reconstruction. A detailed evaluation with comparison of single- and double-tendon harvest. Am J Sports Med. 2003;31:522-529.
37 Hefzy M, Grood E, Noyes F. Factors affecting the region of most isometric femoral attachments. Am J Sports Med. 1989;17:208-216.
38 Loh JC, Fukuda Y, Tsuda E, et al. Knee stability and graft function following anterior cruciate ligament reconstruction: comparison between 11 o’clock and 10 o’clock femoral tunnel placement. Arthroscopy. 2003;19:297-304.
39 Sapega AA, Moyer RA, Schneck C, et al. Testing for isometry during reconstruction of the anterior cruciate ligament. Anatomical and biomechanical considerations. J Bone Joint Surg. 1990;72A:259-267.
40 Arnold MP, Kooloos J, van Kampen A. Single-incision technique misses the anatomical femoral anterior cruciate ligament insertion: a cadaver study. Knee Surg Sports Traumatol Arthrosc. 2001;9:194-199.
41 Musgrove TP, Salmon LJ, Burt CF, et al. The influence of reverse-thread screw femoral fixation on laxity measurements after anterior cruciate ligament reconstruction with hamstring tendon. Am J Sports Med. 2000;28:695-699.
42 Musahl V, Plakseychuk A, Vanscyoc A, et al. Varying femoral tunnels between the anatomical footprint and isometric positions. Am J Sports Med. 2005;33:712-718.
43 Tsuda E, Fukuda Y, Loh JC, et al. The effect of soft tissue graft fixation in anterior cruciate ligament reconstruction on graft-tunnel motion under anterior tibial loading. Arthroscopy. 2002;18:960-967.
44 Arneja S, Froese W, MacDonald P. Augmentation of femoral fixation in hamstring anterior cruciate ligament reconstruction with a bioabsorbable bead: a prospective single-blind randomized clinical trial. Am J Sports Med. 2004;32:159-163.
45 Nagarkatti DG, McKeon BP, Donahue BS, et al. Mechanical evaluation of a soft tissue interference screw in free tendon anterior cruciate ligament graft fixation. Am J Sports Med. 2001;29:67-71.
46 Kousa P, Jarvinen TL, Vihavainen M, et al. The fixation strength of six hamstring tendon graft fixation devices in anterior cruciate ligament reconstruction. Part II: tibial site. Am J Sports Med. 2003;31:182-188.
47 Hill PF, Russell VJ, Salmon LJ, et al. The influence of supplementary tibial fixation on laxity measurements after anterior cruciate ligament reconstruction with hamstring tendons in female patients. Am J Sports Med. 2005;33:94-101.
48 Weiler A, Richter M, Schmidmaier G, et al. The EndoPearl device increases fixation strength and eliminates construct slippage of hamstring tendon grafts with interference screw fixation. Arthroscopy. 2001;17:353-359.