Chapter 62 Genetic Diseases
The Long QT Syndrome
Congenital long QT syndrome (LQTS) is a relatively uncommon but important clinical disorder. Since 1975, under the unifying name of the “long QT syndrome,” it has been considered to include two hereditary variants.1 Jervell and Lange-Nielsen syndrome (J-LN) is associated with deafness, and Romano-Ward syndrome (R-W) is not.2–5
Molecular Genetics of Long QT Syndrome
The list of LQTS genes continues to grow. At the latest count, 12 of them had been identified, and the number is certain to increase. For practical purposes, however, the first three genes identified continue to remain the most important. This chapter will review only essential concepts; for additional details, interested readers are referred to more extensive reviews.6
KCNQ1 (LQT1) and KCNE1 (LQT5)
Expression studies of mutated proteins have suggested multiple mechanisms of functional failure. Defective proteins may coassemble with wild-type proteins and exert a dominant negative effect. Other mutations lead to defective proteins that do not assemble with wild-type peptides, which results in a loss of function that reduces the IKs current by 50% or less (haplo-insufficiency). Finally, defective peptides may not even reach the membrane of the cardiac cell because the mutations interfere with intracellular protein trafficking.7
KCNH2 (LQT2) and KCNE2 (LQT6)
The KCNH2 and the KCNE2 gene encode the α (HERG)-subunit and the β (MIRP)-subunit, respectively, of the potassium channel conducting the IKr current. This is the second most common variant of LQTS accounting for 35% to 40% of mutations in patients with the LQTS genotype. Mutations in KCNH2 cause a reduction of IKr current. Defective proteins may cause a dominant negative effect on the wild-type subunits, or they may not interfere with the function of the normal subunits, thus causing haplo-insufficiency. Trafficking abnormalities are another consequence of KCNH2 mutations.7
SCN5A (LQT3)
The SCN5A gene encodes the protein of the cardiac sodium channel. In vitro expression studies have shown that LQTS-SCN5A mutations produce the LQTS phenotype by inducing a “gain of function” leading to increase in the Na+ inward current, which prolongs the action potential duration. The prevalence of LQT3 among LQTS patients is around 10%.8
CACNA1c (LQT8): Timothy Syndrome
LQT8 is a rare variant characterized by marked Q-T interval prolongation, often presenting with 2 : 1 functional atrioventricular (AV) block, macroscopic T-wave alternans, and syndactyly. LQT8 is highly malignant and 10 (59%) of 17 of the children reported by Splawski et al died at a mean age of 2.5 years. Additional abnormalities may be present in Timothy syndrome.9
This variant has been associated, so far, with one specific missense mutation (G406R) in the voltage-gated calcium channel gene (CACNA1c).9 G406R produces sustained inward Ca2+ currents by causing nearly complete loss of voltage-dependent inactivation.9 In the heart, prolonged Ca2+ current delays cardiomyocyte repolarization and increases the risk of arrhythmia.
Prevalence
In 1975, it was already suggested that LQTS “could be more unrecognized than rare.”1 However, until now the prevalence was assumed to be anywhere between 1 in 5000 and 1 in 20,000. None of these estimates was based on actual data.
The first data-based assessment of the prevalence of LQTS comes from the largest prospective study of neonatal electrocardiography ever performed, which involved 44,596 infants of 3 to 4 weeks of age in 18 Italian maternity hospitals.10 An electrocardiogram (ECG) was performed in 44,596 infants 15 to 25 days old (43,080 whites). In infants with a QTc greater than 450 ms, the ECG was repeated within 1 to 2 weeks. Genetic analysis, by screening seven LQTS genes, was performed in 28 (90%) of 31 and in 14 (50%) of 28 infants with a QTc greater than 470 ms or between 461 and 470 ms, respectively, regarded as markedly prolonged by the European Task Force on Neonatal Electrocardiography.11 QTc readings of 451 to 460 ms, 461 to 470 ms, and greater than 470 ms were observed in 184 (0.41%), 28 (0.06%), and 31 (0.07%) infants, respectively (Figure 62-1). Among genotyped infants, disease-causing mutations were found in 12 (43%) of 28 with a QTc greater than 470 ms and in 4 (29%) of 14 with a QTc of 461 to 470 ms. One genotype-negative infant (QTc of 482 ms) was diagnosed to be affected by LQTS on clinical grounds. Among family members of genotype-positive infants, 51% were found to carry disease-causing mutations. In total, 17 of 43,080 white infants were affected by LQTS, which demonstrated a prevalence of at least 1 per 2534 apparently healthy live births (95% confidence interval [CI] 1:1 to 583 to 1:4 to 350). Among them, 1.4% had a QTc between 440 ms and 469 ms and 0.7 of 1000 had a QTc of 470 ms or greater. As 43% of the infants with QTc 470 ms or greater (0.7 per 1000) and 29% of the infants with a QTc between 460 ms and 469 ms have disease-causing mutations and as at least some of the infants with a QTc between 450 ms and 459 ms are also likely to be mutation carriers, it follows that the prevalence of LQTS must be close to 1 per 2000. This does not include the silent mutation carriers (QTc < 440 ms), a group ranging between 10% and 36% according to genotype.6 This is the first time that the prevalence of a cardiac disease of genetic origin has been quantified on the basis of actual data.
Clinical Presentation
The large increase in the number of patients diagnosed as affected has significantly modified the perception of the natural clinical history of the disease. Since the early 1970s, when the first consistent series of patients were reported, the typical clinical presentation of LQTS was considered to be the occurrence of syncope or CA, precipitated by emotional or physical stress, in a young individual with a prolonged Q-T interval on the surface ECG.1 If these symptomatic patients were left untreated, the syncopal episodes would recur and eventually prove fatal in the majority of cases. This concept was largely based on the fact that the patients initially diagnosed were those most severely affected. It has become progressively evident that this traditional picture represents an oversimplification, and it now appears that even though some patients have the severe manifestations described above, numerous patients have a very benign course as well. Unfortunately, the occasional occurrence of sudden death cannot yet be predicted. Evidence that modifier genes, some of them associated with the release of autonomic mediators, contribute to the highly different severity of the clinical manifestations of LQTS is growing.12–15
When family screening is performed, prolongation of the Q-T interval can be often detected, and a family history of fainting episodes or of sudden unexpected deaths in an early age is often present. Nonetheless, numerous sporadic cases (approximately 30%), that is, patients with syncope and a prolonged Q-T interval but without clinical evidence of familial involvement, do exist.6
The clinical history of repeated episodes of loss of consciousness under emotional or physical stress is typical and unique and is therefore unmistakable; however, the physician must be aware of the existence of LQTS to make the correct diagnosis. However, the clinical presentation is not always clear, so sometimes the diagnosis may be uncertain. The highly malignant J-LN syndrome will not be discussed here2; it must, however, be noted that its clinical course is very severe and that the patients become symptomatic at a very early age (Figure 62-2). Interested readers are referred to a comprehensive report on this variant.3
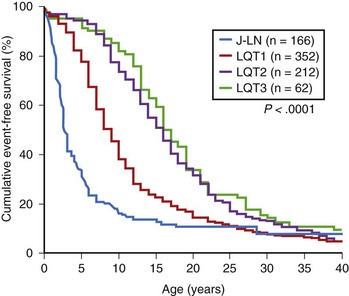
FIGURE 62-2 Kaplan-Meier curves of event-free survival. A, all patients with Jervell and Lange-Nielsen (J-LN) syndrome. B, All patients with J-LN, with a magnified view of the events occurring in the first 15 years of life. C, Patients with J-LN versus symptomatic patients with Romano-Ward syndrome of known genotype.10 D, Patients with J-LN versus symptomatic patients with LQT1 and an unselected LQT1 population.10,12
(From Schwartz PJ, Spazzolini C, Crotti L, et al: The Jervell and Lange-Nielsen syndrome. Natural history, molecular basis, and clinical outcome, Circulation 113:783–790, 2006.)
The two cardinal manifestations of LQTS are syncopal episodes and electrocardiographic abnormalities. The latter have been repeatedly described. Interested readers are referred to previous reviews.5 Here we will mention only T wave alternans and a specific echocardiographic pattern.
T-Wave Alternans
In 1975, Schwartz proposed that T-wave alternans represents a characteristic ECG feature of LQTS.16 Beat-to-beat alternation of the T wave, in polarity or amplitude, may be present at rest for brief moments but usually appears during emotional or physical stress and may precede torsades de pointes (TdP) (Figure 62-3). T-wave alternans is a marker of major electrical instability, and it identifies patients at particularly high risk. Its transient nature limits the possibility of its observation. This is a rather gross phenomenon that should not go unnoticed, when present. The observation of T-wave alternans in a patient with LQTS who is already on therapy strongly suggests the presence of a persisting high degree of cardiac electrical instability, which should prompt reassessment of therapy.
Echocardiographic Abnormalities
LQTS is still regarded by many as a purely electrical disease that does not involve any mechanical alterations. However, a case-control study demonstrated the frequent presence of highly unusual echocardiographic abnormalities among the patients.17 These abnormalities included an increased rate of thickening in the early phase of contraction and the presence of a slow movement in the late thickening phase with a plateau morphology sometimes accompanied by a second peak. Recently, these findings have been fully confirmed by a Norwegian study.18,19 LQTS is, therefore, not a purely electrical disease. These abnormalities were more frequent in symptomatic patients than in asymptomatic patients, which suggests that these abnormalities may reflect the presence of an arrhythmogenic mechanism. The evidence that the calcium-entry blocker verapamil completely normalizes the contraction pattern suggests that symptomatic LQTS patients may have an abnormal increase in the intracellular calcium concentration before relaxation is completed and that this may be related to an early after-depolarization (EAD): The contraction abnormality would be the mechanical equivalent of an EAD.20 The inward flux of calcium linked to the EAD may cause a small but rapid increase in intracellular calcium, which may be sufficient to trigger the calcium-induced release of calcium from the sarcoplasmic reticulum, causing a much greater increase in cytosolic calcium and the occurrence of a second contraction or the prolongation of the contraction itself.
Cardiac Events and Their Relationship to Genotype
The syncopal episodes are caused by TdP often degenerating into ventricular fibrillation. Long pauses facilitate the onset of TdP.21 Although most LQTS patients develop their symptoms under stress, sometimes these life-threatening cardiac events occur at rest. The reason(s) for these different patterns remained obscure until molecular biology was able to distinguish among different genotypes. In 1995, an unexpected observation was made that among a tiny group of genotyped individuals, LQT2 patients appeared to be at higher risk during emotional stress, whereas LQT3 patients had their events mostly at rest or during sleep; this fostered a large and targeted study that shed light on this issue of major clinical relevance.22,23
In 670 LQTS patients of known genotype and who all had symptoms (syncope, CA, or sudden death), Schwartz et al examined possible relationships between genotype and the conditions (“triggers”) associated with the events.23 As predicted by their impairment on the IKs current (essential for QT shortening during increases in heart rate), most of the events in LQT1 patients occurred during exercise or stress. Swimming is especially dangerous for them, and 99% of the events which occurred while swimming did involve LQT1 patients. Conversely, most of the events (including the lethal ones) in LQT2 patients occurred during emotional stress such as auditory stimuli (e.g., sudden noises and telephone ringing, especially occurring while at rest), and most of the events of LQT3 patients occurred while they were asleep or at rest (Figure 62-4).
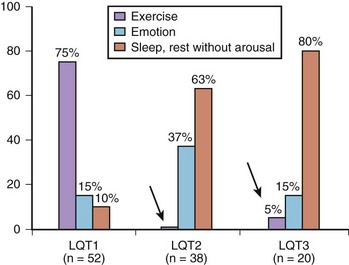
FIGURE 62-4 Lethal cardiac events according to triggers and genotype. Numbers in parentheses are triggers, not patients.
(Modified from Schwartz PJ, Priori SG, Spazzolini C, et al: Genotype-phenotype correlation in the long QT syndrome. Gene-specific triggers for life-threatening arrhythmias, Circulation 103:89–95, 2001.)
In women, even during the postpartum period, genotype is important because the risk is higher for patients with LQT2 than for those with LQT1.24,25 The higher risk for women with LQT2 is probably partly related to sleep disruption; accordingly, it is recommended that the spouses or other caregivers take on some of the night-time feeding of the infants, thus allowing a fair amount of uninterrupted sleep for the women with LQT2.
These significant genotype-phenotype correlations have an impact on management.
Molecular Diagnosis in Long QT Syndrome
Who Should Be Screened for Long QT Syndrome and Medico-legal Implications
Molecular diagnosis should always be attempted whenever LQTS has either been diagnosed or is suspected on sound clinical grounds. When successful (80% to 85% of cases in the author’s laboratory), it allows the rapid screening of all the family members and the identification of all the “silent mutation carriers” (individuals with a disease-causing mutation and a QTc less than 440-ms interval) whose prevalence increases from 10% for LQT3 to 37% for LQT1 patients.26