14 Gastrointestinal and Nutritional Disorders
Pearls
• Abdominal distension can compromise diaphragm expansion and can lead to respiratory insufficiency.
• A child with significant intraabdominal pathology may have subtle clinical signs, such as somnolence and mental status changes.
• Bilious vomiting with a clinical examination suggestive of an acute abdomen can be a sign of a bowel-threatening pathologic condition (e.g., volvulus) and almost always warrants immediate operative intervention.
• If a child does not recover as expected after gastrointestinal surgery, a technical complication of the procedure should be considered as a cause until it is ruled out.
• Correct placement of a nasogastric or orogastric tube is verified with an abdominal radiograph.
• Enteral feedings should begin as soon as possible, because even small quantities of feedings reduce the risk of infection and promote gut healing.
Essential anatomy and physiology
This section summarizes the basic anatomy and physiology of major structures composing the GI tract (Fig. 14-1). See the Chapter 14 Supplement on the Evolve Website for additional information on maturational anatomy and physiology.
Stomach
Secreted mucus forms a protective barrier between the mucosa and the acid and proteolytic enzymes. Acid produced by partial cells creates a gastric pH of 1 to 2 that dissolves food fiber, acts as a bactericide against swallowed organisms, and converts pepsinogen to pepsin. Pepsinogen arises from chief cells. Under the influence of gastric acid, pepsinogen is converted into pepsin, a proteolytic enzyme that continues the breakdown of proteins that was started by gastric acids. Intrinsic factor is a glycoprotein required for vitamin B12 absorption.27
Small Intestine
The small intestine (Fig. 14-2) begins beyond the pylorus of the stomach and is divided into the duodenum (receives enzymes important for digestion), the jejunum (principle absorbing site), and the ileum (the only site for the absorption of vitamin B12 and bile acids). Two layers of smooth muscle, an outer longitudinal layer, and an inner thicker circular layer produce peristalsis.
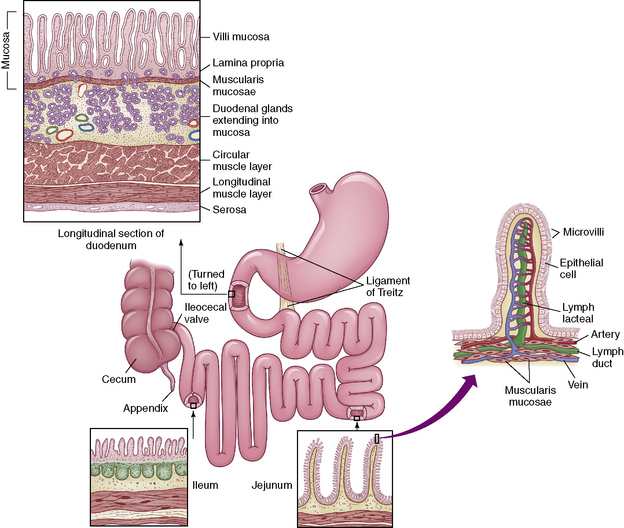
Fig. 14-2 The small intestine.
(From McCance KL, Heuther SE, editors: Pathophysiology: the biologic basis for disease in adults and children, ed 6, St Louis, 2010, Elsevier.)
Liver
The liver is one of the largest organs of the body, and it performs hundreds of functions. It is divided into right and left lobes by the falciform ligament (Fig. 14-3). The right lobe is the largest lobe, composed of the right lobe proper, the caudate lobe (posterior surface), and the quadrate lobe (inferior surface).
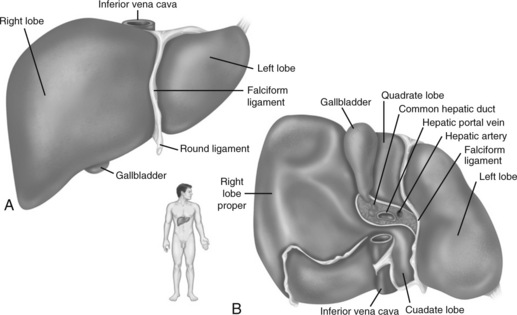
Fig. 14-3 Gross structure of the liver. A, Anterior view. B, Inferior view.
(From Patton KT, Thibodeau GA: Anatomy and physiology, ed 7, St Louis, 2010, Mosby.)
The liver has 50,000-100,000 functional units or lobules composed of hepatic plates (i.e., plates of hepatocytes) that each radiate centrally around a central vein (Fig. 14-4). The porta hepatis is a fissure that serves as the entry point for the hepatic artery, the portal vein, and the common bile duct. The artery, vein, and duct divide into intralobular branches as they follow the septa throughout the liver.
Pancreas
The pancreas is composed of a head, a body, and a tail. The head is tucked into the curve of the duodenum, and the tail reaches laterally across the midline to the spleen. The pancreas is a retroperitoneal organ and is well protected from most environmental impacts. It functions as both an exocrine and endocrine organ. The working cells of the exocrine pancreas are acini cells; these cells are organized as spheres encompassing small secretory ducts scattered throughout the organ. This series of ducts transports fluid containing the digestive enzymes (proteases, amylase, and lipase) and sodium bicarbonate (pH 7.1-8.2) produced by acini cells to the pancreatic duct.27 The pancreatic duct runs the length of the organ and then drains into the common bile duct at the ampulla of Vater, ultimately draining into the duodenum through the sphincter of Oddi.
Nutrition
There is little evidence to identify the best nutritional support of the critically ill child.30,44 A recent Cochrane review that attempted to assess the impact of enteral and parenteral nutrition (PN) on clinically important outcomes in the critically ill child found only one trial that was relevant to the review question.30 Appropriate nutritional assessment, determination of energy requirements, and the timing, route, and type of nutritional delivery have yet to be established for seriously ill or injured children in the critical care unit.
Recommended Daily Nutritional Intake
Adequate energy (caloric) intake is necessary for rapid growth and development throughout childhood. During infancy the requirement for energy intake per kilogram is greater than in all other age groups. The average requirements for energy intake during infancy and childhood are shown in Table 14-1. Of note, these requirements are for healthy, active infants and children.
Table 14-1 Daily Energy Requirements for Infants and Children
Age | kcal/kg |
Up to 6 months | 90-110 |
6-12 months | 80-100 |
12-36 months | 75-90 |
4-6 years | 65-75 |
7-10 years | 55-75 |
11-18 years | 40-55 |
It is useful to divide energy requirements into resting energy expenditure (REE) and requirements for growth and physical activity. REE is determined by the basal metabolic rate or the energy consumed for the normal maintenance of cellular energy. Estimates of an ill child’s REE by standard equations are unreliable, and indirect calorimetry is not always available to clinicians.44 Caloric requirements for the hospitalized child may be substantially more or less than the normal daily recommended requirements. Hypermetabolic states, excessive nutrient losses, trauma, burns, and surgery will increase energy requirements, whereas neurologic impairment, reduced activity, and ventilator support can decrease energy needs. Typically, fever increases energy requirements 12% per day for each degree Celsius elevation in temperature above 37° C.
Fluid and electrolyte requirements vary as a function of age, weight, and clinical condition. Normal daily fluid and electrolyte requirements are listed in Table 14-2. Any calculation of maintenance fluid requirements should use the formulas only to estimate a baseline. The actual volume of fluid administered to the patient must be tailored to the patient’s clinical condition and fluid balance. For additional information about fluid and electrolyte balance, see Chapter 12.
Table 14-2 Formulas for Estimating Daily Maintenance Fluid and Electrolyte Requirements for Children
Daily Requirements | Hourly Requirements | |
Fluid Requirements Estimated from Weight* | ||
Newborn (up to 72 h after birth) | 60-100 mL/kg (newborns are born with excess body water) | – |
Up to 10 kg | 100 mL/kg (can increase up to 150 mL/kg to provide caloric requirements if renal and cardiac function are adequate) | 4 mL/kg |
11-20 kg | 1000 mL for the first 10 kg + 50 mL/kg for each kg over 10 kg | 40 mL for first 10 kg + 2 mL/kg for each kg over 10 kg |
21-30 kg | 1500 mL for the first 20 kg + 25 mL/kg for each kg over 20 kg | 60 mL for first 20 kg + 1 mL/kg for each kg over 20 kg |
Fluid Requirements Estimated from Body Surface Area (BSA) | ||
Maintenance | 1500 mL/m2 BSA | – |
Insensible losses | 300-400 mL/m2 BSA | – |
Electrolytes | ||
Sodium (Na) | 2-4 mEq/kg | – |
Potassium (K) | 1-2 mEq/kg | – |
Chloride (Cl) | 2-3 mEq/kg | – |
Calcium (Ca) | 0.5-3 mEq/kg | – |
Phosphorous (Phos) | 0.5-2 mmol/kg | – |
Magnesium (Mg) | 0.4-0.9 mEq/kg | – |
* The “maintenance” fluids calculated by these formulas must only be used as a starting point to determine the fluid requirements of an individual patient. If intravascular volume is adequate, children with cardiac, pulmonary, or renal failure or increased intracranial pressure should generally receive less than these calculated “maintenance” fluids. The formula utilizing body weight generally results in a generous “maintenance” fluid total.
Macronutrient Metabolism and Requirements
Recommended daily requirements of carbohydrate, fat, and protein are summarized in Table 14-3. The percentage of fat required in younger children is usually greater than requirements in older children. Disease states including renal and liver failure may decrease the amount of protein needed.
Table 14-3 Caloric Distribution of Daily Macronutrient Intake
Macronutrient | Percent of Total Calories (%) |
Carbohydrates | 40-70 |
Fat | 20-50 (40-50 in infants) |
Protein | 7-15 |
Enteral Nutrition
Enteral feeding is preferable to parenteral nutrition, because enteral feedings will maintain gut structure and function and will reduce complications and cost. Specific benefits include intestinal trophism and preservation of the gut barrier to minimize bacterial translocation.38
The practice of aspirating residual liquid and refeeding it is controversial. Although a large volume of residual feeding that remains in the stomach may contribute to inadvertent aspiration,45 the residual volume that should be considered significant has not been clearly established. Nurses should measure and record the volume of residual feeding and notify the on-call provider according to unit policy or orders. Enteral feeding delivery devices, routes, placement methods, nursing considerations, and complications are summarized in Table 14-4.
Parenteral Nutrition (PN)
Nursing Responsibilities
The nurse must closely monitor the child’s clinical appearance and fluid and electrolyte balance to prevent and detect complications of PN as soon as possible. This monitoring requires documentation of the quantity and content of the child’s fluid intake and output and evaluation of the child’s fluid and electrolyte status and daily weight. A sample monitoring schedule for children receiving PN is provided in Table 14-5.
Table 14-5 Sample Monitoring Schedule for Children Receiving Parenteral Nutrition: Must Be Tailored to Child’s Clinical Status
Monitoring | Frequency |
General and Anthropometric Measurements | |
Vital signs | Every 4 hours, or more often as patient condition warrants |
Weight | Daily |
Strict intake and output | Constant |
Caloric intake | Daily |
Height | Weekly |
Head circumference | Weekly (for children younger than 2 years) |
Blood Sampling | |
Glucose | Initial + Daily until stable (more often in neonates) |
Electrolytes | Initial + Daily until stable |
BUN, Creatinine | Initial + Weekly |
Ca2+/ionized calcium, ![]() |
Initial + Weekly (![]() |
Alkaline phosphatase, AST, ALT | Initial + Weekly |
Total and direct bilirubin | Initial + Weekly |
Total protein, albumin | Initial + Weekly |
Prealbumin | Initiation |
Triglycerides, cholesterol | Weekly |
Zinc, copper, selenium, manganese, iron | Monthly |
Glucose point-of-care testing | When PN is abruptly discontinued, cycled, or if signs or symptoms of hypoglycemia or hyperglycemia suspected |
CBC count with differential | When febrile |
Blood culture | When febrile |
ALT, Alanine aminotransferase; AST, aspartate aminotransferase; BUN, blood urea nitrogen; Ca2+, calcium; CBC, complete blood count; Mg2+, magnesium; PN, parenteral nutrition; phosphorous.
Potential complications of PN include electrolyte abnormalities, infection, cholestasis leading to hepatic dysfunction, and hyperlipidemias (with triglyceride administration). In critically ill children, there is an association between hyperglycemia and organ dysfunction.33
Because PN solution contains a high concentration of glucose, it provides an excellent medium for bacterial growth. When hanging a new bag of PN solution and tubing, the nurse must use strict aseptic technique to prevent line contamination. Many hospitals require that the entire tubing system (between PN solution and the patient, including infusion pump tubing) be changed every 24 hours to decrease the possibility of significant bacterial growth. The dressing over the catheter insertion site should be changed when it is no longer occlusive (per hospital policy), using sterile technique and an occlusive dressing. The nurse should assess the catheter insertion site for evidence of inflammation (erythema or exudate) and should report any abnormalities to the appropriate provider. Wound exudate should be cultured and sent for gram stain analysis as ordered (or per protocol). Nurses should be careful to follow the healthcare institutional policies and protocols to prevent central venous catheter-associated blood stream infections (see Chapter 22, Box 22-6 for further information).
Because serum magnesium, phosphate, and calcium levels may fall during PN therapy, providers will monitor the concentration of these elements at least weekly. Trace element deficiencies are more likely to develop with long-term PN therapy or when PN therapy is used in premature infants. The signs and symptoms of copper deficiency include anemia, neutropenia, loss of taste (obviously difficult to assess), and rash. Zinc deficiency can produce an erythematous maculopapular rash (called acrodermatitis enteropathica) over the face, trunk, and digits; poor wound healing; hair loss and loss of taste; and a functional ileus.62 A chromium deficiency can produce a diabetes-like syndrome.62
Because the central venous PN catheter is inserted into a relatively large vein, a loose or cracked tubing connection can rapidly result in significant loss of blood (i.e., hemorrhage). It is important that the nurse check all tubing and catheter connections at least every hour. Because insertion of a central venous catheter creates risk of significant complications, the nurse must ensure that the catheter is secured in place with no possibility of dislodgement. If catheter misplacement or migration is suspected, a chest radiograph may be needed to verify placement (for further information, see Chapter 10).
Cholestatic jaundice is a serious but incompletely understood complication of PN that is associated with periportal fibrosis, bile duct proliferation, and bile stasis. This complication contributes to morbidity and mortality in younger infants and children with short gut syndrome (SGS) following intestinal resection.10 The first sign of cholestatic jaundice is elevation in concentrations of liver enzymes (alanine aminotransferase [ALT] and aspartate aminotransferase [AST]). In addition, the bilirubin will begin to rise and the child may appear jaundiced; cholestasis is defined as a direct bilirubin greater than 2 mg/dL. Risk of cholestatic jaundice can be reduced by cycling and limiting lipid administration to 1 g/kg per day or using alternate day dosing of the fat emulsion. If cholestasis is present, treatment includes administration of ursodeoxycholic acid.
Common clinical conditions
Intestinal Failure
Pathophysiology
Each child with intestinal failure is unique, because there are no absolute criteria for the amount and type of bowel needed to sustain absorption of nutrients for appropriate growth. The normal estimated bowel length at birth is 250 ± 40 cm.22 After the loss of a bowel segment, the intestine undergoes a process termed intestinal adaptation. As a general rule, infants can experience acceptable intestinal function with less than 15 cm of intestine if the ileocecal valve is intact, and with 30 to 45 cm of intestine if the ileocecal valve is absent or does not function.17 It is unlikely that children with less than 20 cm of remaining bowel will be able to grow and develop with enteral nutrition alone. Intestinal transplantation may offer hope to these patients.
Intestinal adaptation is characterized by increasing intestinal mass, lengthening of villi, and improved absorption at the epithelial level.10,22 This process allows for the remaining intestine to compensate for the loss of the bowel by increasing its surface area and functional abilities.10 Successful adaptation is described as the ability to achieve normal growth, fluid balance, and electrolyte concentration without PN.53 The time frame required for adaptation is unclear and dependent on the etiology of the SBS and the functional state of the remaining bowel, although adaptation can occur over weeks to months.10,22 Children have been transitioned to enteral feedings exclusively over periods as long as 8 years. There are a number of metabolic derangements that occur after loss of bowel that are summarized in Table 14-6.
Table 14-6 Metabolic Derangements and Consequences in Children with Short Gut Syndrome
Derangements | Consequences |
Early | |
Gastric hypersecretion | Peptic ulceration |
Dumping syndrome | Diarrhea, hyperglycemia, reactive hypoglycemia |
Rapid intestinal transit | Nutrient malabsorption |
High output from enterostomies | Electrolyte disturbances |
Late | |
Bile and fatty acid malabsorption | Gallstones, steatorrhea |
Bowel dilation and stasis | Bacterial overgrowth syndrome, D-lactic acidosis |
Anastomotic ulceration | Gastrointestinal bleeding |
From Cohran VC and Kocoshis SA: Short bowel. In Baker S, Baker R, David A, editors: Pediatric nutrition support, Sudburry, MA, 2007, Jones and Bartlett.
Management
Often 20 Kcal/ounce feedings are initiated and can be advanced to higher caloric density (to as high as 30 Kcal/ounce [1 Kcal/mL]) as tolerated. Usually these children are fed with casein hydrolysate formulas and elementary amino acid-based formulas such as Pregestimil (Mead Johnson, Evansville, IN), EleCare (Abbott Nutrition, Columbus, OH), and Neocate (Nutricia North America, Gaithersburg, MD) in infants and Peptamen Jr (Nestle Nutrition, North America, Minneapolis, MN) and Neocate Jr.(Nutricia North America, Gaithersburg, MD) in older children. These formula types are easier to digest and are less likely to trigger an immune response, so they will enhance bowel adaptation.10
When children are unable to achieve appropriate growth with enteral feedings, they may be considered surgical candidates for procedures to restore normal bowel diameter, lengthen the bowel, or both. The goals of surgical intervention include reducing stasis, improving motility, and increasing the effective mucosal surface area.29 The most common short bowel procedures are the Bianchi, Kimura, and the serial transverse enteroplasty procedure.
The Bianchi procedure is the oldest procedure and involves a longitudinal incision to create two tubes to lengthen the bowel (Fig. 14-5). The Kimura procedure (Fig. 14-6) is an alternative procedure for patients with SGS and inadequate mesentery who are not candidates for the Bianchi procedure. The serial transverse enteroplasty (STEP) procedure augments bowel length by stapling dilated bowel in a zigzag fashion to achieve more effective bowel surface area (Fig. 14-7).
With advances in surgical techniques and immunosuppressive therapy, intestine transplantation is successful as an isolated procedure and when combined with transplantation of other organs.54 A major reason for the increased success of intestinal transplantation is the availability of more potent immunosuppressants such as tacrolimus (Prograf). The major limiting factor that prevents widespread use of transplantation is the lack of available organs for transplantation. Because children with less than 15 cm of bowel are not likely to tolerate enteral feeding and thrive, they should be referred to an intestinal rehabilitation and transplant program as soon as possible.
The ideal intestine donor has the same blood type, weighs within 10% of the recipient’s body weight, and is close in age to the recipient. All intestine recipients have a stoma to allow for bowel surveillance and access for endoscopy and biopsy. Figure 14-8 shows the technical details of isolated intestine transplant procedure and intestine transplant in combination with other organs.54
Critical care goals are to support the patient to be hemodynamically stable, with adequate oxygenation and ventilation, free of requirement for mechanical ventilation support. Airway clearance and spontaneous ventilation may be ineffective as a result of the lengthy abdominal surgical procedure (and resultant need for anesthetic administration during the long procedure), visceral edema, and pain. With the postoperative resolution of coagulopathies, aggressive chest physiotherapy is initiated. Intestine recipients generally require mechanical ventilation longer than isolated liver recipients (see Liver Transplantation in Chapter 17), because intestine recipients typically are in poor health before the transplant and often have a precarious fluid balance. Additional challenges include open abdominal wounds and potential need for surgical reexploration for complications such as perforation or bleeding.
Post-transplant lymphoproliferative disorder (PTLD) is one of the most underrated complications of immunosuppression. PTLD is the development of continually proliferating B-lymphocytes, presumably under the influence of Epstein-Barr virus. Diagnosis is made by clinical examination and histologic review. Clinical signs and symptoms include fever, lymphadenopathy, GI symptoms, and weight loss. If the biopsy specimen is diagnostic for PTLD, treatment includes holding or reducing immunosuppression and initiating IV antiviral therapy and immunoglobulin therapy (see Chapter 17 for additional information).
Gastrointestinal Bleeding
Etiology and Pathophysiology
GI bleeding in children can result from inflammation of the intestine, congenital or acquired visceral or vascular anomalies, trauma, esophageal varices, ulcers, or coagulopathies. The incidence of acquired GI bleeding in critically ill children receiving mechanical ventilation has been reported to be as high as 51.8% in some series.14,47 Identified risk factors include a pediatric risk of mortality 2 score of 10 or higher, operating room procedures longer than 3 hours, hepatic insufficiency, coagulopathy, respiratory failure, and high-pressure ventilator settings of greater than or equal to 25 cm H2O.14,47
Lower GI bleeding is the result of mucosal disruption distal to the ligament of Treitz and can be caused by Crohn’s disease, intussusception, or ischemic injury. Common causes of GI bleeding in children are listed in Table 14-7.
Table 14-7 Causes of Gastrointestinal Bleeding in Infants and Children
Age Group and Status | Upper GI Bleeding | Lower GI Bleeding |
Healthy neonate | Swallowed maternal blood, hemorrhagic disease of the newborn, esophagitis, gastric duplication | Swallowed maternal blood, infectious colitis, milk allergy, hemorrhagic disease of the newborn, duplication of the bowel, Meckel’s diverticulum, anal fissure |
Sick neonate | Stress ulcer, gastritis, vascular malformations | Necrotizing enterocolitis, infectious colitis, disseminated coagulopathy, midgut volvulus, intussusception |
Infancy | Stress ulcer, esophagitis, gastritis, gastric duplication | Anal fissure, infectious colitis, milk allergy, nonspecific colitis, juvenile polyps, intestinal duplication, intussusception, Meckel’s diverticulum |
Preschool age | Esophagitis, gastritis, stress ulcer, peptic ulcer disease, foreign body, caustic ingestion, vascular disease (Rendu-Osler-Weber disease, hemophilia), trauma, portal hypertension | Infectious colitis, juvenile polyps, anal fissure, intussusception, Meckel’s diverticulum, angio-dysplasia, Henoch-Schönlein purpura, hemolytic-uremic syndrome, inflammatory bowel disease |
School age and adolescence | Esophagitis, gastritis, stress ulcer, peptic ulcer disease, portal hypertension, trauma | Infectious colitis, inflammatory bowel disease, polyps, angiodysplasia, Henoch-Schönlein purpura, hemolytic-uremic syndrome, hemorrhoids, rectal trauma |
GI, Gastrointestinal.
Data from Arensman RM, Browne M, Madonna MB: Gastrointestinal bleeding. In Grosfeld JL et al, editors: Pediatric surgery, ed 6, Philadelphia, 2006, Mosby; Martin SA, Simone S: Gastrointestinal system: In Slota MC, editor: Core curriculum for pediatric critical care nursing, ed 2, St Louis, 2006, Mosby-Elsevier.
Microscopic bleeding may cause no symptoms and may be detectable only through analysis of GI secretions or feces. Significant GI bleeding may result in hypovolemia and low cardiac output, shock, and death (see also Shock, in Chapter 6). See the section on Gastrointestinal Bleeding in the Chapter 14 Supplement on the Evolve Website for additional information about the pathophysiology of GI hemorrhage.
Clinical Signs and Symptoms
Metabolic acidosis and a rise in serum lactate may be noted. Oxyhemoglobin desaturation may not be present or detected by pulse oximetry, because existing hemoglobin may be saturated with oxygen. The oximeter device may have difficulty detecting a signal if the child’s pulses are weak. The nurse should notify an on-call provider immediately of these findings, because the patient’s status is critical (see Chapter 6 for more information about recognition and treatment of shock).
During the first days of life, the Apt test (named for Leonard Apt) may be performed to distinguish between swallowed maternal blood and GI bleeding as a cause of blood in the newborn’s stool.4 The Apt test is performed by placing blood from the neonate on filter paper with 1% sodium hydroxide (a reagent that reacts with fetal hemoglobin).4 Maternal blood will appear rusty brown, whereas the neonate’s blood that contains fetal hemoglobin will remain pink or red.4 The pink or red color is a positive result, indicative of presence of blood from the neonate.
Management
The three phases of management of the child with GI bleeding are resuscitation, specific diagnosis, and specific treatment. A diagnostic algorithm for upper GI bleeding is presented in Fig. 14-9.
During resuscitation and replacement of intravascular volume, nursing observations may help to determine the source of the child’s bleeding. If saline lavage through a nasogastric tube reveals grossly bloody or red-tinged aspirate, ongoing upper intestinal bleeding is present. Nursing interventions during resuscitation of the child with GI bleeding are summarized in Box 14-1.
Box 14-1 Nursing Interventions During Resuscitation of the Child with GI Bleeding
1. Assess and support airway, breathing, and oxygenation. Optimize oxygenation and provide supplementary oxygen as indicated. If the child’s level of consciousness is decreased, the child will likely require insertion of an advanced airway and support of ventilation.
2. Restore adequate intravascular volume. Establish vascular (intravascular or intraosseous) access. Because the rate of intravenous fluid replacement will be limited by the size of the vascular catheter, insert the largest catheter possible. It is preferable to establish two vascular catheters to allow one catheter to be used for rapid volume expansion while the other is used for administering medication and measuring the child’s CVP.
3. Assess systemic perfusion. Monitor arterial and central venous pressures and systemic perfusion.
4. Insert a urinary catheter to monitor urine output.
5. Obtain blood samples for frequent (at least every 4 h) measurement of hematocrit or complete blood count; notify an on-call provider if either falls.
6. Administer blood volume expanders or packed red blood cells as ordered. Children with active gastrointestinal bleeding should have an active type and cross match with blood available at all times.
7. Assess for signs of further hemorrhage, including signs of poor systemic perfusion, abdominal pain or tenderness, changes in bowel sounds, hematemesis, or hematochezia.
8. Administer antibleeding pharmacologic agents as prescribed. See Table 14-8 for specific drug information.
Proton pump inhibitors and histamine-2 receptor antagonists are the most commonly used pharmacologic agents to prevent the development of GI bleeding (Table 14-8). Although only a small number of critically ill children will develop gastric bleeding, the associated morbidity justifies the common practice of prophylaxis.
Hyperbilirubinemia
Etiology
When the neonate’s TSB is elevated, the bilirubin can cross the blood-brain barrier, causing acute bilirubin encephalopathy or the chronic form of bilirubin encephalopathy, kernicterus. Kernicterus is a yellow staining and brain tissue damage with degenerative lesions, resulting from central nervous system exposure to high concentrations of unconjugated bilirubin. Hyperbilirubinemia is commonly associated with prematurity, breast feeding, and other factors summarized in Box 14-2.
Box 14-2 Risk Factors for Hyperbilirubinemia in the Neonate
Factors That Increase Risk
• Jaundice in first 24 h (usually related to hemolysis)
• Visible jaundice before hospital discharge or TSB or TcB level in the high-risk zone
• Hemolytic disease: blood group incompatibility, Rh incompatibility, glucose-6-phosphate dehydrogenase deficiency
• Prematurity (less than 35 weeks’ gestation)
• Cephalohematoma or significant bruising
• Sibling previously received phototherapy (possible genetic cause)
• Ethnicity: East Asian, certain Native American tribes, and Greek ancestry
• Maternal age greater than 25 years
Data from American Academy of Pediatrics Subcommittee on Hyperbilirubinemia: Management of hyperbilirubinemia in the newborn infant 35 or more weeks of gestation, Pediatrics 114:297, 2004; Watson RL: Hyperbilirubinemia. Crit Care Nurs Clin N Am 21:97, 2009.Rh, Rhesus (factor); TcB, transcutaneous bilirubin; TSB, total serum bilirubin.
In the first week of life, it is estimated that approximately 60% of normal newborns will become clinically jaundiced.42 Newborns are now discharged from the hospital at approximately 24 to 48 hours after birth, before the typical TSB peak at 48 to 96 hours. As a result, hyperbilirubinemia is the most common reason for hospital readmission of the neonate. A sentinel event alert was issued by the Joint Commission in 2001, because an increased number of cases of kernicterus were being diagnosed in otherwise healthy newborns.
Pathophysiology
When RBCs reach the end of their 120-day life span, they normally are sequestered in the spleen. The cells are destroyed, and the heme portion of the hemoglobin molecule is oxidized, and bilirubin is formed. Bilirubin is bound to albumin in the plasma and taken up in the liver, where it is combined with a sugar through the action of the enzyme, bilirubin uridine diphosphate glucuronosyltransferase, making conjugated bilirubin.11 Conjugated bilirubin is water soluble, it cannot cross the blood-brain barrier, and it is normally excreted in bile (Fig. 14-10). Free bilirubin, called unconjugated (indirect) bilirubin, is lipid soluble and not water soluble. Because unconjugated bilirubin is thought to diffuse freely into the brain, high concentrations of this form of bilirubin may be neurotoxic and cause kernicterus.
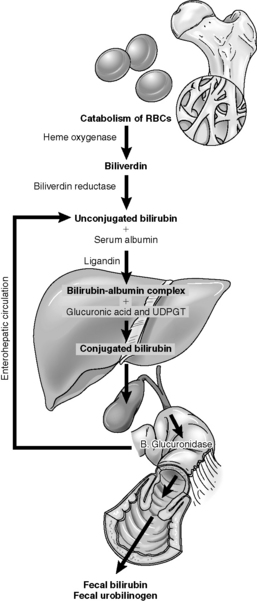
Fig. 14-10 The pathophysiology of neonatal hyperbilirubinemia.
(From Colletti JE, et al: An emergency medicine approach to neonatal hyperbilirubinemia. Emerg Med Clin N Am 25:1117-1135, 2007.)
Kernicterus is yellow (bilirubin) staining of the basal ganglia in the brain of neonates with severe jaundice. Although the precise mechanisms responsible for the entry of bilirubin into the brain are not known, disruption of the blood brain barrier from increased permeability (e.g., caused by hyperosmolarity or severe asphyxia), prolonged transit time (e.g., caused by increased central venous pressure [CVP]), or increased blood flow (e.g., caused by hypercarbia and acidosis) are thought to be contributory.63
The neurotoxic sequelae of hyperbilirubinemia are the most worrisome, and a grading for acute bilirubin encephalopathy has been proposed. The earliest signs may include alteration in the tone of extensor muscles (hypotonia or hypertonia), retrocollis (backward arching of the neck), opisthotonus (backward arching of the trunk), and a poor suck.3 The early symptoms may intensify and be accompanied by a shrill cry and unexplained irritability alternating with lethargy.3 Therapy at this stage may prevent advancement of symptoms. Cessation of feeding, irritability, seizures, and altered mental status are later symptoms of acute bilirubin encephalopathy. The final stage is kernicterus and may be irreversible with the development of cerebral palsy, deafness or hearing loss, and impairment of upward gaze. Kernicterus has a significant mortality (at least 10%) and long-term morbidity (at least 70%), and most cases are reported in infants with TSB greater than 20 mg/dL.28
Noninvasive (transcutaneous) bilirubin measurements can be obtained with newer instruments. Published data suggest that in most infants the measurements are within 2 to 3 mg/dL of serum measurements and can replace TSB measurements in most cases.2 In infants undergoing phototherapy, this measurement is not reliable and should not be used.
It is possible to measure serum levels of total bilirubin and direct (or conjugated) bilirubin. Current recommendations caution against inferring the indirect (unconjugated) bilirubin from the TSB, because the difference between the total and direct bilirubin is not constant. Current guidelines recommend that providers evaluate the TSB level63; the higher the TSB, the greater the risk of bilirubin encephalopathy. Treatment is indicated for infants with bilirubin levels in excess of 20 mg/dL.
Management
The current American Academy of Pediatrics (AAP) recommendations for phototherapy are based on age and TSB levels (see Evolve Fig. 14-1 in the Chapter 14 Supplement on the Evolve Website to view a graphic representation of recommended therapies based on infant age and total serum bilirubin concentration). Prophylactic phototherapy is often instituted for neonates weighing less than 1500 g. Although exclusive breast feeding can contribute to high TSB levels, the AAP recommends that breast feeding be continued with 10 to 12 feedings recommended per day, plus administration of supplementary intravenous fluid to treat dehydration.2
Phototherapy converts bilirubin to a water-soluble form that can be excreted without glucuronidation. Phototherapy units contain day lights, fluorescent tubes, fiberoptic light, and halogen bulbs. The effectiveness of phototherapy is determined by the type of light, the infant’s distance to the light, and the amount of exposed body surface area. Light emitted at a wavelength in the blue-green spectrum of 425 to 495 nm is thought to be most effective because of the optical qualities of bilirubin and the skin. The AAP recommends the use of special blue fluorescent lamps or light-emitting diodes.41,42
The duration of phototherapy required is affected by the TSB level, the cause of the hyperbilirubinemia, and the infant’s age. For infants readmitted for phototherapy during the first days of life, the treatment is usually discontinued when TSB levels reach 13 to 14 mg/dL. It is not uncommon for infants to experience a rebound increase in TSB level of 1 to 2 mg/dL when the therapy is discontinued.41
Portal Hypertension
Etiology
Portal hypertension is an increase in portal venous pressure above 5 to 10 mmHg. It is caused by obstruction to the normal flow of blood through the portal venous system, the liver sinusoids, or the hepatic vein from the mesenteric vascular bed (small and large intestine, stomach, spleen, and pancreas).61 It may be caused by (1) obstruction of the portal vein or its immediate tributaries (this is a form of extrahepatic or prehepatic portal hypertension), (2) an increase in vascular resistance within the liver that occurs secondary to fibrosis of the liver (this form is called intrahepatic or hepatocellular portal hypertension), or rarely (3) obstruction of hepatic venous outflow into the inferior vena cava (this is a form of suprahepatic or posthepatic portal hypertension).
Intrahepatic portal hypertension can complicate any form of chronic liver disease, including neonatal or childhood hepatitis, biliary atresia, congenital hepatic fibrosis, or liver disease secondary to infection or metabolic diseases (e.g., alpha1-antitrypsin deficiency). Suprahepatic portal hypertension may be caused by inferior vena cava (IVC) obstruction, hepatic vein occlusion, thrombosis from Budd-Chiari syndrome, or stenosis of the hepatic vein orifice.61
Pathophysiology
Impedance to portal blood flow and hypertension in the portal and splanchnic circulation promote the formation of collateral vessels between the systemic and portal circulations and the IVC, or other major central veins (Fig. 14-11). Major collateral vessels form from the portal vein, along the stomach and esophagus to the intercostal veins, in the paraumbilical veins (causing enlarged abdominal wall vessels), and around the rectum and anus (in the hemorrhoidal veins). Submucosal veins of the esophagus often enlarge and form collateral vessels between the portal venous system and vena cava. These enlarged veins often protrude into the esophagus and are known as esophageal varices.
Clinical Signs and Symptoms
The mortality rate for patients with bleeding esophageal varices was previously high; however, current treatment with vasopressin and somatostatin, correction of coagulopathy, endoscopic sclerotherapy and ligation (banding), and balloon tamponade (with a Sengstaken-Blakemore tube) are generally effective in controlling bleeding in children.55 Balloon tamponade requires skilled personnel and is reserved for uncontrollable bleeding in larger patients.
Management
Bleeding from esophageal varices is a life-threatening complication of portal hypertension. The goals of initial management are to maintain intravascular volume and systemic perfusion and to stop active bleeding (Box 14-3). For patients with portal hypertension from intrahepatic causes, there is a higher mortality when the initial bleeding episode is associated with liver dysfunction, malnutrition, and coagulopathy.
Box 14-3 Management of Hemorrhage Associated with Portal Hypertension
Resuscitation
• Establish intravenous access and appropriate hemodynamic monitoring (e.g., arterial and central venous monitoring).
• Provide isotonic intravenous crystalloid boluses (20 mL/kg and repeat as needed) and blood (10 mL/kg as needed). Warm blood products before administration in infants and young children (or per hospital policy).
• Evaluate systemic perfusion frequently.
• Assess for signs of ongoing hemorrhage (abdominal pain, changes in bowel sounds, hematemesis, or hematochezia).
From Lin PW, Stoll BJ: Necrotizing enterocolitis. Lancet 368:1271-1283, 2006.
If bleeding from esophageal varices persists after correction of coagulopathies and volume resuscitation, a continuous IV infusion of vasopressin or somatostatin analog (octreotide acetate) can be initiated (see Table 14-8).
During endoscopic sclerotherapy, the endoscopist injects a sclerosing agent such as 5% ethanolamine, 1 to 1.5% tetradecyl sulfate, or 5% sodium morrhuate either into or around bleeding esophageal varices.55 The resulting edema and thrombosis stops bleeding in most patients (Fig. 14-12). Esophageal varices can be obliterated completely with repeated injections at 2 to 4-week intervals, thus preventing recurrent episodes of bleeding. Potential complications of this technique include exacerbation of bleeding, esophageal stricture, and esophageal perforation.43
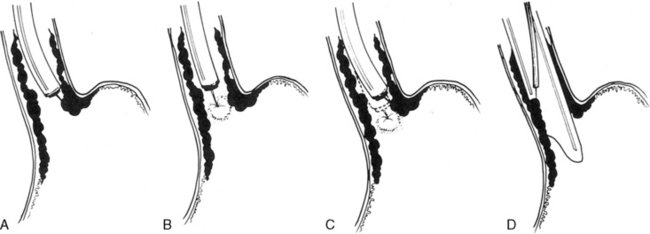
Fig. 14-12 Techniques of injection sclerotherapy.
(From Terblanche J, Burroughs AK, Hobbs EKF. Controversies in the management of bleeding esophageal varices. N Engl J Med 320:1393-1398, 1989.)
A pediatric Sengstaken-Blakemore tube (Fig. 14-13) can be inserted at the onset of the bleeding. Although this tube is usually effective in controlling bleeding, it is used infrequently because the complication rate is high. The tube must be inserted by skilled, experienced personnel, and the child typically requires arterial pressure monitoring and airway control with endotracheal intubation. Complications include pulmonary aspiration, discomfort, and pressure necrosis of the distal esophagus. When a Sengstaken-Blakemore tube is used, bedside providers should constantly monitor the tube function, placement, and integrity. In many instances bleeding reoccurs after the tube is removed. See the Chapter 14 Supplement on the Evolve Website, Portal Hypertension, Management, for additional information on The Sengstaken-Blakemore Tube.
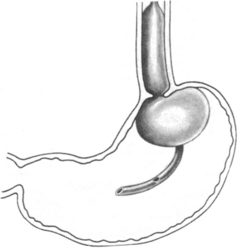
Fig. 14-13 Positioning of a Sengstaken-Blakemore tube.
(From Given B, Simmons S: Gastroenterology in clinical nursing, ed 4, St Louis, 1984, Mosby.)
The Rex shunt is an option for surgical correction of extrahepatic portal vein obstruction. The word Rex refers to the anatomic name of the portion of the liver to which the blood is shunted. The Rex shunt uses an autologous vein, most commonly the left jugular vein; this vessel extends from the superior mesenteric vein to the left branch of the portal vein.13 To prevent clotting of the shunt, heparin is initially provided. When oral intake is tolerated, anticoagulation therapy is transitioned to baby aspirin and dipyridamole (Persantine). The duration of this anticoagulation is variable, and is determined by the size of the child, the size of the graft, and the presence of any hypercoagulable conditions. For further information, readers are referred to websites about this procedure (e.g., http://www.childrensmemorial.org/depts/siragusa/transplant-surgery/rex-shunt.aspx)
Additional shunting procedures include the splenorenal shunt and the mesocaval shunt. The most popular shunting procedure performed in children is the distal splenorenal shunt, which results in anastomosis of the splenic vein to the left renal vein (Fig. 14-14). An alternative procedure, the mesocaval shunt, is occasionally performed; it redirects blood from the obstructed mesenteric system to the patent intrahepatic system.
Ascites
Pathophysiology
Congestive heart failure may produce ascites if the CVP rises sufficiently. Initially, hepatic venous and sinusoidal pressures rise, and the veins and sinusoids expand to accommodate a greater blood volume. This venous congestion causes one of the earliest signs of congestive heart failure in children—hepatomegaly (see Congestive Heart Failure in Chapter 8). If the CVP continues to rise, the liver’s storage capacity for blood is exceeded, and fluids and proteins will exude into the abdominal cavity, creating ascites.
Spontaneous bacterial peritonitis occurs in children with ascites from cirrhosis.35 Chylous ascites develops secondary to lymphatic obstruction at the level of the cisterna chyli or above.
Clinical Signs and Symptoms
If the ascites is secondary to liver, cardiovascular, or renal disease, then the child will exhibit signs of the primary disease and the ascites. For further information, see the appropriate sections in this chapter (e.g., Liver Failure), in Chapter 8 (Congestive Heart Failure) and in Chapter 13 (Acute Renal Failure).
Management
Most cases of chylous ascites resolve without surgical intervention. The goal of treatment is to reduce lymphatic flow. For mild cases, reduce enteral fat intake with or without the use of medium chain triglyceride. Some patients require a period of no enteral intake and PN. In others, the addition of somatostatin will be effective. Palliative procedures to reduce fluid include decompression with paracentesis or placement of an intraabdominal drain and thoracentesis. After paracentesis, monitor the child closely for complications such as hypotension (large shifts of intravascular volume) and bacterial peritonitis.35 Surgical decompression using a peritoneal-jugular shunt may necessary.
Bowel Obstruction
Etiology
Bowel obstruction can be mechanical or functional in origin, and it can occur anywhere along the length of the GI tract. Mechanical obstruction is a physical barrier that blocks the lumen of the GI tract, so that peristaltic contractions are unable to propel GI contents forward. Four main causes of mechanical bowel obstruction are: (1) congenital GI malformations (e.g., duodenal atresia, annular pancreas), (2) intraluminal obstruction (e.g., foreign body, stool, bezoars [masses of organic or inorganic material—often swallowed material—trapped in the gastrointestinal system]), (3) intramural lesions within the bowel wall (e.g., tumors, Crohn’s disease, webs), and (4) extramural causes (e.g., bowel adhesions, hernias, intestinal volvulus).32
Clinical Signs and Symptoms
Obstructions may be complete or partial and intermittent. Complete obstruction stops all progression of bowel contents, but patients will evacuate distal contents before all output ceases. Many patients with complete obstruction will develop bowel ischemia.46 Patients with partial or intermittent obstructions will pass some air and liquid stool (diarrhea and flatus).
Abdominal plain radiographs are the first choice for diagnostic imaging when bowel obstruction is suspected. The normal bowel gas pattern includes a paucity of air in the small bowel, but air is normally visible in the colon and rectum (Fig. 14-15, A). With obstruction, bowel proximal to the obstruction dilates as air and fluid accumulate, causing air fluid levels that are visible in the abdominal plain radiographs (Fig. 14-15, B). The colon and rectum have little or no air, so they may not be visible. The more distal the obstruction, the higher the visible air fluids levels (Fig. 14-15, C). With complete obstruction a paucity of air is visible distal to the obstruction and a cutoff point maybe evident. It is difficult to differentiate between large and small bowel obstruction on the basis of plain abdominal radiographs in infants.
Management
Surgery is often required for the management of bowel obstruction and may be required on an emergent basis to avoid loss of bowel. Emergent surgery is indicated in the setting of complete obstruction (e.g., midgut volvulus, unsuccessful reduction of intussusception, incarcerated hernia) or when the patient demonstrates signs of bowel ischemia and peritonitis. Patients without clinical signs of septic shock, peritonitis, or bowel ischemia can be treated safely with nasogastric decompression and fluid resuscitation before surgical intervention. Partial obstructions can be treated initially with decompression that may resolve the obstruction.46
Care of the child after abdominal surgery
Management
Postoperatively the nurse should assess the child’s pain and administer adequate pain medication to maintain comfort and to prevent splinting of the abdominal incision. Typically an intravenous opioid is administered either as an intermittent bolus or a continuous infusion with or without a patient-controlled mode. For further information about analgesia, see Chapter 5.
Specific diseases
Congenital Gastrointestinal Abnormalities
Failure to pass air through the GI tract immediately after birth is an early sign of a GI anomaly. During the first breath, air enters the stomach and lungs. The gastric air normally is passed through the GI tract in a predictable sequence. With the second breath, air reaches the duodenum. At 6 hours of life air reaches the cecum, and at 24 hours of life air reaches the rectum. In most newborns with abdominal obstruction, plain abdominal radiographs (with air as the contrast) will outline the obstruction (see Fig. 14-15).
Congenital anomalies of the GI tract are listed in Table 14-9. Because neonatal critical care is not a focus of this text, nursing care of the newborn with these anomalies is highlighted but not detailed.
Necrotizing Enterocolitis
Etiology
NEC is an acquired disease of unknown origin that develops during the neonatal period. NEC typically occurs in premature low-birth weight newborns (<1500 g), but cases have been described in term babies.60 Infants with NEC develop necrotic lesions in the GI tract, most commonly in the ileum and colon. The necrosis may be superficial and only detectable microscopically, or it may be transmural and involve both the small and large intestines. Mild disease may be completely reversible, but infants with extensive involvement may not survive or may be rendered as short gut with subsequent intestinal failure following surgical resection of the affected bowel.
A large number of epidemiologic factors have been associated with the development of NEC. The majority (more than 90%) of patients are premature neonates (less than 36 weeks’ gestation), who have received enteral nutrition. Newborns with low-flow states, such as patent ductus arteriosus or congenital heart disease (especially in infants with left ventricular outflow tract lesions), are at risk.20,60 NEC can also develop following repair of gastroschisis. Further discussion of the etiology can be found in the Chapter 14 Supplement on the Evolve Website, Specific Diseases, Necrotizing Enterocolitis.
Pathophysiology
The pathophysiology of NEC is poorly understood but includes: intestinal ischemia, distension, colonization by pathogenic bacteria, subsequent bacterial translocation, and intestinal necrosis and perforation. The proposed inciting mechanisms are schematically presented in Fig. 14-16. The initiating injury in NEC is disruption of the intestinal mucosal epithelium; this disruption can result from a variety of mechanisms, most likely hypoxia or an infection. Most cases occur after the second week of life following bacterial colonization of the intestine.25 See the Chapter 14 Supplement on the Evolve Website, Necrotizing Enterocolitis, for additional information about the role of enteral feeding in the development of NEC.
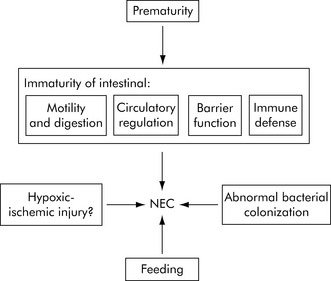
Fig. 14-16 Pathophysiology of necrotizing enterocolitis.
(From Lin PW, Stoll BJ: Necrotizing enterocolitis. Lancet 368:1271-1283, 2006.)
Pneumatosis intestinalis, a radiologic hallmark of well-established NEC, is the accumulation of extraluminal gas in the submucosal intestinal tissue following the invasion of intestinal bacterial flora. It is postulated that progressive infiltration of the mucosa and bowel wall with gram-negative or gram-positive bacteria leads to more extensive tissue inflammation and destruction from activated neutrophils, producing a vasoconstrictive response, with progression to necrosis, and perforation.25
Clinical Signs and Symptoms
The classic clinical presentation of infant NEC includes the symptom group of abdominal tenderness, abdominal distension, hemepositive stools (or other gross or occult signs of GI bleeding), and ileus (decreased bowel sounds and increased gastric residual volume following tube feeding). Abdominal distension is the most commonly observed symptom.25 Bilious vomiting after feeding also may be noted.
Signs of clinical deterioration in the child with NEC include apnea and bradycardia, lethargy, temperature instability, decreased urine output, further abdominal distension and discoloration of the abdominal wall. The wide range of symptoms in NEC has led to a clinical categorization or Bell’s staging of the condition.6 Symptoms and factors utilized in this staging include the infant’s history (and risk factors), nonspecific manifestations of distress, and specific gastrointestinal and radiographic clinical indicators (Box 14-4). Recently, a modification of Bell’s staging has been proposed to include histologic findings of each stage.21
Management
Preventative interventions currently under investigation include: antenatal glucocorticoid administration, preferential use of breast milk, use of a standardized feeding regimen, restricting water intake without compromising hydration, and the use of probiotics (i.e., live microorganisms that may confer health benefits when administered in adequate amounts) and immunoglobulin A and arginine supplementation.37,49,60
If NEC is identified in its early stages and prompt medical treatment is provided, 30% to 50% of affected infants will improve without surgical intervention.25 Once the diagnosis of NEC is suggested, enteral feedings are discontinued and a nasogastric or orogastric tube is passed for gastric drainage and decompression. Umbilical artery or venous catheters are discontinued. The infant will require administration of IV fluid therapy, including PN and appropriate IV antibiotics for both gram-negative and gram-positive organisms. Blood, urine, and sputum cultures should be sent before initiation of antibiotic therapy.
Surgical intervention is indicated if the infant demonstrates evidence of intestinal perforation, erythema of the abdominal wall, persistent metabolic acidosis, or clinical deterioration that is unresponsive to vigorous medical management (Box 14-5).39,51 Surgical options include laparotomy with the resection of the affected bowel and creation of stomas or the placement of a peritoneal drain. See the Chapter 14 Supplement on the Evolve Website, Necrotizing Enterocolitis, for information about treatment with a peritoneal drain.
Biliary Atresia
Etiology
Biliary atresia (BA) is an inflammatory obstruction of the extrahepatic bile ducts with progressive inflammation of intrahepatic bile ducts. The atresia can involve isolated segments of the bile duct system or the entire biliary tree including the gallbladder. The progressive inflammation leads to fibrosis, with eventual bridging fibrosis and cirrhosis. There are two types of BA: the embryonic (fetal) form and the perinatal or acquired form. The embryonic form is usually associated with other congenital anomalies (e.g., polysplenia, cardiac anomalies, malrotation). Eighty percent of patients with biliary atresia in Western countries have the perinatal or acquired form of the disease, in which infants born with a patent hepatobiliary system develop progressive obstruction.40 Precipitating factors include viral, toxic, vascular or immune mediators.40
Clinical Signs and Symptoms
An ultrasound examination may reveal a small noncontractile gallbladder, or the gallbladder is not visualized.18 A radionuclide hepatoiminodiacetic scan will detect movement of a radioactive tracer. The injected tracer is filtered by the liver, which adds it to the bile, so that the tracer normally flows through the bile ducts into the gallbladder and finally into the small intestine. In infants with BA there is no detectable excretion of the isotope into the small intestine.18 A liver biopsy may reveal bile duct proliferation and portal tract fibrosis, which suggest the diagnosis.
Management
When there is significant fibrosis or cirrhosis at the time of operation, the prognosis is poor, even after the Kasai procedure. These patients will demonstrate progressive liver failure postoperatively and will require liver transplantation (see Chapter 17, Overview of Solid Organ Transplantation).
Hepatitis
Etiology
Hepatitis, or inflammation of the liver, is most commonly caused by viral illness. Other causes include autoimmune liver disease, toxins, and idiopathic neonatal hepatitis. Six etiologically and immunologically distinct viruses are known to cause hepatitis: hepatitis A, B, C, D, E, and G. Hepatitis that is not type A, B, or type C is referred to as non-A–non-B–non-C hepatitis and is a diagnosis of exclusion in fulminant hepatic failure when other serologic markers are negative. Other classifications of hepatitis and common viruses that can cause hepatitis are listed in Box 14-6. Idiopathic neonatal hepatitis is considered a distinct form of hepatitis and is not thought to be caused by a virus, but is a histologic descriptive term of giant cell hepatitis.
Hepatitis B
Hepatitis B virus (HBV) is transmitted through blood transfusions or other contact with secretions (including saliva, breast milk, vaginal fluids, and semen) and serum containing HBV. In highly endemic areas of the world, children usually contract the virus through vertical transmission (mother to child); however, in the United States and other developed countries horizontal transmission is more common.58
This form of hepatitis frequently is preceded by a prodrome of arthritis and rash; fever is less common. The onset of hepatitis B is usually insidious and occurs after an incubation period of 60 to 180 days. When infants and young children are infected, they are at high risk (50% to 90%) to become chronically infected; by comparison, only 10% of infected adults become chronically infected.58,59
Hepatitis C
Hepatitis C virus (HCV) is transmitted through blood exposure or transfusion and is less contagious than HBV. In children, vertical transmission at birth (from mother to infant) accounts for most cases.56 The incubation period lasts 6 to 12 weeks, and RNA of the HCV can usually be detected in the serum 2 weeks after infection.56
Clinical Signs and Symptoms
Hepatitis A
Children with HAV may be asymptomatic, but they are contagious (see Hepatitis and Evolve Fig. 14-3 in the Chapter 14 Supplement on the Evolve Website for more details)
Hepatitis B
Children with HBV are less likely than adults to exhibit symptoms. Chronically infected children are usually asymptomatic unless they develop cirrhosis or hepatocellular carcinoma. In addition to general symptoms, children may develop extrahepatic symptoms including glomerulonephritis, papular acrodermatitis (papular rash of the face, extremities, and trunk), and lymphadenopathy.58,59 For further information about clinical course of HBV, see Hepatitis and Evolve Fig. 14-4 in the Chapter 14 Supplement on the Evolve Website.
Laboratory Tests
The hepatitis serologies (antigens and antibodies) are used to diagnose HAV, HBV, HCV, and the disease stage and to identify contaminated units of blood. If the critically ill patient has serologic markers, the nurse should be knowledgeable about implications for the patient’s possible disease state and transmission of the infection. A brief summary of the clinical significance of these markers and their interpretation is included in Table 14-10.
Table 14-10 Hepatitis Virus Serum Markers and Interpretation
Virus, Virus Component, or Antibody | Abbreviation | Interpretation |
Hepatitis A Virus | HAV | |
Antibody (IgM subclass) directed against HAV | Anti-HAV IgM | Current or recent infection; present for 4-6 months |
Antibody (IgG subclass) directed against HAV | Anti-HAV IgG | Confirms past exposure and immunity (resulting from immunization or following an infection) |
Hepatitis B Virus | HBV | |
HBV surface antigen | HBsAg | Indicates infection (either acute or chronic) |
Hepatitis Be antigen | HBeAg | Is a derivative of HBsAg that correlates with viral replication and signifies high infectivity; if present beyond 6-8 weeks, suggests chronic carrier or disease |
Antibody to HBsAg, subclass IgM (early) and IgG | Anti-HBs | Indicates clinical recovery from HBV infection and immunity |
Total antibody to HBV core antigen | Anti-HBc | Indicates active HBV infection (either acute or chronic) |
IgM antibody to HBcAg | Anti-HBc IgM | Early index of acute HBV infection, increases during the acute phase then declines (over 4-6 months), not present with chronic HBV |
DNA of HBV | HBV DNA | Indicates HBV replication |
Hepatitis C Virus | HCV | |
Antibody to HCV | Anti-HCV | Indicates exposure to HCV |
RNA of HCV | HCV RNA | Indicates HCV infection |
Hepatitis δ (Delta) Virus | HDV | |
δ antigen | HDV Ag | Indicates HDV infection |
Total antibody to HDV | Anti-HDV | Indicates exposure to the agent (HDV) |
RNA of HDV | HDV DNA | Indicates HDV replication |
Ig, Immunoglobulin.
Adapted from Yazigi NA, Balistreri WF: Acute and chronic viral hepatitis. In Suchy FJ, Sokol RJ, Balistreri WF, editors: Liver disease in children, ed 2, Philadelphia, 2001, Lippincott Williams and Wilkins.
Management
In some affected patients, progression of liver damage occurs and cirrhosis develops; this indicates a poor prognosis for long-term survival without transplantation (see Liver Failure in this chapter and in Chapter 17, Overview of Solid Organ Transplantation).
Hepatitis A
For children with HAV, supportive care is indicated. Approximately one third of infected patients will not develop immunity, so reinfection is possible. However, those infected do not develop chronic carrier states or chronic hepatitis.64
Hepatitis B
Current treatment for chronic HBV infection includes administration of lamivudine alone or in combination with interferon.24,58 Lamivudine inhibits HBV replication and is prescribed for 1 year.58 The advantage of this therapy is its oral route of administration. Interferon reduces viral replication, so it accelerates seroconversion in those who would have naturally cleared the virus.58 Interferon is administered subcutaneously for 4 to 6 months.9 Side effects can be problematic and include flulike symptoms, weight loss, and decreased growth velocity.58 It is unclear whether interferon alone or in combination with lamivudine is the most effective therapy.
Hepatitis C
There is no vaccine available for HCV. Therapy with pegylated interferon alpha (weekly infusion) and ribavirin have been shown to be most effective in children.24 Children appear to respond better than adults to treatment, probably because children have a shorter duration of chronic disease.16
Liver Failure
Etiology
Nonalcoholic fatty liver disease has become the most common cause of liver disease in children in developed countries.34,36 This diagnosis is considered in children with mild to moderate elevation in ALT and AST levels with a body mass index in the 95th percentile or higher. The diagnosis is confirmed by liver biopsy, which reveals the accumulation of droplet fat in the hepatocytes in a child with no alcohol exposure.36 Treatment goals include identification and treatment of obesity, alleviation of risk factors such as insulin resistance, and avoidance of factors that may worsen the liver disease (e.g., exposure to hepatotoxic drugs and alcohol).36 There are no standard treatment regimens available, although lipid-lowering drugs, metformin, and vitamin E have been studied.34,36
Pathophysiology
Hepatic Encephalopathy
There are three major proposed mechanisms for development of this complication: the ammonia hypothesis, the gamma-aminobutyric acid (GABA)-benzodiazepine hypothesis and the false neurotransmitter hypothesis.15 There is evidence to support each of these theories and some successes and failures in the associated treatment modalities.
According to the GABA hypothesis, hepatic encephalopathy results from a buildup of endogenous benzodiazepines that cause neuroinhibition.15 The false neurotransmitter hypothesis is based on the observation that branch chain amino acids are increased in liver failure and aromatic amino acids are decreased in liver failure.15 In FHF, aromatic amino acids in the brain are metabolized to false neurotransmitters. These false transmitters inhibit actual neurotransmitters and disrupt message transmission in the brain.15 This neurotransmitter dysfunction appears to be secondary to hyperammonemia.
Other theories suggest that toxins such as ammonia and manganese cross the blood-brain barrier and damage nerve cells and astrocytes.8 Ammonia appears to have a direct toxic effect on the brain, and increased ammonia levels correlate with increasing grades of encephalopathy.
Renal Failure
Mechanisms of renal failure in the child with liver failure include prerenal azotemia (prerenal failure), hepatorenal syndrome, and acute tubular necrosis. Liver failure can cause decreased renal perfusion and prerenal failure as the result of the extravascular fluid shift caused by ascites, splanchnic sequestration that develops with portal hypertension, or from acute hemorrhage caused by coagulopathy. Any decrease in circulating blood volume will stimulate aldosterone secretion, causing renal retention of sodium and water (see Acute Renal Failure and Acute Kidney Injury in Chapter 13).
Clinical Signs and Symptoms
Early signs of hepatic encephalopathy include poor school performance, forgetfulness and malaise. Neurologic signs of hepatic encephalopathy include tremors, incoordination, muscle twitching, and violent movements. The classic early symptom of hepatic encephalopathy is a peculiar flapping tremor known as asterixis. It can be elicited by asking an older child to stretch the arms straight out and dorsiflex the hand. The child will be unable to maintain the hyperextended position of the hand and will develop coarse bursts of twitching movements at the wrist (see Chapter 11 for further information about encephalopathy).
A staging of hepatic encephalopathy is described in Box 14-7, and children with acute failure can progress to stage 4 (coma) in a period as short as 1 or 2 days. Progressive encephalopathy is indicated by a decreased level of consciousness. Cerebral edema can produce increased intracranial pressure (see Chapter 11 for further information regarding the recognition and management of increased intracranial pressure).
Box 14-7 Stages of Hepatic Encephalopathy
Stage 1: Mild confusion with periods of depression and euphoria, disrupted sleep
Stage 2: Moderate confusion, lethargy
EEG abnormal, with generalized slowing
Stage 3: Marked confusion, incoherent speech, sleeps but is arousable
Data from Han MK, Hyzy R: Advances in critical care management of hepatic failure and insufficiency. Crit Care Med 2006; 34(Suppl):S235-S241.EEG, Electroencephalogram.
Liver failure produces many hematologic, coagulation, and serum chemistry derangements. Both the intrinsic and extrinsic clotting cascades are affected (see Chapter 15). An ominous laboratory constellation for the child with ALF is markedly elevated liver transaminases (>1000 IU/L with a trend to normal values once few functioning hepatocytes remain to release enzymes) with a rising prothrombin time. Decreased production of serum clotting factors by the liver causes a prolonged prothrombin time, manifested by ecchymosis or petechiae and increased bleeding from puncture sites or from mucosal irritation.
Clinical signs of hepatorenal syndrome include abrupt oliguria and azotemia, often without apparent precipitating factors such as infection, sepsis, or shock. Diagnostic criteria in adults include a creatinine greater than 1.5 mg/dL (or 24-hour creatinine clearance less than 40 mL/min); absence of shock, infection, or fluid losses; no improvement in renal function after withdrawal of diuretics and expansion of intravascular volume with plasma expander (adult receives 1.5 L, child receives 20 mL/kg boluses); proteinuria less than 500 mg/day; and no evidence of parenchymal or obstructive renal disease.23 Additional laboratory findings include a normal urinalysis, hyponatremia (despite the renal retention of sodium, the serum sodium falls because there is relatively greater renal absorption of water), and hypokalemia (see the discussion of Acute Renal Failure and Acute Kidney Injury in Chapter 13).
If the cause of the hepatic failure has not been identified, diagnostic studies will be performed immediately. These studies include extensive toxicology screening (for evidence of alcohol, drug, or chemical ingestion), liver function studies, hepatitis serologies, ultrasound examination to evaluate vessels, and possible liver biopsy (Table 14-11). Although a liver biopsy can be performed in the presence of significant coagulopathy, a coagulation profile should be obtained and significant coagulopathy corrected before the biopsy. Viral, bacteriologic, and fungal blood cultures also may be ordered.
Table 14-11 Common Liver Function Tests and Changes with Liver Failure
Test | Normal Value | Interpretation |
Serum Enzymes | ||
Alkaline phosphatase | Infant, 150-420 units/L | Increases with biliary obstruction and cholestatic hepatitis |
2-10 years, 100-320 units/L | ||
γ-Glutamyltransferase | 0-3 months 0-120 units/L | Increases with biliary obstruction and cholestatic hepatitis |
3-12 months, male: 5-65 units/L | ||
3-12 months, female: 5-35 units/L | ||
1-15 years.: 0-28 units/L | ||
Aspartate aminotransferase | 5-40 units/mL | Increases with hepatocellular injury |
Alanine aminotransferase (ALT) | 5-35 units/mL | Increases with hepatocellular injury |
Lactate dehydrogenase | 10 days to 24 months: 180-430 units/L; | Isoenzyme is elevated with hypoxic and primary liver injury |
24 months to 12 years,: 110-295 units/L | ||
5′-nucleotidase | 2-11 units/mL | Increases with increase in alkaline phosphatase |
Bilirubin Metabolism | ||
Serum bilirubin | ||
Indirect (unconjugated) | <0.8 mg/dL | Increases with hemolysis (lysis of red blood cells) |
Direct (conjugated) | Neonate, <0.6 mg/dL | Increases with hepatocellular injury or obstruction |
Infants/children, <0.2 mg/dL | ||
Total | 0-1 days, <8 mg/dL | Increases with biliary obstruction |
1-2 days, <12 mg/dL | ||
3-5 days, <12-16 mg/dL | ||
Older infant, < 2 mg/dL | ||
Adult, 0.3-1.2 mg/dL | ||
Urine bilirubin | 0 | Increases with biliary obstruction |
Urine urobilinogen | 0-4 mg/24 h | Increases with hemolysis or shunting of portal blood flow |
Serum Proteins | ||
Albumin | 3.5-5.5 gm/dL | Reduced with hepatocellular injury |
Globulin | 2.5-3.5 gm/dL | Increases with hepatitis |
Total | 6-7 gm/dL | |
Albumin/Globulin (A:G) ratio | 1.5:1 to 2.5:1 | Ratio reverses with chronic hepatitis or other chronic liver disease |
Transferrin | 203-380 mcg/dL | Liver damage with decreased values, iron deficiency with increased values |
Blood Clotting Functions | ||
Prothrombin time | 11.5-14 s or 90%-100% of control | Increases with chronic liver disease (cirrhosis) or vitamin K deficiency |
Activated partial thromboplastin time (aPTT) | 30-33 seconds | Increases with severe liver disease or heparin therapy |
Data from Huether SE: Structure and function of the digestive system. In McCance KL, Huether SE, editors: Pathophysiology: the biologic basis for disease in adults and children, ed 6, St Louis, 2010, Mosby; Custer JW: Blood chemistries and body fluids. In Custer JW, Rau RE, editors: The Harriet Lane Handbook, ed 18, St Louis, 2009, Mosby-Elsevier; Values for blood clotting functions from Aquino J: Hematology. In Custer JW, Rau RE, editors: The Harriet Lane Handbook, ed 18, St Louis, 2009, Mosby-Elsevier.
Management
All children who are critically ill require planned nursing assessment and support of all body systems. The child with liver failure with multiorgan failure is no exception. Careful monitoring for respiratory cardiac, neurologic, and renal function is often more important than monitoring the child’s liver function. The focus of the following information is on clinical assessment and prevention of the complications of liver failure and encephalopathy. Figure 14-17 is an overview of care considerations for the child with end stage liver disease.
Encephalopathy
Mannitol and diuretics can be administered to treat increased intracranial pressure. Maintain the child’s serum osmolality at approximately 300 to 310 mOsm/L, and do not allow the serum sodium concentration to rise or fall acutely (see Chapter 11, Increased Intracranial Pressure).
Coagulopathies
If the child has any clinical or significant laboratory (i.e., serologic) evidence of coagulopathies, blood components are administered (see Blood and Blood Component Therapies in Chapter 15). Calculate the child’s total circulating blood volume and consider any blood loss as a percentage of this blood volume. Consider transfusion to prevent hemodynamic compromise if blood loss totals 7% to 10% of the child’s estimated blood volume.
Fluid and Electrolyte Balance
For the treatment of renal failure, the child may require hemodialysis or continuous hemofiltration. The goal should be to achieve euvolemia, and to correct electrolyte imbalances (refer to Acute Renal Failure and Acute Kidney Injury in Chapter 13).
Electrolyte and coagulation abnormalities and problems associated with liver failure are listed in Box 14-8. Electrolyte imbalance may result from fluid shifts, hyperaldosteronism, or diuretic therapy. Evaluate the child’s serum electrolyte concentrations frequently, and provide electrolyte replacement therapy as needed.
Additional Supportive Care
If the child is receiving any medications that are normally metabolized by the liver, review the doses of the medications and adjust as necessary (to prevent development of toxic drug levels). Box 14-9 details nursing interventions appropriate for care of the child with hepatic failure (note that it assumes that appropriate diagnostic studies have been performed).
Box 14-9 Nursing Care of the Child with Liver Failure
Potential for Depressed Level of Consciousness and Potential for Increased Intracranial Pressure Related to:
Alteration in Cerebral Perfusion or Alteration in Thought Processes Related to:
Nursing interventions
• Assess patient level of consciousness and neurologic function at regular intervals:
• Determine stage of hepatic encephalopathy (see Box 14-7), and report any change (particularly in arousability, speech, irritability, pupil size, and response to light) to a provider immediately.
• Monitor for early signs of encephalopathy, including tremors, lack of coordination, muscle twitching, and flapping tremors (called asterixis). These tremors may be elicited if the child is asked to outstretch the arms and dorsiflex the hand; monitor for coarse bursts of twitching at wrist.
• If the child’s level of consciousness deteriorates, monitor airway patency and airway protective mechanisms (e.g., cough, gag). Be prepared to assist with emergency intubation and begin mechanical ventilatory support.
• Once signs of increased intracranial pressure are observed, provide standard treatment (as ordered (see Chapter 11).
• Assist in diagnostic studies, including computed tomography to determine presence and severity of cerebral edema, and laboratory studies to monitor hepatic function and causes of hepatic failure.
Potential Respiratory Insufficiency as a Result of:
Expected patient outcomes
• Patient will demonstrate effective systemic arterial oxygenation and carbon dioxide elimination (ventilation).
• Respiratory rate will remain appropriate for age and clinical condition, oxygenation and carbon dioxide elimination will be adequate, and child will not demonstrate increased or inadequate respiratory effort.
Nursing interventions
• Monitor patient’s respiratory rate and effort; report any tachypnea and increased respiratory effort to provider immediately.
• If patient is breathing spontaneously, elevate the head of the bed and position the patient comfortably to maximize diaphragm excursion.
• Monitor patient level of consciousness and be prepared to assist with intubation and to provide assisted ventilation if airway protective mechanisms are compromised or respiratory insufficiency develops.
• Monitor pulse oximetry and exhaled (end-tidal) carbon dioxide or arterial blood gases to determine effectiveness of oxygenation and ventilation; notify provider of any deterioration.
• Auscultate breath sounds on a regular basis; notify provider of decreased intensity or change in pitch of breath sounds, which may indicate the development of atelectasis or hydrothorax.
• If mechanical ventilatory support is required, ensure that support is appropriate and that the child’s chest expands equally and adequately during positive pressure inspiration.
• Monitor heart rate and systemic perfusion; if a deterioration in clinical appearance is observed, evaluate systemic oxygenation and ventilatory support.
• Maintain emergency intubation (and reintubation) equipment at the bedside.
Potential Hypovolemia Related to:
Expected patient outcomes
• Patient will maintain adequate intravascular volume as indicated by effective systemic perfusion (warm extremities with brisk capillary refill, good color, strong peripheral pulses, urine output of 1 mL/kg per hour), a central venous pressure of 2-5 mmHg, and appropriate heart rate and blood pressure for age and clinical condition.
• Patient will maintain adequate hemoglobin and hematocrit, and significant acute blood loss will be replaced (per provider order).
• Patient will not demonstrate excessive weight gain associated with edema.
Nursing interventions
• Constantly evaluate systemic perfusion; notify provider of signs of inadequate systemic perfusion, including tachycardia, cool extremities, mottled color, delayed capillary refill, decreased intensity of peripheral pulses, and oliguria. Note that hypotension is usually only a late sign of hypovolemia in children.
• Monitor for signs of dehydration, including dry mucous membranes, poor skin turgor, negative fluid balance, and sunken fontanelle in infants. Notify provider if these signs are observed.
• If esophageal or gastrointestinal bleeding develops, notify provider, estimate quantity, and provide replacement blood products as ordered. Monitor serial hemoglobin and hematocrit, and notify provider of a fall in these variables.
• Calculate daily maintenance fluid requirements and record fluid intake and output. Notify provider of imbalance. Administer crystalloids and colloids as ordered to maintain intravascular volume and support systemic perfusion.
• Record patient weight daily or twice daily. Notify provider of significant weight gain or loss.
• Administer diuretics as ordered and monitor their effectiveness. These drugs are usually avoided unless increased intracranial pressure develops, because they can contribute to acute reduction in intravascular volume.
• Monitor electrolyte balance, and notify provider of signs of hemoconcentration. In general, sodium intake is limited, and potassium supplements must be administered.
• Monitor for signs of hepatorenal syndrome (oliguria and azotemia) caused by reduced renal perfusion. Notify provider of reduction in urine volume, and monitor renal function as needed (e.g., serum-urine creatinine). Low-dose dopamine therapy may be ordered to optimize renal perfusion.
Potential for Bleeding Related to:
Nursing interventions
• Observe patient closely for evidence of bleeding. Test gastric drainage and stool for evidence of blood, and notify provider of positive results.
• Insert nasogastric tube and institute gastric gravity drainage. Administer nasogastric antacids, intravenous histamine type 2 receptor blockers, and proton pump inhibitors as ordered.
• If esophageal varices are present, institute precautionary measures (e.g., prevent Valsalva maneuver, provide soft diet), and monitor the patient closely for evidence of bleeding. If bleeding develops, monitor the quantity of bleeding and be prepared to provide replacement blood therapy. (Ensure that a patient blood sample has been sent for type and cross-match analysis.) Also see Portal Hypertension elsewhere in this chapter; management of hemorrhage associated with portal hypertension includes:
• Monitor results of coagulation studies. Notify provider of coagulopathies and ensure that appropriate blood components are available before invasive procedures. Be alert for the appearance of petechiae or ecchymoses; notify provider if these are observed.
• Avoid injections and minimize venipunctures if possible. Assist with insertion of indwelling arterial line for blood sampling. It may be necessary to administer blood components before catheter insertion to ensure hemostasis during procedure.
Potential Infection and Sepsis Related to:
Nursing interventions
• Ensure that every member of the healthcare team washes their hands before and after every patient contact.
• Ensure that sterile and aseptic procedures are used for insertion and maintenance of monitoring and treatment catheters (see Box 22-6).
• Change dressings and intravenous tubing and catheters according to unit policy. Maintain occlusive dressings over central venous and intracranial pressure catheter skin entrance sites (per hospital or unit policy).
• Monitor for signs of infection, including localized signs of inflammation, fever or hypothermia, leukocytosis, or leukopenia. Notify provider of cloudy urine or drainage from wounds or catheter insertion sites. Obtain cultures as ordered or per unit policy.
• Administer antibiotics as ordered.
• If infection is present, monitor for evidence of bacteremia and development of sepsis, including a change in level of consciousness, tachycardia, respiratory alkalosis (if patient is breathing spontaneously), and thrombocytopenia. Notify provider if these signs are observed.
Potential for Poor Nutrition Related to:
Nursing interventions
• Monitor patient’s total daily intake of protein; typically, protein intake is limited to approximately 0.5-1.0 g/kg per day.
• Monitor serum albumin; notify provider of hypoalbuminemia.
• Calculate maintenance caloric requirements (see Table 14-3); determine child’s caloric intake and notify provider if caloric intake is inadequate.
• Monitor serum glucose concentration; ensure ongoing source of glucose intake and notify provider if hypoglycemia or hyperglycemia develop.
• Consult dietician to optimize the child’s nutritional status.
Liver transplantation is challenging in these extremely unstable children, particularly if multiorgan failure is present. However, transplantation affords the child with ALF the greatest chance of survival (see Chapter 17, Overview of Solid Organ Transplantation). Five-year survival for children undergoing liver transplantation for acute liver failure is relatively high (exceeding 70%).30a,31 For the most recent transplantation survival data, the reader is referred to the National Organ Procurement and Transplantation Website National Data (http://optn.transplant.hrsa.gov/latestData/viewDataReports.asp).
Pancreatitis
Etiology
Pancreatitis in children is relatively rare. Causes are diverse and can be categorized as nonobstructive, obstructive, or inflammatory. Nonobstructive causes predominate and include blunt abdominal trauma, typically caused by bicycle handle bar injuries and motor vehicle crashes. Nonobstructive pancreatitis is also associated with several medications, including didanosine, azathioprine, mercaptopurine, L-asparaginase, and valproic acid.52
Clinical Signs and Symptoms
Metabolic complications of acute pancreatitis include lipid abnormalities, hypocalcemia, hyperglycemia and metabolic acidosis.12 Hypocalcemia is observed in approximately 15% of patients with pancreatitis and develops when extensive fat necrosis occurs. Approximately 25% of patients with acute pancreatitis demonstrate hyperglycemia, because damaged pancreatic tissue releases less insulin than normal. The white blood cell count and differential are frequently normal, whereas the hematocrit reflects hemoconcentration secondary to dehydration.
Management
Because the pain associated with pancreatitis can be severe, parenteral analgesia with narcotics or nonsteroidal antiinflammatory agents is generally required (see Chapter 5). The use of morphine and other opiate derivatives is theoretically less desirable in patients with pancreatitis, because these medications increase spasm of the sphincter of Oddi and may cause additional pain.
Shock is the leading cause of death in patients with acute fulminant pancreatitis.19 Nurses must closely monitor the child’s vital signs, systemic perfusion, and evidence of fluid balance, including CVP and urine output. Fluid shifts can cause severe abdominal distension that hinders diaphragm excursion. Close monitoring of respiratory status (oxygenation, ventilation, aeration, and respiratory effort) is required.
Inflammatory Bowel Disease with Toxic Megacolon
Acute Diarrhea
Acute diarrhea is a sudden change in the frequency and consistency of stools.5 Because many GI fluids contain large amounts of sodium, chloride, hydrogen, and bicarbonate ions, any significant GI fluid loss can result in dehydration and electrolyte imbalances. The severity and type of dehydration resulting from these conditions will be influenced by the volume and content of any replacement fluids administered.
Etiology
In children, diarrhea is most often the result of a viral or bacterial organism (Table 14-12). Rotavirus is the most common inciting pathogen worldwide in children younger than 5 years.5,48 Two live oral rotavirus vaccines (Rota Teq, Merck, Sharp and Dohme, Merck Incorporated, Whitehouse, NJ; and Rotarex, GlaxoSmithKline, Research Triangle Park NC), have been approved by the Food and Drug Administration for use in the United States, and current recommendations are to immunize all children for rotavirus starting at 2 months of age.1,48
Clostridium difficile is a common cause of antibiotic-associated diarrhea. This gram-positive organism is part of the normal GI bacterial flora, but antibiotics and some other medications and conditions can disrupt normal bacterial flora and allow some organisms to grow unchecked. Hospitalized children at risk for C. difficile disease include those receiving antimicrobial agents. Additional conditions that increase risk for this organism include recent abdominal surgery, presence of a feeding tube, and decreased stomach acidity from the administration of histamine type 2 antagonists and proton pump inhibitors.50 Medications that increase GI motility (e.g., docusate [Colace], polyethylene glycol [MiraLax]) can also cause diarrhea.
Pathophysiology
Acute diarrhea alters the GI tract and can lead to dehydration. Dehydration is present when the total output of all fluids and electrolytes exceeds the total fluid intake. Dehydrated patients are deficient in both fluids and electrolytes. Dehydration is classified according to severity, based on clinical examination, and according to effects on the serum sodium and osmolality (see Dehydration and Hypovolemia in Chapter 12).
Clinical Signs and Symptoms
All children with diarrhea and dehydration will have a history of inadequate fluid intake and excessive fluid loss. The child may be febrile and usually is irritable and looks ill; there may or may not be associated vomiting. Initial clinical signs produced by dehydration can be difficult to separate from those produced by meningitis, because both can include a history of fever and irritability. However, a careful examination will reveal signs of dehydration, including sunken eyes, dry mucous membranes, and a sunken fontanelle in infants. The child will be tachycardic, and signs of compensated or hypotensive shock may be present. For further information, see Shock in Chapter 6 and Dehydration and Hypovolemia in Chapter 12.
The characteristics of the diarrhea stool can vary depending on the pathogen (see Table 14-12). If there is blood or mucous in the stool, stool studies should be obtained. The stool should be sent to identify stool pH, presence of reducing substance, presence of white blood cells, and C. difficile toxin and to check for ova and parasites and perform culture and sensitivity studies.
Management
The goals of the treatment of dehydration include restoration and maintenance of intravascular volume and systemic perfusion, and correction of abnormal serum electrolytes. If shock is present a urinary catheter is placed to enable continuous evaluation of urine production. Urine output should average approximately 0.5-1.0 mL/kg per hour. If urine output does not improve despite the presence of adequate systemic perfusion, notify an on-call provider immediately, because renal failure may be present (see Acute Renal Failure and Acute Kidney Injury in Chapter 13).
It is important to monitor the child’s response throughout therapy. If the shock is corrected and rehydration is successful, systemic perfusion, neurologic function, acid-base and electrolyte balance, and urine output should all improve (for further information, see Shock in Chapter 6 and Dehydration and Hypovolemia in Chapter 12).
Although the majority of acute diarrhea episodes are caused by viral pathogens, antimicrobial agents are administered for some bacteria or culture-proven parasitic infections. For culture-positive C. difficile infection, the causative antibiotic is discontinued if possible, and a 7-day oral course of metronidazole (Flagyl) is provided.50 Oral vancomycin can also be administered to patients who cannot tolerate metronidazole or any adolescent patients who are breastfeeding or pregnant.50
Diagnostic testing
Abdominal Plain Film
Abdominal plain films are frequently the first radiologic images obtained when abdominal pathology is suspected. Abdominal plain films are readily available, noninvasive, inexpensive, require no patient preparation, and can be obtained with portable machines in a timely manner. To review principles of interpretation of radiographs, refer to Chapter 10.
On a plain film, air appears black against a background of white and grey representing bony and soft tissue structures. This color contrast allows assessment of the distribution of air in the GI tract (see Fig. 14-15, A). Anteroposterior (AP) views are generally ordered for abdominal films, with specific positioning options such as spine, upright, and lateral decubitus. These positioning strategies are implemented to assess the distribution of air and fluid that change because of gravity. Free air rises, so when free intraperitoneal air is present it will rise to the highest location within the abdomen. With upright imaging, free intra-abdominal air will be visible just below the diaphragm. When an upright image is not possible because the patient is unstable, the lateral decubitus film is used. When the patient is placed with the left side down (left lateral decubitus), free air will appear as a dark border (air) outlining the light tissue density of the liver. Free fluid in the abdominal cavity will also be affected by gravity and will assume a dependent position.
Air fluid levels refer to a visible demarcation where air and fluid meet. When present, an air fluid level can be diagnostic of a bowel obstruction (see Fig. 14-15, B and C).
Abdominal plain films can serve as a triage tool for further evaluation of patients with an acute condition in the abdomen, especially with perforation of hollow viscera or intestinal obstruction.26
Abdominal Computed Tomography
IV contrast is iodine-based, so patients with seafood or iodine allergies must be premedicated to avoid an allergic reaction. IV contrast is cleared by the kidneys and requires adequate renal function. The nurse or other providers should verify appropriate blood urea nitrogen and serum creatinine concentration before the contrast injection. A large bore (22-gauge) IV catheter is required for administration of contrast, because power injectors are often used to ensure accurate timing between contrast administration and imaging.57
Patient movement during the study can negatively affect image quality, so sedation is recommended for children younger than 5 years or when patients are unable to cooperate with the procedure. Nothing-by-mouth status is recommended before CT imaging to minimize risks of vomiting the oral contrast. Studies have shown no increase in risk of aspiration when sedation is started after administering oral contrast.57
Exposure to ionizing radiation is a serious risk associated with CT imaging. In children, this radiation exposure can increase the risk of cancer.57 Caution and careful consideration should be taken before radiation exposure. For pediatric patients the dose of radiation can be decreased, because children have less body mass than adults. Studies limited to the specific areas of interest (i.e., pelvic CT versus full abdominal CT) will decrease radiation exposure.
CT technology continues to improve with the advent of multidetector CT scanners. This technology speeds image acquisition and will decrease artifacts associated with patient motion, such as breathing. This technology also maximizes contrast vascular enhancement. High-quality, three-dimensional images can be reconstructed, thus enhancing the diagnostic capabilities of this scanner. One of the biggest advantages for pediatric patients is a shorter scan time, so less sedation is needed.57
Abdominal Ultrasound
Ultrasound examination uses reflected sound waves to produce real-time pictures and excellent visualization (i.e., imaging) of the liver, kidneys, gallbladder, spleen, pancreas, and female pelvis.7 Different tissues (fluid, air, bone) reflect sound waves differently, so transmission and recording of reflected sound waves will produce outlines of structures on the ultrasound screen. Ultrasound with color Doppler capability can be used to evaluate blood flow characteristics and patency of blood vessels. One frequent application of this feature is to rule out the possibility of a deep vein thrombus.
1 American Academy of Pediatrics Committee on Infectious Disease. Prevention of rotavirus disease: guidelines for use of rotavirus vaccine. Pediatrics. 2007;119:171.
2 American Academy of Pediatrics Subcommittee on Hyperbilirubinemia. Management of hyperbilirubinemia in the newborn infant 35 or more weeks of gestation. Pediatrics. 2004;114:297.
3 American Academy of Pediatrics Subcommittee on Hyperbilirubinemia. Neonatal jaundice and kernicterus. Pediatrics. 2001;108:763.
4 Arensman R.M., Browne M., Madonna M.B. Gastrointestinal bleeding. In Grosfeld J.L., et al, editors: Pediatric surgery, ed 6, Philadelphia: Mosby, 2006.
5 Baker R.A.U., Mondozzi M.A., Hockenberry M.J. Conditions that produce fluid and electrolyte imbalance. In Hockenberry M.J., Wilson D., editors: Wong’s nursing care of infants and children, ed 8, St Louis: Mosby-Elsevier, 2007.
6 Bell M., et al. Neonatal necrotizing enterocolitis. Therapeutic decisions based upon clinical staging. Ann Surg. 1978;187:1-7.
7 Bloom D.A., Siegel M.J. Acute abdominal pain in childhood. In Bluth E.I., et al, editors: Ultrasound: a practical approach to clinical problems, ed 2, New York: Thieme Medical Publishers, 2008.
8 Butterworth R.F. Hepatic encephalopathy. Alcohol Res Health. 2003;27:240.
9 Chang M. Hepatitis B virus infection. Semin Fetal Neonatal Med. 2007;12:160.
10 Cohran V.C., Kocoshis S.A. Short bowel. In Baker S., Baker R., Davis A., editors: Pediatric nutrition support, ed 1, Sudbury, MA: Jones and Bartlett, 2007.
11 Colletti J.E., et al. An emergency medicine approach to neonatal hyperbilirubinema. Emerg Med Clin N Am. 2007;25:1117.
12 Czako L., et al. Interactions between endocrine and exocrine pancreas and their clinical relevance. Pancreatology. 2009;9:351.
13 Dasgupta R., et al. Effectiveness of REX shunt in the treatment of portal hypertension. J Ped Surg. 2006;41:108.
14 Deerojanawong J., et al. Incidence and risk factors of upper gastrointestinal bleeding in mechanically ventilated children. Pediatr Crit Care Med. 2009;10:91.
15 Faint V. The pathophysiology of hepatic encephalopathy. Nurs Crit Care. 2006;11:69.
16 Fischler B. Hepatitis C virus infection. Semin Fetal Neonatal Med. 2007;12:168.
17 Fishbein T.M., Matsumoto CS. Intestinal replacement therapy: timing and indications for referral of patients to an intestinal rehabilitation and transplant program. Gastroenterology. 2006;130:S147-S151.
18 Flanigan L.M. Biliary atresia and choledochal cyst. In Brown N.T., et al, editors: Nursing care of the pediatric surgical patient, ed 2, Sudbury, MA: Jones and Bartlett, 2007.
19 Gavaghan M. The pancreas-hermit of the abdomen. AORN J. 2002;75:1109.
20 Giannone P.J., et al. Necrotizing enterocolitis in neonates with congenital heart disease. Life Sci. 2008;82:341.
21 Gordon P.V., et al. Emerging trends in acquired neonatal intestinal disease: is it time to abandon Bell’s criteria? J Perinatol. 2007;27:661.
22 Goulet O., et al. Irreversible intestinal failure. J Pediatr Gastroenterol Nutr. 2004;38:250.
23 Han M.K., Hyzy R. Advances in critical care management of hepatic failure and insufficiency. Crit Care Med. 2006;34:S225.
24 Heller S., Valencia-Mayoral P. Treatment of viral hepatitis in children. Arch Med Res. 2002;38:702.
25 Henry M.C.W., Moss L.R. Current issues in the management of necrotizing enterocolitis. Semin Perinatol. 2004;28:221.
26 Hernanz-Schulman M., Spottswood S. Gastrointestinal tract. In Slovis T.L., editor: Caffey’s pediatric diagnostic imaging, ed 2, Philadelphia: Mosby, 2008.
27 Huether S.E. Structure and function of the digestive system. In McCance K., Huether S.E., editors: Pathophysiology: the basis for disease in adults and children, ed 5, St Louis: Elsevier, 2006.
28 Ip S., et al. An evidence-based review of important issues concerning neonatal hyperbilirubinemia. Pediatrics. 2004;114:e130.
29 Iyer K.R., Richard M. Surgery for intestinal failure. In: Matrese L.E., Steiger E., Seidner D.L., editors. Intestinal failure and rehabilitation: a clinical guide. Boca Raton, FL: CRC Press, 2005.
30 Joffe A., et al: Nutritional support for critically ill children (review). Cochrane Database Syst Rev. 2009:(2):CD005144 Apr 15
30a Kamath B.M., Olthoff K.M. Liver transplantation in children: update 2010. Pediatr Clin North Am. 2010;57(2):401-414.
31 Kelly D.A. Managing acute liver failure. Current Paediatr. 2001;11:96-101.
32 Khan A.N., Macdonald S., Howat J. Small-bowel obstruction, 2009. Available at http:emedicine.medscape.com/article/374962, 2011. Accessed October 3, Updated July 22, 2001
33 Kyle U.G., et al. Organ dysfunction is associated with hyperglycemia in critically ill children. Intens Care Med. 2010;36:312-320.
34 Lee C.K., Jonas M.M. Pediatric hepatobiliary disease. Curr Opin Gastroenterol. 2007;23:306.
35 Leonis M.A., Balistreri W.F. Evaluation and management of end-stage liver disease in children. Gastroenterology. 2008;143:1741.
36 Lerret S.M., Skelton J.A. Pediatric nonalcoholic fatty liver disease. Gastroenterol Nurs. 2008;31:115.
37 Lin P.W., Stoll B.J. Necrotising enterocolitis. Lancet. 2006;368:1271.
38 Lopez-Herce J. Gastrointestinal complications in critically ill patient: what differs between adults and children? Curr Opin Clin Nutr Metab Care. 2009;12:180.
39 Louie J.P. Essential diagnosis of abdominal emergencies in the first year of life. Emerg Med Clin N Am. 2007;25:1009.
40 Mack C.L., Sokol R.J. Unraveling the pathogenesis and etiology of biliary atresia. Pediatr Rev. 2005;57:87R.
41 Maisels M.J. A primer on phototherapy for the jaundiced infant. Contemp Pediatr. 2005;22:38.
42 Maisels M.J., McDonagh A.F. Phototherapy for neonatal jaundice. N Eng J Med. 2008;358:920.
43 Martin S.A., Simone S. Gastrointestinal system. In Slota M.C., editor: Core curriculum for pediatric critical care nursing, ed 2, St Louis: Elsevier, 2006.
44 Mehta N.M., Compher C., A.S.P.E.N. Board of Directors. A.S.P.E.N. clinical guidelines: nutrition support of the critically ill child. JPEN J Parenter Enteral Nutr. 2009;33:260.
45 Metheny N.A. Residual volume measurement should be retained in enteral feeding protocols. Am J Crit Care. 2008;17:62.
46 Moyer M.S., Warner B. Surgical disorders. In Kleinman R.E., et al, editors: Walker’s pediatric gastrointestinal disease: physiology, diagnosis and management, ed 5, Ontario, Canada: B.C. Decker, Incorporated, Hamilton, 2008.
47 Nithiwathanapong C., Reungrongrat S., Ukarapol N. Prevalence and risk factors of stress induced gastrointestinal bleeding in critically ill children. World J Gastrol. 2005;11:6839.
48 Parez N. Rotavirus gastroenteritis: why to back up the development of new vaccines? Comp Immun Microbiol Infec Dis. 2008;31:253.
49 Patole S. Prevention and treatment of necrotizing enterocolitis in preterm neonates. Early Hum Devel. 2007;83:635.
50 Pelleschi M.E. Clostridium difficile-associated disease diagnosis, prevention, treatment, and nursing care. Crit Care Nurs. 2008;28:27.
51 Pierro A., Hall N.J., Chowdhury M.M. Gastrointestinal surgery in the neonate. Curr Paedeatr. 2006;16:153.
52 Pietzak T.T., Thomas D.W. Pancreatitis in childhood. Pediatr Rev. 2000;21:406.
53 Quiros-Tejeira R.E., et al. Long-term parenteral nutritional support and intestinal adaptation in children with short bowel syndrome: a 25-year experience. J Pediatr. 2004;145:157.
54 Reyes J.D. Intestinal transplantation. Semin Pediart Surg. 2006;15:228.
55 Ryckman F.C., Alonso M.H. Causes and management of portal hypertension in the pediatric population. Clin Liver Dis. 2001;5:789.
56 Sehgal S., Allen P.L.J. Hepatitis C in children. Pediatr Nurs. 2004;20:409.
57 Siegel M.J. Practical CT techniques. In Siegel M.J., editor: Pediatric body CT, ed 2, Philadelphia: Lippincott, 2008.
58 Sims R.J.M., Woodgate R.L. Managing chronic hepatitis B in children. J Pediatr Health Care. 2008;22:360.
59 Slowik M.K., Jhaveri R. Hepatitis B and C viruses in infants and young children. Semin Pediatr Infect Dis. 2005;16:296.
60 Srinivasan P.S., Brandler M.D., D’Souza A. Necrotizing enterocolitis. Clin Perinatol. 2008;35:251.
61 Superina R. Portal hypertension. In Grosfeld J.L., et al, editors: Pediatric surgery, ed 6, Philadelphia: Mosby, 2006.
62 Teitelbaum D.H., Coran A.G. Nutritional support. In Grosfeld J.L., et al, editors: Pediatric surgery, ed 6, Philadelphia: Mosby, 2006.
63 Wennberg R.P., et al. Toward understanding kernicterus: a challenge to improve the management of jaundiced newborns. Pediatrics. 2006;117:474.
64 Yazigi N.A., Balistreri W.F. Acute and chronic viral hepatitis. In Suchy F.J., Sokol R.J., Balistreri W.F., editors: Liver disease in children, ed 2, Philadelphia: Lippincott Williams and Wilkins, 2001.