CHAPTER 114 Frame and Frameless Stereotactic Brain Biopsy
Historical Landmarks
Pioneering neurosurgeons in the end of the 19th century and at the dawn of the 20th century, working without the benefit of imaging modalities, relied exclusively on anatomic knowledge and clinical findings to strategize their operative approaches. The first person to use a device for localizing brain structures was D. N. Zernov in 1889. A professor of anatomy in Moscow, Russia, he invented an apparatus that could be fastened to the head and adjusted to localize points that could then be identified on a map that showed the average positions of cranial structures.1 Zernov first used this device to identify and drain an abscess in the interparietal sulcus. Although his work was continued by others in Russia, his method did not become well known worldwide.2 Without being aware of Zernov’s method, Victor Horsley and Robert Clarke described a “steretotactic” method of localizing brain structures in animals with a frame based on a three-dimensional coordinate system in 1906 (Greek stereo meaning three-dimensional; taxis meaning to move toward).3 However, because subcortical structures could not be reliably localized, stereotaxy in humans was not widely adopted until intracranial imaging was available.2
It was not until 1947 that the first human stereotactic system, termed the encephalotome, was demonstrated by Spiegel and Wycis.4 By this time, x-ray technology combined with the development of ventriculography, a method whereby air or contrast material is introduced into the ventricles to facilitate clearer imaging on x-ray plates, made it practical to take films in the operating room and develop them in a timely manner.5 The underlying principle is to target an instrument toward a particular neural structure based on information regarding its location obtained on the preoperative images. Successful completion of this task required that the instrument involved be guided stably toward the target in a coordinate system common to both the preoperative images and the in vivo structures. Before image acquisition, a frame was firmly attached to the patient’s skull to produce images with markers that are referenced to a frame-based coordinate system.2 By using the same coordinate system during the operation, surgical instruments could be aimed toward the target structure shown on the images.
The pioneering development of the encephalotome led to development of other stereotactic frames, and with the introduction of CT in 19736,7 and MRI in 1980,8 frame-based stereotactic guidance took a firm place in the conduct of neurosurgical operations.2,5 These frame-based systems were initially used to generate lesions in different neural structures for treating movement disorders, pain, and epilepsy and then evolved for performing drainage of abscesses and biopsy of tumors.2,9 Although frame-based systems have proved to be reliable and accurate targeting devices,10,11 they have several disadvantages that limit their utility2: (1) attachment of the frame to the patient’s skull often needs to be done on the same day as the operation, especially when frame application requires general anesthesia, (2) posterior fossa lesions are difficult to target, (3) the frame can limit access to the surgical field, (4) guidance is limited to a single target or only a few targets, and (5) maneuvering the apparatus during surgery can be unwieldy.2 For these reasons, frameless stereotaxy has been developed to improve the efficacy and accuracy of targeting systems.
Background
Frame-Based Stereotaxy
The Leksell frame (Fig. 114-1), introduced in 1949, is based on the arc-radius principle and is one of the more commonly used frame-based systems in modern neurosurgery. A frame is rigidly attached to the patient’s head to which the semicircular arc is mounted by pivoting shafts that in turn are connected to the graduated bars of the frame. Ultimately, the arc can be manipulated for vertical and anterior-posterior adjustments to permit differential targeting by moving the center of the arc.
The frame consists of four graduated bars that define the corners of a cube; on the left/right sides of the frame (in relation to the patient) there is a diagonal bar on each side connecting the corner of these posts to create an “N.” Once the patient is scanned (CT or MRI), the geometric center in the anterior-posterior plane is defined by looking at an axial image and drawing an “X” by connecting the corners of the frame. The center in the vertical plane is assessed by a line connecting the midpoint of the corner posts going through the “N.” After the center is defined, the location of the target in relation to the geometric center can be calculated and the semicircular arc manipulated (Fig. 114-2) accordingly to place that lesion at the center of the arc.
Frameless Stereotaxy
Principles of Neuronavigation
For both frame-based and frameless systems (Fig. 114-3), the relationship between the coordinate space for the preoperative images and that for the surgical field must be established. In frame-based systems, by using the same frame for both preoperative image acquisition and performing the surgery, the relationship between the two coordinate systems is known and no further transformation is required. For frameless systems, point-pair registration or surface contour registration can be used to establish the relationship between the preoperative images and that for the surgical field.
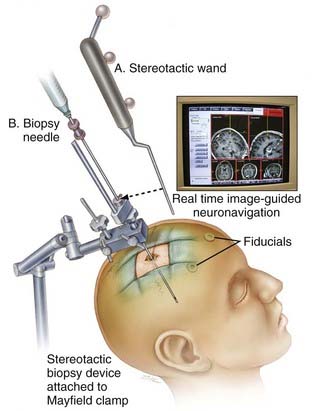
FIGURE 114-3 Image depicting a frameless stereotactic biopsy system that relies on neuronavigation systems for lesion targeting.
(From Woodworth GF, McGirt MJ, Samdani A, et al. Frameless image-guided stereotactic brain biopsy procedure: diagnostic yield, surgical morbidity, and comparison with the frame-based technique. J Neurosurg. 2006;104:233-237.)
Point-Pair Registration
In frameless systems, the relationship between the two different coordinate systems, one related to the image and the other related to the patient during surgery, requires a patient-to-image registration procedure to create a mutual relationship.2 Point-pair matching was the first and has been the most common method to achieve transformation between the two coordinate systems.12,13 This method requires a set of at least three non-colinear points to be defined in the coordinates of the images. These points are known as fiducial points and can consist of either natural anatomic landmarks (i.e., nasion, lateral canthus, or tragus), skin-applied markers, or bone-implanted markers.2 These same fiducial points are defined again within the coordinate system of the surgical field in the operating room. Software is then used to establish the relationship between the coordinates of the fiducial points in the image space and their counterpart in the surgical space.2
Surface Contour Registration
In a comparison of natural landmarks and skin-applied fiducial markers, navigation based on registration of natural landmarks alone has generally been slightly less accurate, in large part because they consist of less discretely defined points. Golfinos and coauthors reported that anatomic landmark and surface-fit algorithms have an accuracy of half that achieved with fiducial-based registration.14 To this day, the sophistication of surface registration strategies continues to evolve. Although surface matching can be used as an alternative to fiducial mapping, its use in combination with fiducial point-pair matching may be an optimal strategy to further improve reliability in stereotactic localization for biopsy.
Once the spatial relationship is established with either point-pair registration or surface contour registration, it is used throughout the remainder of the operation to map anatomic targets of the patient to that of the preoperative images. This thus requires that the patient’s head be immobilized with a head clamp and the operating table not be moved during surgery. To overcome the error that may be induced with patient or operating table movement, tracking units attached to the patient’s head15 or a surgical head clamp16 can be used. These sets of reference markers, termed dynamic reference frames, can be used to correct for any unwanted movement. Markers attached to a pointing device or, alternatively, a microscope can be tracked to locate position and orient the axis given these fixed dynamic reference frames.
Indications for Stereotactic Biopsy
The significant development of intracranial imaging over the past few decades has allowed much earlier diagnosis of brain tumors. Although some tumors have a characteristic appearance on imaging, no imaging modality is yet able to provide sufficient diagnostic information to direct subsequent therapy. As a result, tissue biopsy remains indispensible in many cases. The goal of biopsy is to provide a representative sample for pathologic diagnosis to guide subsequent treatment, which can include cytoreductive surgery, radiotherapy, or chemotherapy.17
The main stimulus for the adoption of stereotactic biopsy over an open operative procedure is to achieve a higher rate of diagnostic accuracy while minimizing potential adverse effects. Diagnostic accuracy is important for dictating appropriate adjuvant therapy. Particular characteristics of the tumor that favor the use of stereotactic biopsy over open biopsy include (1) lesions that do not exert a symptomatic mass effect or that are not curable by surgical excision, such as metastases or malignant intrinsic brain tumors (Fig. 114-4); (2) deep-seated lesions or those occupying space in eloquent cortex or deep nuclei (i.e., basal ganglia, thalamus), where open resection would lead to unacceptable morbidity/mortality; and (3) infiltrative lesions (i.e., gliomatosis cerebri) that do not have a clear brain-tumor margin and are unlikely to be excised completely without significant loss of normal brain parenchyma.17 Moreover, if the lesion’s appearance on imaging or the course of the disease suggests an alternative cause such as an infectious or demyelinating process rather than a neoplastic one, stereotactic biopsy is a more appropriate first step than a large open procedure.17
Nevertheless, although stereotactic biopsy is a relatively safe procedure, there are relative contraindications. For example, vascular tumors such as metastatic renal cell carcinoma, choriocarcinoma, or metastatic melanoma should not be approached stereotactically, if possible, because of their inherently higher risk for hemorrhage.17 In such cases of metastatic tumor, a stronger effort to biopsy and diagnose the primary neoplasm instead is generally recommended. Furthermore, location of the tumor close to a major blood vessel, the vessel-rich sylvian fissure, the cavernous sinus, or the brain-pial border should also alert the neurosurgeon to the more elevated risk for hemorrhage.