14. Fluid and Electrolyte Management*
James E. Jones, Ray D. Hayes, Alisa L. Starbuck and Peter J. Porcelli
Advances in the management of specific neonatal disorders have contributed to a remarkable decline in morbidity and mortality in newborns. Fluid and electrolyte therapy, thermal regulation, and maintenance of oxygenation remain central features of modern, supportive neonatal intensive care. Thus infants requiring tertiary care (and most infants requiring intermediate level II or secondary care) will initially receive parenteral fluid and electrolytes. Fluid and nutrition data have been accumulated for full-term infants, but some crucial information is still missing regarding very-low-birth-weight (VLBW) infants (infants with birth weights <1500 g). For example, it is clear that the restrictive fluid policies of the 1950s aimed at reducing the observed postnatal diuresis were misguided efforts that caused hyperosmolality, hyperbilirubinemia, and hypoglycemia. On the other hand, the degree to which initial fluid, electrolyte, and glucose administration should be “liberalized” remains uncertain, 1,3,4 largely because patent ductus arteriosus, necrotizing enterocolitis, bronchopulmonary dysplasia (BPD), intraventricular hemorrhage (IVH), and hyperglycemia in VLBW infants are associated with larger volumes of fluid, electrolyte, and glucose administration. 1 At best, clinicians make approximations for therapy in many clinical situations, which is why good measures of fluid and electrolyte requirements are needed. In addition, the fluid requirements of VLBW infants may be modified by prenatal steroid administration to mothers and the use of artificial surfactant in infants.
This chapter discusses implementation of the following fundamental principles: (1) rapidly assessing the infant’s initial condition; (2) developing a short-term, time-oriented management plan; (3) initiating therapy; and (4) monitoring the infant and modifying the plan based on clinical and biochemical data.
PHYSIOLOGY
Neonates show significant physiologic differences when compared (on a per-kilogram basis) with older children and adults: (1) their basic metabolic rate is greater, even double; (2) their fluid requirements are four to five times higher; (3) their sodium excretion is only 10% of that in older children and adults; and (4) their glomerular filtration rate is 5 to 10 times less than that of adults. 3 The subdivisions of total body mass (TBM) are illustrated in Figure 14-1. Total body water (TBW) as a percentage of TBM demonstrates a curvilinear decline with increasing gestational age (Figure 14-2). During the early fetal period, the fetus’s TBW is 95% of total weight and decreases to 80% at 8 months gestation and then to 75% at term. 3 Intracellular fluid (ICF) and extracellular fluid (ECF) as percentages of TBM change in opposite directions as gestational age advances, whereas ECF decreases as ICF increases with growth. 2
![]() |
FIGURE 14-1
(From Winters RW, editor: The body fluids in pediatrics, Boston, 1973, Little, Brown.)
|
![]() |
FIGURE 14-2
(From Winters RW, editor: The body fluids in pediatrics, Boston, 1973, Little, Brown.)
|
These physiologic and body composition phenomena result in a narrow margin of safety when calculating fluids and electrolytes for small infants, especially those less than 1250 g. Caregivers should independently calculate all requirements and compare calculations with standard guidelines. Intravenous (IV) fluid should be administered by a special infusion pump that can regulate fluid with precision of at least 0.1 mL/hr. Intake should be measured hourly and all output measured. The balance of intake versus output should be assessed at least every 8 to 12 hours using a standard form (Figure 14-3). Once clinical signs of fluid overload or deficit occur, it may be difficult to regain balance. Fluid balance should be managed prospectively with consistent procedures as a part of every initial care plan.
![]() |
![]() |
FIGURE 14-3
(Courtesy Brenner Children’s Hospital, Winston-Salem, North Carolina.)
|
The effect of gestational age on body composition is striking (Figure 14-4). Because gestational age is a significant determinant of the percentage and distribution of TBW, accurate assessment is important. Changes in distribution and percent of body water will be influenced by intrauterine growth, maternal fluid balance, postnatal age, postnatal diet, daily water intake, and changing fluid and electrolyte absorption and excretion.
![]() |
FIGURE 14-4
(From Heird WC, Driscoll JM Jr, Schullinger JN, et al: Intravenous alimentation in pediatric patients, J Pediatr 80:351, 1972.)
|
The initial (first 1 to 3 days) weight loss of both healthy term (up to 5% to 10% of TBM) and preterm (up to 10% to 15% of TBM) infants should be considered a normal physiologic loss of fluid from the interstitial fluid (ISF), rather than a pathophysiologic catabolism of body tissues. After birth, contraction of the ECF compartment occurs, followed by natriuresis, diuresis, and weight loss. 2,3 This weight loss is then regained over 7 to 10 days as muscle and fat. Preterm neonates often demonstrate relative oliguria during the first 24 to 48 hours. Neonates with respiratory distress syndrome (RDS) will have delayed postnatal contraction of the ECF compartment, further delaying diuresis. Onset of the diuresis after several days old usually coincides with the initial stages of recovery from RDS. 5
Despite the period of natriuresis after birth, infants usually require no additional sodium during the first 24 to 48 hours of life. This strategy can promote decreased oxygen requirement and possibly decreased incidence of chronic lung disease. 2 It is normal to have an initial negative sodium balance, but later it is necessary to retain sodium and often additional sodium supplementation is required for appropriate growth. 5
Reviewing the maternal history and the intrapartum course may be helpful to calculate the infant’s fluid and electrolyte requirements. For example, if the mother received large amounts of electrolyte-free fluids in the intrapartum period, the neonate may be hyponatremic and have an expanded ECF space at birth. Because small-for-gestational-age (SGA) infants have reduced amounts of fat, body water (as a percentage of TBM) increases. Conversely, large-for-gestational-age (LGA) infants with an increased amount of body fat have a lower percentage of TBW.
ECF comprises both intravascular fluid (plasma) and ISF. The electrolyte composition of ISF and plasma is similar, but it is strikingly different from ICF (Figure 14-5). Sodium is the major cation in ECF (both ISF and plasma) and is easily measured. Potassium, the major cation in ICF, on the other hand, cannot be measured readily because ICF is not easily accessible. Because 90% of the total body potassium is intracellular, low levels of plasma potassium are assumed to reflect low total body potassium.
![]() |
FIGURE 14-5
(From Winters RW, editor: The body fluids in pediatrics. Boston, 1973, Little, Brown.)
|
Osmotic force or pressure is a property of solutions. Osmotic phenomena depend on the number (N) of particles in a solution regardless of size or charge and are measured in milliosmoles (mOsm) according to this equation:

where mOsm represents the osmolality or osmolarity of the solution, mM represents the concentration of the measured particles in moles per liter and N represents the number of particles in the solution for each molecule after the substance is dissolved. For example, each molecule of NaCl when dissolved in water dissociates into two ions, each of which is osmotically active. N for NaCl is therefore 2.
Table 14-1 shows three examples. Unfortunately, two physical chemistry terms are used interchangeably in clinical medicine: (1) osmolality (milliosmole per kilogram of water) and (2) osmolarity (milliosmole per liter of solution). In most laboratories, osmotic forces are determined by the technique of freezing point depression, so osmolality is the correct term (normally 280 to 300 mOsm/kg water). The difference in terms is usually unimportant, because the total solid content per liter of plasma is small.
mM | N | mOsm | |
---|---|---|---|
NaCl | 1 | 2 | 2 |
Glucose | 1 | 1 | 1 |
CaCl2 | 1 | 3 | 3 |
Osmolality can be satisfactorily estimated in many clinical settings by the following formula (Figure 14-6):
![]() |
FIGURE 14-6
(From Winters RW, editor: The body fluids in pediatrics, Boston, 1973, Little, Brown.)
|

The coefficients 1/18 and 1/2.8 for the glucose and blood urea nitrogen (BUN) terms are present to convert milligrams per deciliter values to millimolar concentrations. For example, a patient with a serum sodium concentration of 135 mEq/L, a glucose of 72 mg/dL, and a BUN of 28 mg/dL has a calculated osmolality of:

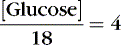
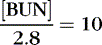

Osmotic forces are responsible for apparently low plasma electrolyte concentrations in some common clinical settings. For example, in hyperglycemia, the plasma sodium concentration reported by the laboratory is usually low but the total effective osmolality may be normal, as seen in this example:





An analogous situation exists for the less frequent condition, hyperlipidemia, in which low laboratory plasma sodium values are reported with a normal osmolality (Figure 14-7). Low laboratory values for plasma sodium occur because the increase in plasma solids (lipids) causes a lower plasma water content because of water displacement and hence a lower sodium concentration per liter of whole plasma. In this case, the plasma water and sodium concentration may be normal.
![]() |
FIGURE 14-7
(From Winters RW, editor: The body fluids in pediatrics, Boston, 1973, Little, Brown.)
|
Osmotic forces largely determine shifts in the internal redistribution of water in hydration disturbances. Four pure disturbances of hydration exist: (1) too much electrolyte; (2) too little electrolyte; (3) too much water; and (4) too little water. Combinations of these disturbances often occur. Another example of changes in osmolality occurs in preterm infants who undergo insensible water loss because of skin immaturity, decreased body fat, and a large surface-to-volume ratio leading to increased evaporation. This water loss from the interstitial space results in a hyperosmolar extracellular compartment exhibited by hypernatremia and occasionally hyperkalemia and hyperglycemia. 2,3
Neonatal renal “immaturity” affects fluid balance and electrolyte needs. Renal functions do not develop at the same rate. Neonatal glomerular filtration rate (GFR) is low in utero but increases rapidly within a few hours after delivery because renal blood flow increases as a result of the increasing mean arterial blood pressure and increasing glomerular permeability. 5 Glomerular filtration rate is a measure of renal function, which assesses how infants vary their fluid and electrolyte excretion and reabsorption according to their input and losses.
GFR is independent of gestational age. It rises rapidly during the first 6 weeks of life, gradually increasing more slowly during infancy, and reaches adult values by 12 months of age. A very-low-birth-weight (VLBW) infant in satisfactory condition at 6 weeks should have adequate GFR. These observations are based on the infant developing a full complement of nephrons (about 34 weeks’ gestation) and the continued increase in glomerular surface area (beyond 40 weeks’ gestation). 3,4 Glomerular filtration rate can be compromised in critically ill neonates by a patent ductus arteriosus and mechanical ventilation. Extubation can improve GFR by up to 15%. 5
Renal tubular function is responsible for mineral and electrolyte excretion and reabsorption. Renal tubular function is influenced by gestational age. 5 Therefore urine sodium losses are a function of gestational age and sodium intake. Preterm infants with immature tubular function have a limited ability to excrete sodium. Urine sodium excretion increases slowly during the first 2 years of life. When increased amounts of sodium are provided to more mature infants, there will be a renal response resulting in elevated urine sodium as the renal system attempts to normalize serum sodium.
The capacity to change urine concentration in VLBW infants appears limited but can be influenced by gestational age and nutrient intake. Immature concentrating ability (maximum of approximately 600 mOsm/L) (Figure 14-8) coupled with an inability to excrete (rapidly) an acute water or sodium load results in a narrow margin of safety when prescribing fluid and electrolytes, especially in VLBW infants. 1,3,4
![]() |
FIGURE 14-8
(From Jones MD, Gresham EL, Battaglia FC: Urinary flow rate and urea excretion rates in newborn infants, Biol Neonate 21:322, 1972.)
|
Urea is the major component of urine osmolality (and hence specific gravity), whereas electrolytes quantitatively contribute less. When total parenteral nutrition is provided, urine specific gravity may rise because of the low renal threshold for glucose and amino acids. When specific gravity rises, the cause should be identified before adjusting the fluid infusion rate. A diagnostic test (Multistix 10 SC or Chemstrip) can screen for glucose and protein but not for amino acids, which must be detected by amino acid chromatography when necessary.
Neonatal urinary acidification is limited, and the threshold for bicarbonate excretion is reduced. Both physiologic and pathologic factors can contribute to alkaline urine. VLBW infants have a limited capacity for hydrogen ion excretion, whereas other infants may have acute illnesses such as bicarbonate-losing tubular necrosis or urinary tract infection.
The roles of hormones including antidiuretic hormone, aldosterone, atrial natriuretic factor, and parathormone to regulate neonatal fluid and electrolyte balance are not well defined. Hormonal influences can be primary, such as in the syndrome of inappropriate secretion of antidiuretic hormone and in some cases of hypocalcemia. Secondary hormonal influences can be caused by certain drugs, such as the aldosterone antagonist spironolactone.
Insensible water loss (IWL) occurs via pulmonary and cutaneous routes and is influenced by the factors listed in Table 14-2. However, IWL varies greatly depending on gestational age and birth weight (Table 14-3). The neonate’s environment significantly affects fluid balance. Radiant warmer usage decreases the neonate’s radiant heat loss but can increase IWL by 50% to 200%, resulting in hypernatremic dehydration. 6 Incubators reduce radiant heat loss via their double-walled Plexiglas design.
Decrease IWL | Increase IWL |
---|---|
Heat shield or double-walled incubators
Plastic blankets
Clothes
High relative humidity (ambient ventilator gas)
Emollient use
|
Inversely related to gestational age and weight
Respiratory distress
Ambient temperature above thermoneutral
Fever
Radiant warmer
Phototherapy
Activity
|
Increment for phototherapy: 20-30 mL/kg/day if patient is in open warmer and has radiant phototherapy. No adjustment if baby is in humidified environment and/or has fiberoptic phototherapy source. | |||||
Increment for radiant warmer: 20-30 mL/kg/day. | |||||
Maintenance solutes: Glucose: 7-12 g/kg/day (4-8 g/kg in VLBW infants) | |||||
Na: 1-4 mEq/kg/day (2-8 mEq/kg/day in VLBW infants) K: 1-4 mEq/kg/day Cl: 1-4 mEq/kg/day Ca: 1 mEq/kg/day | |||||
IWL, Insensible water loss; VLBW, very-low-birth-weight. | |||||
*Adjustment based on a urine flow rate of 2 to 5 mL/kg/hr and a stable weight. |
|||||
†May be reduced by 30% if the infant is on a ventilator. |
|||||
Weight (g) | Ranges of Water Loss | Day 1* | Days 2-3* | Days 4-7* | |
---|---|---|---|---|---|
Less than 1250 |
40-170
50-100
5-10
|
||||
TOTAL | 95-280 | 120 | 140 | 150-175 | |
1250-1750 |
20-50
50-100
5-10
|
||||
TOTAL | 75-160 | 90 | 110 | 130-140 | |
More than 1750 |
15-40
50-100
5-10
|
||||
TOTAL | 70-150 | 80 | 90 | 100-200 |
Buy Membership for Neonatal and Perinatal Medicine Category to continue reading. Learn more here