Chapter 21 Femoral Tunnel Placement to Restore Normal Knee Laxity After Anterior Cruciate Ligament Reconstruction
Introduction
In order to perform a successful anterior cruciate ligament (ACL) reconstruction, the surgeon must make a number of steps that require correct judgment and execution, but there is evidence that the most frequent cause of failure is malpositioning of the graft tunnel in the femur.1 This is not surprising because of the anatomy of the interior of the knee joint and the difficulty of seeing the femoral attachment of the ACL. Because the surgeon views the interior of the intercondylar notch when the knee is flexed, the ACL attachment is carried back into the furthest recess of the knee. This means that there is plenty of scope to err with the tunnel placement if the ACL attachment is not visualized clearly. In particular, the undulating surface of the femoral intercondylar notch includes a transverse ridge or bulge that should come between the observer and the proximal part of the ACL attachment; the inexperienced surgeon may believe that this ridge is the posterior outlet of the notch and then place the graft tunnel shallow to that ridge in a nonanatomical position. The frequency of this error has led to common usage of the term “resident’s ridge” to describe this anatomical feature.
The aim of this chapter is to describe the evolution of knowledge regarding ACL graft placement on the femur, which relates closely to our understanding of the function of the ACL itself. There has been a recent move toward “anatomical” reconstructions, with two grafts in parallel, attempting to reproduce two functional fiber bundles of the ACL. This has prompted a better appreciation of the natural ACL attachment anatomy when performing a conventional single-bundle reconstruction. The tibial attachment is not considered here because changes of the femoral attachment have a much larger effect on ACL graft tension and length changes.2
In this chapter, two distinct sets of terminology will be used to describe femoral graft tunnel positions: (1) anatomical nomenclature for describing positions when the knee is in extension (anterior-posterior, proximal-distal) and (2) surgical nomenclature for describing what the surgeon views when the knee is flexed approximately 90 degrees (high-low, deep-shallow, respectively).3
Functional Anatomy of the Anterior Cruciate Ligament Related to Graft Tunnels
The ACL has a complex fiber structure composed of many fascicles bound together within a synovial covering layer. The fibers are not arranged simply in parallel, and this gives rise to the cross-sectional area being less at the mid-length than at the bony attachments: the fibers must splay out toward the bones.4 The functional significance of this architecture is not understood. However, at a gross level, the fibers of the ACL are arranged as a flat band, and all are tensed when the knee is extended (Fig. 21-1, A). This fiber band is oriented in a sagittal plane so that the ACL fits into and fills the narrow slot between the posterior cruciate ligament (PCL), which occupies most of the width of the intercondylar notch, and the lateral femoral condyle. The sagittal plane of the ACL orientation means that it attaches to the tibia over an area that is oriented anteroposterior (AP). The ACL attaches to the femur over an area that is oriented from anteroproximal to posterodistal.5 This femoral attachment is close to and bounded posteriorly by the condylar articular cartilage and has an overall alignment approximately 35 degrees posterior-distal to the axial.6
When the knee flexes, the axis of rotation moves within the distal femur and the kinematics are affected by the loads imposed on the knee, but the overall effect in the intact knee is that the most anterior fibers of the ACL remain close to a constant length and thus are often described as being “isometric.” Meanwhile, the more posterior the fibers, the more they slacken as the knee flexes, up to 90 degrees flexion2,7–10 (Fig. 21-1, B). These length change patterns have been measured in a number of studies,2,7,11 and it is generally accepted that an “isometry map” can be derived from such measurements.2,9,10 A modern surgical navigation system can produce such maps in response to the surgeon moving the knee during ACL reconstruction procedures, giving a patient-specific feedback on the likely length changes associated with choices of graft tunnel positions around the intercondylar notch12 (Fig. 21-2).
Anterior Cruciate Ligament Isometry and Reconstruction
The observation that the anterior fibers of the ACL remained tight across the range of knee flexion, whereas the more posterior parts slackened, led to the belief that the anterior fibers were the most important. This was reinforced by the finding that the more anterior fibers had a greater material failure strength,13 which suggests that they have adapted to a more mechanically demanding role. A similar finding has been made for the PCL.14 These findings have been correlated with a higher collagen density in the anterior fiber bundles of both the ACL and PCL.15 A more practical reason to place a graft isometrically is that this implies the graft will not be subjected to cyclical length changes when the knee is moving, thus helping to protect it from fatigue or loosening effects. For example, O’Meara et al16 reported that isometric grafts survived cyclical motion in a continuous passive motion machine, whereas nonisometric grafts did not.
The problem with this line of reasoning is that isometry measurements depend on the ACL being intact; otherwise the kinematics may be abnormal. Even when the ACL is intact, the isometric area on the femoral condyle is influenced sensitively by the loads imposed on the knee while it is being moved. This was shown by Zavras et al,17 who published a map showing a range of different recommended isometric graft locations from the previous literature (Fig. 21-3). Their reproduction of the published works confirmed that isometric behavior could be found reliably for attachment points only at the extreme anteroproximal corner of the natural ACL attachment area.2,9 This means that “isometric” ACL reconstructions are nonanatomical, with the femoral graft tunnel centered higher and deeper in the notch (with the knee flexed) than the natural attachment area. Despite this, the mainstream of opinion through the 1990s favored femoral graft tunnels placed isometrically. Although many clinical papers were published to report a high percentage of good and excellent results, there remained a high level of interest in ACL research and development, reflecting an underlying dissatisfaction with clinical outcome and a desire to find ways of improvement.
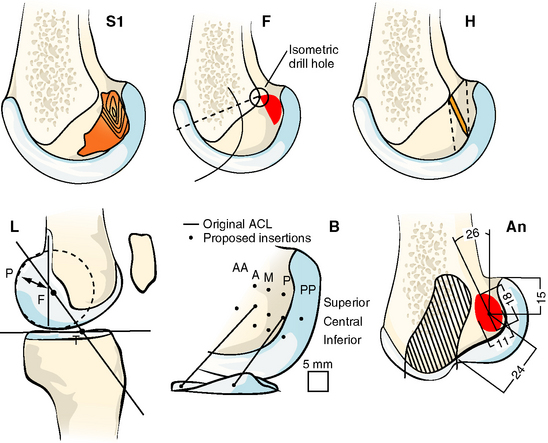
Fig. 21-3 Published isometric graft attachment sites: S1, Sidles et al10; F, Friederich and O’Brien9; H, Hefzy et al2; L, Cazenave and Laboureau31; B, Blankevoort et al32; An (anatomic), Odensten and Gillquist.33
(Reproduced from Zavras TD, Race A, Bull AMJ, et al. A comparative study of isometric points for ACL reconstruction. Knee Surg Sports Traumatol Arthrosc 2001;9:28–33, with kind permission of Springer Science and Business Media.)