CHAPTER 4 Extremity Trauma
Knowing the important steps in interpretation of skeletal trauma is useful for increasing diagnostic accuracy (Box 4-1). Fracture terminology and a guide to fracture description are summarized in Box 4-2 and Box 4-3.
Box 4-2 Fracture Terminology
Box 4-3 Fracture Description
UPPER EXTREMITY
The following diagram shows sites of common fractures of the upper extremity.
Fractures of the Scapula (Box 4-4)
neck, glenoid, acromion process, and coracoid process. Approximately 80% of fractures of the scapula involve either the body (Fig. 4-1) or the neck. Glenoid and neck fractures have the potential to threaten the function of the shoulder girdle, as malalignment of these fractures can contribute to instability of the glenohumeral joint, arthrosis, and dysfunction of the rotator cuff and girdle musculatures. They may require open reduction and internal fixation. Fractures that pass through the suprascapular notch or spinoglenoid notch are at risk for neurovascular bundle injury. Early recognition may lead to a change in the type of therapy provided to polytrauma patients.
Fractures of the Clavicle
The primary mechanism causing fractures of the clavicle (Box 4-5) is a fall onto the superolateral shoulder. Because of the strength of the sternoclavicular ligament, the force exits the clavicle in the midshaft, resulting in the most common pattern of clavicle fracture: fracture of the middle third. The most widely used classification system (by Allman) divides clavicular fractures into three groups by location: group 1, middle third; group 2, lateral third; and group 3, medial third. Group 2 (or lateral third) fractures are further divided into three distinct types by Neer, depending on whether the coracoclavicular ligament is involved. The middle third of the clavicle (group 1) (Fig. 4-2A) is involved in 65% to 85% of all fractures. Complete fractures typically result in superior displacement of the medial fragment (due to pull of the sternocleidomastoid muscle) and inferior displacement of the lateral fragment (due to gravity pull by the shoulder joint). Overriding of fragments is common, with the lateral fragment underlying the medial fragment. The lateral third of the clavicle (group 2 fracture) is involved in 15% to 30% of cases. In this group, the integrity of the coracoclavicular ligaments influences the severity of displacement. The fracture may extend between the two portions of the coracoclavicular ligaments (medial conoid and lateral trapezoid ligaments) either without causing ligamentous disruption or involving the conoid ligament. The latter is a type II fracture that is more prone to delayed union and nonunion. A stress radiograph is often useful to make an accurate determination. Rarely, the fracture line involves only the joint margin and extends into the acromioclavicular joint. Fractures of the medial third of the clavicle (group 3) (Fig. 4-2B and C) are rare, accounting for only 5% of all clavicular fractures. These fractures are easily overlooked on conventional radiographs because of their lack of displacement and the overlapping ribs and spine. CT should be obtained when there is a question of injury to the medial third of the clavicle or the sternoclavicular joint. Unusual patterns of clavicular fractures include medial physeal separation and periosteal sleeve fracture that occur in the most medial and most lateral portions of the clavicle, respectively, in children and young adults.
Acromioclavicular Joint Injuries
Acromioclavicular (AC) injuries (Box 4-6) commonly result from a direct injury owing to a fall on the lateral aspect of the shoulder with an adducted arm during athletic activities. The initial trauma is directed at the AC joint capsule, and with greater force there is disruption of the coracoclavicular ligaments and detachment of the trapezius and deltoid muscles surrounding the joint. The name understates the importance of the status of the coracoclavicular ligaments, which are the most important structures maintaining alignment of the clavicle and scapula. Radiographic evaluation of the AC joint injury typically includes a standard anteroposterior view with the x-ray beam directed 15 degrees cephalad in the upright position. Both shoulders should be included in the same image. Stress radiographs may be performed with a patient holding a 10- to 15-pound weight if the initial radiograph without weight is normal. However, the increasing trend toward nonoperative management of even severe injuries has made stress radiographs less clinically relevant. There are six types of AC joint injuries. In type I injury (ligament sprain, intact coracoclavicular ligament), there is a normal AC joint space in radiographs obtained with and without weight bearing. In type II injury (Fig. 4-3A), the AC joint may be normal or slightly widened on nonstress radiographs. On the stress view (Fig. 4-3B), the AC joint is wide, which implies partial or complete ligament tear. In type III injury (Fig. 4-3C), there is a complete disruption of the acromioclavicular and coracoclavicular ligaments, resulting in widened acromioclavicular and coracoclavicular distances on nonstress radiographs. The distal clavicle is displaced superiorly. In type IV injury, the clavicle is displaced posteriorly; this is best visualized on the axillary projection. Type V and type VI injuries have associated separation of the sternoclavicular joint and are the result of severe trauma with other, accompanying fractures.
Subluxations and Dislocations About the Glenohumeral Joint
The glenohumeral joint (Box 4-7) is the most frequent site of dislocation of any joint in the body. Most commonly, these are post-traumatic dislocations. However, non-traumatic etiologies such as seizures and voluntary dislocations do occur. The type of dislocation is determined by the final resting place of the humeral head relative to the glenoid: anterior, posterior, superior, or inferior.
Anterior Dislocation
The vast majority of glenohumeral joint dislocations are anterior dislocations (Fig. 4-4A). This usually occurs as a result of indirect force applied to the arm in abduction, extension, and external rotation. Subcoracoid anterior dislocation is the most common subtype, followed by subglenoid, subclavicular, and intrathoracic. It is readily diagnosed on clinical exam and frontal radiographs as a medially and inferiorly located humeral head relative to the glenoid. The common associated injuries are Hill-Sachs fracture and Bankart lesion as a result of impaction of the humeral head against the glenoid during the movement of dislocation. The Hill-Sachs fracture (Fig. 4-4B) is a large defect or groove in the posterolateral aspect of the humeral head, best visualized in the internally rotated anteroposterior (AP) projection. Bankart lesions (Fig. 4-4B and C) may be composed of soft tissue (fibro-cartilaginous, cartilaginous labrum) or a piece of bone avulsed from the anteroinferior portion of the glenoid rim. They are less common than Hill-Sachs fractures. Neither one of these lesions is a sign of recurrent or previous dislocations, because they do occur at the initial dislocation.
Posterior Dislocation
Posterior dislocations of the glenohumeral joint (Fig. 4-5) usually occur with violent muscle contractions, as seen with seizures, or electric shocks. The dislocation can be either subacromial, subglenoid, or subspinous. It is an uncommon injury that frequently coexists with an impacted fracture of the anterior humeral head due to trauma to the humeral head on the glenoid fossa during dislocation. It is difficult to detect clinically, especially in obese or muscular individuals. On frontal radiographs, posterior dislocations may go unrecognized in up to 50% of cases. Multiple signs have been described as helpful for detection of this subtle injury on frontal projections. The “positive rim” sign is an increase in the distance between the articular cortex of the humeral head and the anterior glenoid rim to more than 6 mm. The “light bulb” sign is a humeral head fixed in internal rotation, resembling a light bulb. The “trough line” is a curvilinear dense line parallel to the articular margin of the humeral head, representing an impacted fracture of the anterior aspect of the humeral head that coexists with the dislocation.
Fractures of the Proximal Segment and Shaft of the Humerus
Fractures of the Proximal Humerus
The proximal humerus consists of the humeral head, anatomical neck, greater tuberosity, lesser tuberosity, surgical neck, and proximal shaft. Fractures of the proximal humerus (Box 4-8) are associated with osteoporosis. The majority of fractures are the result of indirect forces such as a fall onto an outstretched arm. Patterns of fracture and displacement are dictated by the position of the humerus at the time of injury, by bone quality, and by the direction of muscular pull on humeral fracture fragments. By counting the number of major displaced fragments and defining specific parts of involvement (head, greater tuberosity, lesser tuberosity, and shaft), Neer classified fractures of the proximal humerus into one-, two-, three-, and four-part fractures. Each of the four fracture sites results in a potential fragment, or “part.” A fragment is considered a “part” if it is displaced more than 1 cm or rotated more than 45 degrees. Regardless of the number of fracture lines, a lesser degree of displacement is considered to be minimal. One-part fractures (Fig. 4-6A) are nondisplaced or minimally displaced fractures. Muscles inserting on the proximal humerus influence the direction of fragment displacement fragments. The pectoralis major pulls the humeral shaft anteromedially. The supraspinatous and infraspinatous pull the greater tuberosity posterosuperiorly, and the subscapularis pulls the lesser tuberosity medially. When describing fractures of the proximal humerus, it is important to specify the type (one-, two-, three-, or four-part; surgical or anatomical neck) and the involved fragments (shaft, greater, lesser tuberosity). According to Neer’s description, 80% of fractures of the proximal humerus are one-part fractures and the majority of them are fractures of the surgical neck. Nondisplaced surgical neck fractures usually have a good prognosis because blood supply to the humeral head is preserved. On the contrary, fractures of the anatomical neck heal poorly because of the completely disrupted vascular supply that results in avascular necrosis and secondary osteoarthritis. Two-part fractures (Fig. 4-6B and C) account for 10% of all fractures of the proximal humerus. Displaced fractures of the surgical neck may injure the brachial plexus or axillary artery because these structures lie immediately anterior to the humeral head and surgical neck. Isolated fractures of the lesser tuberosity are unusual. A four-part fracture (Fig. 4-6D and E) is one in which the articular segment is isolated from both tuberosities and the humeral shaft. A “classic” four-part fracture is a fracture-dislocation, in which the articular segment dislocates anteriorly with no remaining soft tissue attachments. This results in an increased risk of osteonecrosis. Another important variant of a four-part fracture is the “valgus-impacted” fracture, which has a better prognosis due to maintained residual vascularity.
Box 4-8 Fractures of the Proximal Humerus and Shaft of the Humerus
Fractures of the Shaft of the Humerus
A humeral shaft fracture (see Box 4-8) is defined when the main fracture line is distal to the surgical neck of the proximal humerus, and proximal to the supracondylar ridge. Fractures of the humeral shaft are common. They are classified according to their location: above or below the pectoralis major insertion and above or below the deltoid insertion. Location of the fracture line affects the way the fragments are displaced. With fractures occurring above the pectoralis major insertion, the distal fragment is displaced anteromedially by the pull of the pectoralis major. Fractures occurring between the insertions of the pectoralis and deltoid muscles (Fig. 4-7) are associated with lateral displacement of the distal fragment. Fractures occurring distal to the deltoid insertion result in abduction of the proximal fragment by the deltoid and shortening of the arm by the pull of the brachialis and biceps muscles, which remain attached to the distal fragment. Associated injuries of the radial nerve are common, especially with fractures located at the junction of the mid and distal thirds. Pathologic fractures of the humerus are common in adults as a result of metastatic disease. In children, this usually occurs through a simple bone cyst of the proximal humerus.
Fractures and Dislocations Around the Elbow (Box 4-9)
The Fat Pad Sign
Normal thin layers of fat lie between the synovium and the joint capsule of the elbow anteriorly and posteriorly. In a normal flexed elbow, the anterior fat pad is visible, while the posterior fat pad is hidden in the intercondylar depression on the posterior surface of the humerus. In the presence of a joint effusion, the posterior fat pad (Fig. 4-8) becomes visible on the lateral radiograph of the flexed elbow because it is displaced posteriorly and the anterior fat pad becomes more elevated. In the acute trauma setting, elbow joint effusions can be a result of an intra-articular fracture or traumatic synovitis. This sign is usually present in children and adolescents, where 70% to 90% of patients with a posterior fat pad sign prove to have a fracture on initial or subsequent examinations. In adults, the sign is less frequently seen, and its absence cannot be used to exclude a fracture. This sign is not present in elbow dislocations, displaced intra-articular fractures with a torn joint capsule, and fracture-dislocations because it requires an intact synovium to prevent blood from mixing with extrasynovial fat. An optimal-quality lateral radiograph of the elbow is essential for evaluation of the fat pad sign. The image is taken when the elbow is flexed at 90 degrees with the hand in a lateral position. On the radiograph, three concentric arcs of the distal humerus (trochlear groove, capitellum, and medial trochlea) should be visualized.
Box 4-9 Fractures and Dislocations Around the Elbow
STANDARD VIEWS
RELEVANT NORMAL ANATOMY
Fractures Around the Elbow in Children
Lateral condylar fracture (Fig. 4-9) is the second most common type of fracture occurring around the elbow in children. This fracture is caused by a lateral blow to the forearm, resulting in varus stress across the elbow. Typically, the fracture line runs across or along the physis, with or without a small metaphyseal fragment. Fractures may terminate in the physis (incomplete) or extend beyond the physis through the ossified capitellum or unossified cartilage (complete). Differentiation between incomplete and complete fractures can be difficult because most of the fractures extend through the unossified cartilage. Additional imaging with magnetic resonance imaging (MRI) or intraoperative assessment with arthrography is often needed.
Fractures of the Distal Humerus
Type A extra-articular fractures (Fig. 4-10A and B) include apophyseal avulsion fractures (avulsion of the lateral epicondyle, medial epicondyle) and simple and complex nonarticular metaphyseal transcolumn fractures. Type B partial articular fractures (Fig. 4-10C and D) are intra-articular fractures that involve only one column (either medial or lateral) with one or more sites of intra-articular extension (capitellum, trochlea, or both). They are subtyped into lateral sagittal fractures, medial sagittal fractures, and frontal fractures. Sagittal fractures are either transcapitellar or transtrochlear fractures depending on the fracture line involvement. Frontal fractures are isolated fractures of the capitellum, trochlea, or both, without metaphyseal extension. Fractures of the capitellum account for 10% of all distal humeral fractures in adults. A shearing force transmitted by the radial head or trochlear groove results in this type of injury. The fragment is visualized on the lateral radiograph of the elbow and is characteristically displaced proximally above the radial head and coronoid process.
Type C, complete articular fractures (Fig. 4-10E and F), are fractures that involve both the medial and lateral columns, with articular extension. These fractures are further subclassified according to the nature (simple or complex) of the column fracture and the presence of intra-articular extension.
Fractures of the Proximal Radius
A radial head fracture (Fig. 4-11A and B) is the most common elbow injury in adults. It is intra-articular and usually produces hemarthrosis. It can be very subtle due to minimal cortical disruption and is usually not seen on all projections. Two patterns of fractures are usually seen: a single longitudinal fracture through the proximal articular surface, and impaction of the intact radial head into the radial neck. The former presents as a step-off or abrupt angulation of the joint surface, or depression of the fragment (double line of cortical bone). The latter creates an abrupt step-off between the normally gentle concave curve of the radial head and neck. Three types of radial head fractures were described by Mason, and later modified by Morrey to include a type IV fracture. Type I is a nondisplaced fracture, which accounts for 50% of cases. Type II is a displaced fracture without comminution, and type III is a comminuted fracture. Type IV is a radial head fracture-dislocation. The fracture is considered displaced if a fragment involves 30% or more of the articular surface and is displaced more than 2 mm. Open reduction with internal fixation is sometimes used to correct this fracture, especially with a large displaced fragment.
Presence of comminuted radial head and neck fractures due to high-energy trauma should raise suspicion for additional elbow and forearm injuries, such as capitellar and coronoid process fractures, elbow dislocation, and the Essex-Lopresti fracture. The latter consists of an unstable, comminuted fracture of the radial head and neck, and proximal migration or subluxation of the distal radioulnar joint that leads to an acute tear of the interosseous ligament of the forearm. Fractures of the olecranon (Fig. 4-11C) are the second most common type of elbow fractures in adults. They can be caused by a direct injury to the olecranon itself, which usually presents with comminution and distracted fragments, or by an indirect injury (e.g., a fall on the forearm) that results in simple fractures. If the periosteum is completely torn, traction by the triceps will displace the proximal fragment proximally resulting in a wide fracture gap. Although the fractures are usually obvious on lateral radiographs, soft tissue swelling in the olecranon bursa is an important clue to the presence of a subtle fracture.
Fractures of the coronoid process (Fig. 4-12) are usually oriented in the coronal plane and are caused by a shearing mechanism, most commonly from a posterior elbow dislocation. These fractures are pathognomonic of an episode of elbow instability. In every posterior dislocation, the coronoid process should be examined closely to exclude a fracture. A free intra-articular bone fragment within the elbow joint of an adult with posterior dislocation is most likely a displaced coronoid process fracture.
Dislocations of the Elbow
The elbow is one of the most commonly dislocated joints of the body. Elbow dislocations usually occur in young people, with a peak in individuals between 5 and 25 years of age. Dislocations of both the radius and ulna, with respect to the distal end of the humerus, are the most frequently seen type. More than 80% are posterior or posterolateral dislocations (see Fig. 4-12). Less commonly, the radius and ulna dislocate in divergent directions. Common associated injuries include fractures of the coronoid process, radial head, and medial epicondyle of the humerus. “Terrible triad” fracture-dislocations describe the combination of elbow dislocation with radial head and coronoid process fractures. This condition is prone to early recurrent instability and post-traumatic arthritis.
Fractures of the Shafts and Distal Radius and Ulna (Box 4-10)
Abnormal Pronator Quadratus Sign
Monteggia Fracture-Dislocation
Monteggia fracture-dislocation is a combination of a fracture of the proximal ulnar shaft and subluxation or dislocation of the radial head. The term “Monteggia lesion” describes a number of traumatic lesions that have in common disruption of the radial head and fracture of the ulna at any level. Four types of Monteggia fracture-dislocation (Bado classification) are described according to the direction of the displaced ulnar fracture and the dislocated radial head. Type I is the most common; it is a fracture-dislocation with volar angulation at the fracture site and volar dislocation of the radial head. Type II, or reverse Monteggia fracture-dislocation (Fig. 4-13), is more likely to be seen in adults. This type has dorsal angulation at the fracture site and dorsal dislocation of the radial head. Type III is the most severe of all Monteggia fracture-dislocations. The fracture fragments are laterally displaced, with lateral or anterolateral dislocation of the radial head. Type IV is a fracture-dislocation that includes fractures of the proximal radius and proximal ulna along with volar dislocation of the radial head. In the presence of an isolated fracture of the shaft of a forearm bone, one must always anticipate the possibility of injuries to other bones.
Galeazzi Fracture-Dislocation
Galeazzi fracture-dislocations (Fig. 4-14) are uncommon, accounting for 3% to 6% of all forearm fractures. They consist of a fracture of the distal third of the radial shaft and dislocation or subluxation of the distal radioulnar joint (DRUJ). When one is faced with an isolated radial shaft fracture, injury of the DRUJ should be sought. The following signs suggest traumatic disruption of the DRUJ: fracture of the ulnar styloid at its base, widened DRUJ space on a frontal radiograph, dislocation of the radius relative to the ulna on a lateral radiograph, and radial foreshortening.
Fractures of the Distal Radius
Colles’ fracture (Fig. 4-15A and B) is a transverse extra-articular fracture of the distal radius with dorsal displacement of the distal fragment along with the bones of the wrist and hand. It usually occurs as a result of a fall on the outstretched hand. It is commonly associated with an ulnar styloid process avulsion fracture, scapholunate dissociation, and other carpal dislocations or fractures.
The Smith fracture (Fig. 4-15C and D) is a transverse fracture of the distal radius with volar displacement of the distal fragment together with the carpus and hand; therefore the term “reverse Colles’ fracture” has been used.
The Barton fracture (Fig. 4-16A and B) is an oblique coronal fracture of the dorsal or volar margins of the distal radius with intra-articular extension. If the fracture line extends to the volar aspect of the distal radius, it is called a volar Barton fracture. The volar Barton fracture is more common than its counterpart, the dorsal Barton fracture, in which the fracture line extends to the dorsal aspect of the distal radius.
Chauffeur’s fracture (Hutchinson fracture) (Fig. 4-16C) is an intra-articular fracture of the base of the radial styloid process. The name comes from the era when hand cranking was needed to start motor vehicles. At present, it is most frequently caused by a fall on an outstretched hand, resulting in an avulsion of the radial collateral ligament, or a direct blow to the radial styloid process.
Torus fracture (buckle fracture) (Fig. 4-16D and E) is the most common fracture of the distal forearm in young children. It is usually caused by a fall on an outstretched hand. Characteristically, the fracture involves only one side of the cortex on the compression side, usually 2 to 3 cm proximal to the physis. The periosteum and cortex are intact on the side opposite to the fracture, hence this fracture has a good prognosis.
Fractures and Dislocations of the Carpus (Box 4-11)
Scaphoid Fracture
Scaphoid fractures (Fig. 4-17) are the most common fractures of the carpal bones. They occur almost exclusively in young adults due to a fall on an outstretched hand, athletic injuries, or motor vehicle accidents. A strong index of suspicion is the key to early diagnosis, because scaphoid fractures may produce only limited motion with little pain or swelling. Although a series of four radiographic views of the wrist is usually sufficient for diagnosis, up to 30% of scaphoid fractures are radiographically occult. Presence of “snuffbox” tenderness and a history of trauma, even with negative radiographs, necessitates immobilization or further imaging with CT or MRI. If immobilization is used, obtaining follow-up radiographs after 10 days usually reveals abnormalities, with either resorption of the fracture line (clearly visible lucent line) or faint sclerosis around the fracture. MRI is the current gold standard for diagnosis of wrist injuries. By excluding a fracture, MRI can eliminate the need for prolonged immobilization. CT is becoming a more common investigation in acute wrist trauma because of its widespread availability and short scan time. Scaphoid fractures are classified based on their anatomic location: proximal third (proximal pole), middle third (waist), distal articulating portion, and tubercle. The most common location is at the waist (80%), followed by the proximal pole (15%), tubercle (4%), and distal articular portion (1%). Location of the fracture determines prognosis because of the characteristic blood supply to the scaphoid. The scaphoid is supplied by two major vascular pedicles. One enters the tubercle, supplies the distal third, and accounts for 20% to 30% of the blood flow. The second one enters a foramen on the spiral groove at the waist and provides 70% to 80% of the blood supply in a retrograde fashion: from the waist to the proximal pole. The more proximal the fracture line, the greater the incidence of avascular necrosis. Stability of the fracture depends on the presence of displacement, degree of comminution, and associated injuries.
Box 4-11 Fractures and Dislocations of the Carpus
RELEVANT NORMAL ANATOMY
Triquetral Fracture
Triquetral fractures (Fig. 4-18A and B) are the second most common fractures of the carpal bones. They commonly involve the dorsal surface, as a result of a shear or chisel injury from impingement of the ulnar styloid on the dorsal aspect of the triquetrum when the wrist is forcibly dorsiflexed and ulnarly deviated. Pain and tenderness are localized to the dorsolateral portion of the wrist. A flake of bone fragment is best visualized in the lateral or pronated oblique projections. A transverse fracture of the triquetral body is easily identified on a standard posteroanterior view. However, CT may be required to determine the extent of injury.
Hamate Fracture
Fractures of the hamate (Fig. 4-18C) are rare and frequently missed on radiographs. They can involve the body or the hook of the hamate. The latter is more common and can produce significant disability. Either a direct blow to the hook or an avulsion of the transverse carpal ligament and pisohamate ligaments can cause this fracture. It is best visualized on a carpal tunnel view or on CT scan. Absence or indistinctness of the “eye” sign (an oval, dense, cortical ring shadow over the hamate) is a finding that suggests a fracture of the hook of the hamate on frontal radiographs.
Carpal Dislocations
The mechanism responsible for most carpal dislocations is an axial compression-hyperextension injury usually from a fall onto the outstretched hand. Failure of the connecting structures bridging the proximal and distal rows of the carpus results in dorsal displacement of the distal row (perilunate dislocation). If the force is more severe, a complete lunate dislocation may occur. These injuries are easily diagnosed on lateral radiographs. Perilunate dislocation (Fig. 4-19A and B) is the most common type of wrist dislocation. There is a dorsal dislocation of the carpal bones (scaphoid, triquetrum, and other carpal bones) about the lunate, while the lunate itself remains in normal position with respect to the distal radius. Loss of congruity of the three carpal arcs and an abnormal triangular configuration of the lunate with the apex pointing distally are signs on frontal radiographs. On the lateral projection, there is a normal relationship of the lunate and distal radius, but all other carpal bones are located posterior to the lunate. When it occurs in association with a scaphoid waist fracture, it is called a transscaphoid perilunate dislocation.
In lunate dislocation (Fig. 4-19C and D), the lunate is dislocated volarly with respect to the distal radius, and all other carpal bones lie dorsal to the lunate. This produces a radiographic “spilled teacup” sign on the lateral radiograph. The capitate migrates proximally and sits in the lunate fossa. On the frontal projection, the congruity of the three carpal arcs is lost, and there is superimposition of the base of the capitate and lunate. Lunate dislocations commonly occur in association with scaphoid fractures.
Carpal Instabilities
Scapholunate dissociation, or rotatory subluxation of the scaphoid (Fig. 4-19E), is a spectrum of injuries caused by interruption of the scapholunate ligament and extrinsic ligaments that stabilize this articulation, resulting in abnormal motion between the scaphoid and lunate. It is the most common pattern of carpal instability. Due to the normal compressive forces across the wrist, which tend to force the scaphoid into further palmar flexion, disruption of the scapholunate ligament seen in scapholunate dissociation will allow an abnormal degree of palmar flexion of the scaphoid. Thus, the key finding on the lateral radiograph is an increased scapholunate angle of more than 60 degrees. On frontal radiographs, there is a widening of the scapholunate space of more than 3 mm measured at or distal to the midpoint of the joint space (5 mm is considered to be pathognomonic); this is called the “Terry Thomas” sign. If in doubt, comparison between the scapholunate space and lunotriquetral space should reveal a significant widening of the former in the case of scapholunate dissociation. The “cortical ring” sign is another radiographic finding of this condition, describing the cortex of the distal pole of the palmar-flexed scaphoid seen end-on on the frontal radiograph. This type of injury can be isolated or associated with distal radial fractures. Without treatment, it may lead to scapholunate advanced collapse.
Fractures and Dislocations of the Hand (Box 4-12)
First Metacarpal (Thumb) Fractures and Dislocations
Fractures of the base of the first metacarpal account for 80% of thumb fractures. They usually result from an axial load to the partially flexed first metacarpal. Four types include epibasal (extra-articular) (Fig. 4-20A), Bennett, Rolando, and comminuted fractures. Bennett fractures (Fig. 4-20B) are intra-articular oblique fractures through the proximal articular surface of the first metacarpal, usually in two separate fragments. Due to traction by the abductor pollicis tendon, which inserts on the dorsal surface of this metacarpal, the large distal fragment together with the rest of the thumb is retracted proximally. Rolando fractures (Fig. 4-20C) are intra-articular comminuted fractures consisting of three major fragments. In Rolando and comminuted fractures (Fig. 4-20D), instead of being retracted, the large distal fragment is impacted into the two smaller fragments.
Gamekeeper’s or skier’s thumb (Fig. 4-21) signifies the injury to the ulnar collateral ligament of the first metacarpophalangeal joint owing to forceful abduction of the first proximal phalanx. The spectrum of injuries ranges from a pure ligamentous injury to an avulsion of the ulnar aspect of the distal attachment site of the ligament or a transverse fracture of the base of the proximal phalanx.
Dislocations of the Carpometacarpal Joints
A very complex network of dense carpometacarpal and intermetacarpal ligaments holds the carpometacarpal (CMC) joints together. Therefore, it requires a major force to cause injuries to this region. Dislocation at the first CMC joint usually occurs with high-energy trauma, with concomitant neurovascular injuries. Pure thumb CMC dislocation is mostly dorsal and results from axial loading on a partially flexed thumb. Of the other CMC joints, fractures and dislocations frequently occur at the fourth and fifth CMC joints (Fig. 4-22) because of their greater flexibility and mobility.
Metacarpal Fractures
Fractures of the metacarpal bones are classified according to their location; base, shaft, neck, or head. They are commonly oblique or spiral, rather than transverse, in configuration, and are usually obvious. The exception is a nondisplaced fracture at the base of the lateral metacarpal bones, which is frequently occult because of the bony configuration, superimposition in this region, and minimal displacement of fragments. Boxer’s fracture (Fig. 4-23) is a metacarpal neck fracture with volar angulation of the distal fragment as a result of a direct impact on the metacarpal head with a clenched fist. It is most commonly seen in the fifth metacarpal. The description of boxer’s fracture is somewhat misleading since professional fighters are more likely to sustain a second or third metacarpal neck fracture. Fractures of the base of the metacarpals are divided into four types: epibasal, two-part (reverse Bennett), three-part, and comminuted. In the reverse Bennett type, the flexor and extensor carpi ulnaris muscles usually retract the larger distal fragment proximally.
Phalangeal Fractures
Phalangeal fractures are more common than metacarpal bone fractures. They may be classified as either extra-articular (involving the shaft) or intra-articular (involving the proximal or distal margins). The distal phalanx is most commonly involved, accounting for more than half of hand fractures. They vary from a comminuted, longitudinal split to a transverse fracture, and frequently are associated with lacerations of the nail bed. Fractures of the proximal phalanx (P1), middle phalanx (P2), and distal phalanx (P3) (Fig. 4-24) are described according to their location (head, neck, shaft, and base) and appearance. Baseball or mallet finger (Fig. 4-25A) is a flexion deformity at the distal interphalangeal (DIP) joint due to an avulsion of the extensor tendon at its insertion on the dorsal lip at the base of the distal phalanx. The injury occurs when an extended DIP joint is forcibly flexed, as occurs when the finger is jammed by a baseball striking the tip of the finger. A small piece of bone may or may not be avulsed along with the tendon. Radiographs may reveal no fracture but only flexion deformity of the DIP joint.
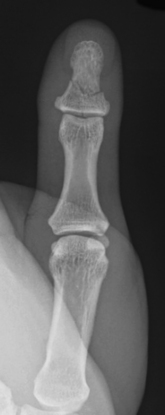
Figure 4-24 Transverse fracture of the distal phalanx. This fracture is commonly associated with nail bed injuries.
Volar plate injury (Fig. 4-25B) is an avulsion injury at the insertion of the volar plate on the volar surface of the base of the middle phalanx at the proximal interphalangeal (PIP) joint. It is a hyperextension injury that can occur with or without bony avulsion. In the latter, a small avulsed bone fragment is usually nondisplaced. The fragment is often a thin sliver of bone, minute and easily overlooked. It is best visualized on lateral or oblique projections of the involved finger.
LOWER EXTREMITY
The diagram below shows sites of common fractures of the lower extremity.
Fractures of the Pelvic Ring (Box 4-13)
Isolated Injuries Without Disruption of the Pelvic Ring
Avulsion injuries (Fig. 4-26) are secondary to sudden muscular contractions or abrupt changes of speed or direction, as seen during athletic activities. They are frequent in adolescents before closure of the apophyses because the muscles and tendons are stronger than the apophyseal attachments. Typical locations are at the anterior superior iliac spine (Sartorius), anterior inferior iliac spine (rectus femoris), ischial tuberosity (biceps femoris), and inferior margin of the pubic body (adductor group). The fractures are displaced due to the mechanical pull by the muscles and are usually obvious on radiographs at the initial injury. The contour of the displaced fragment mirrors the adjacent (donor) portion of the pelvis. These injuries, if not detected at the initial presentation, may heal with exuberant callus and radiographically mimic bone tumors.
Pelvic Ring Disruption
The most widely accepted classification of pelvic ring disruption is the Young-Burgess system. This system divides pelvic ring disruptions into four types according to the direction of forces to the pelvis: lateral compression (LC), anteroposterior compression (APC), vertical shear (VS), and combined mechanism (CM). In the LC category (Fig. 4-27A) are the transverse fractures of the pubic rami with ipsilateral or contralateral posterior ring injuries. In the APC category (Fig. 4-27B and C), there is a symphyseal diastasis or longitudinal fracture of the pubic rami and a disruption of the sacroiliac joint. In the VS category (Fig. 4-27D), there is vertical displacement anteriorly through a symphyseal diastasis and posteriorly through the sacroiliac joints, or, occasionally, the iliac wing or sacrum. The most common pattern of combined mechanism is a combined LC/VS injury.
Sacral Fractures
The majority of sacral fractures are associated with pelvic or lumbar spine fractures. They are frequently oriented in the vertical plane (only 5% to 10% are transverse). The Denis classification divides the sacrum into three zones and describes the risk of neurological deficits associated with fractures in each zone. Zone I (Fig. 4-28A) is located lateral to the sacral foramina, and fractures rarely cause neurological deficit. Zone II fractures (Fig. 4-28B) occur through the sacral foramina (transforaminal); there is an approximately 28% chance of neurological deficit. Zone III fractures involve the central canal and carry a 57% risk of neurological deficit.
Associated Pelvic Injuries
Pelvic hemorrhage is the primary concern in patients with pelvic ring disruption. To reduce the risk of bleeding, patient manipulation and movement should be restricted until conditions are stabilized. Therefore, the initial radiographic exams are restricted to the AP view of the pelvis (and/or outlet view, CT scan). Urinary tract injury is more common with specific types of pelvic fractures, such as symphyseal diastasis and pubic rami fractures. Clinically, gross hematuria is the most obvious sign of urinary tract injury associated with pelvic fractures. The presence of microscopic hematuria is rarely associated with a urinary tract injury requiring surgical intervention. If indicated, complete evaluation of the urinary tract requires CT, cystography (conventional or CT), and urethrography. Direct injury to the gastrointestinal tract is usually caused by fracture fragments lacerating the anus or rectum. The injury often extends to the perineum. For a more complete discussion of associated injuries in pelvic fractures please refer to chapters Chapters 3, 7, and 11.
Fractures of the Acetabulum
Acetabular fractures (Box 4-14) are commonly associated with pelvic fractures. They are usually the result of motor vehicle accidents. Radiographic evaluation aims to answer the following questions: whether the fracture involves the columns, walls, or dome; whether there is any entrapped bone fragment in the joint; and if there is an associated femoral head fracture. CT better defines the degree of rotational displacement, presence of intra-articular fragments, articular impaction, and femoral head injury. The Judet-Letournel system classifies fractures of the acetabulum into 10 different types: 5 elementary and 5 associated types. The elementary type is a simple fracture that runs in one main direction, including fractures of the anterior column, posterior column (Fig. 4-29A and B), anterior wall, posterior wall, and a transverse fracture. Column fractures involve the acetabulum and its corresponding pubic ramus (anterior column fractures have superior pubic ramus involvement and posterior column fractures have inferior pubic ramus involvement). It should be noted that there is no anatomic boundary separating the anterior and posterior columns. Acetabular column fractures are named according to the portion of the acetabulum that is separated from the intact ilium. Wall fractures differ from column fractures in that they separate only a portion of the column’s articular surface. Associated-type fractures are complex; the fracture line runs in more than one plane. Because of the complexity of the anatomy and the fracture classification, it is easier to define an acetabular fracture as one of three main types: wall fracture, column fracture, or transverse fracture. Over 90% of acetabular fractures fall into five common types: associated both column (ABC) fracture, T-shaped fracture, transverse fracture plus posterior wall, transverse fracture, and posterior wall fracture.
Box 4-14 Fractures of the Acetabulum
RELEVANT NORMAL ANATOMY
Posterior Wall Fractures
Posterior wall fractures (Fig. 4-29C) are the most common type of acetabular fractures, accounting for one third of them. There is disruption of a variable amount of the posterior wall of the acetabulum. The fracture may have one single fragment or multiple fragments, the latter being more common.
Transverse Fractures
The transverse fracture line (Fig. 4-29D) separates the innominate bone and acetabulum into two segments: a superior iliac segment and an ischiopubic segment containing both rami. It disrupts both the iliopectineal and ilioischial lines and crosses the acetabulum horizontally or obliquely. Its frequency ranges from 5% to 19% of all acetabular fractures.
Hip Dislocations and Fractures of the Proximal Femur (Box 4-15)
Hip Dislocations
Posterior dislocation of the hip (Fig. 4-30) is the result of an abrupt deceleration injury such as occurs in motor vehicle accidents when the knee strikes the dashboard. It is commonly associated with fractures of the posterior rim or wall of the acetabulum. In adolescents, this injury may cause separation of the capital femoral epiphysis. On frontal radiographs, the femoral head is dislocated from the acetabulum and lies in a superolateral position. The involved femoral head appears smaller than the contralateral one because it is located closer to the radiographic plate (or film), and there is internal rotation of the femur. The injury is obvious on a true lateral projection. Osteonecrosis of the femoral head and secondary osteoarthritis of the hip joint are known complications.
Box 4-15 Hip Dislocations and Fractures of the Proximal Femur
Fractures of the Femoral Neck
Subcapital fracture (Fig. 4-31A and B) is the most common type and may be extremely subtle. There is a disruption and angulation at the junction of the superior cortex of the femoral neck with the femoral head. The Garden classification categorizes subcapital fractures into four stages based on the position of the principal (medial) compressive trabeculae of the femoral head and neck and the acetabular trabeculae on frontal radiographs. Stage I fractures are incomplete fractures that spare the inferior femoral neck trabeculae. There is abduction of the superior portion of the femoral neck due to external rotation of the femoral shaft and valgus of the femoral head. The principal compressive trabeculae of the head and neck are aligned in a mild valgus position. Stage II fractures are complete fractures without displacement. The principal compressive trabeculae of the femoral head and neck are aligned in mild varus or near anatomic position. Stage I and stage II fractures are generally stable and have a good prognosis. However, when this fracture is subtle and impacted in near anatomic position, it may permit ambulation and be relatively painless. Stage III fractures are complete fractures with partial displacement. There is more severe varus alignment of the trabeculae of the femoral head and neck. In addition, the trabeculae of the femoral head are not aligned with the acetabular trabeculae. Stage IV fractures are complete fractures with full displacement. The femoral shaft is not only externally rotated but also telescoped, and the femoral head remains in anatomic alignment with the acetabulum; therefore the trabeculae of the femoral head and the acetabulum are parallel.
Trochanteric Fractures
Isolated fractures of the greater trochanter (Fig. 4-31C) are common in osteoporotic women with a history of fall on their lateral side. The normal irregularity in this region makes it difficult to detect this type of fracture, especially when it is not displaced.
Isolated fractures of the lesser trochanter in children or adolescents are usually due to avulsion injuries of the apophysis from a forceful pull by the iliopsoas muscle tendon, which inserts on the lesser trochanter. In adults, an isolated lesser trochanter fracture (Fig. 4-31D) is unusual and should raise the suspicion of underlying pathology, especially metastatic disease.
Intertrochanteric Fractures
Intertrochanteric fractures (Fig. 4-31E) are the most common type of extracapsular fracture of the proximal femur. These fractures occur in an older population than do femoral neck fractures; men and women are equally affected. They are classified according to the number of separate fragments into two-, three-, or four-part, depending on the involvement of the lesser or greater trochanter. The main fracture line is usually an oblique line extending along the plane that joins the greater and lesser trochanters. Type 0 fractures are nondisplaced and most subtle. Type I fractures are two-part fractures. Type II fractures are three-part fractures consisting of a proximal fragment, a distal fragment, and either trochanter. Type III fractures are four-part fractures consisting of a proximal fragment, a distal fragment, and both trochanters. The status of the lesser trochanter is a very important component of intertrochanteric fractures because, if fractured, it typically includes a large fragment of cortex of the femoral neck. Comminution of the posteromedial cortex, subtrochanteric extension, or a reverse obliquity pattern (when the main fracture line runs perpendicular to the line joining the greater and lesser trochanters) makes the fracture unstable.
Fractures and Dislocations Around the Knee (Box 4-16)
Hemarthrosis/Lipohemarthrosis
Box 4-16 Fractures and Dislocations Around the Knee
RELEVANT NORMAL ANATOMY
On the other hand, the presence of blood mixed with liquid fat in the knee joint, or lipohemarthrosis (Fig. 4-32), suggests the presence of an intra-articular fracture of the distal femur or proximal tibia. The fracture permits the flow of blood and liquid fat from the medullary cavity into the joint space. The sign is visualized in a horizontal-beam (cross-table) lateral radiograph taken with the patient in the supine position. The joint capsule is distended with a layering fat–blood interface that resembles an air–fluid level, called the “fat–blood interface” (FBI) sign. The presence of lipohemarthrosis is very useful as it aids in the identification of a minimally displaced intra-articular fracture. Pneumolipohemarthrosis and pneumohemarthrosis have a very similar radiologic characteristic, except that air has greater lucency than fat. It signifies an open, intra-articular fracture of the distal femur or proximal tibia.
Fractures of the Distal Femur
Fractures of the distal femur are caused by a severe axial load with varus, valgus, or rotational force. In younger individuals, these are usually a result of motor vehicle accidents. In elderly populations, a minor slip and fall on a flexed knee can result in these types of fractures. The supracondylar area of the femur is defined as the zone between the femoral condyles and the junction of the distal metaphysis and diaphysis of the femur. There are three types of supracondylar fractures, classified by the AO/OTA: extra-articular supracondylar, intra-articular unicondylar, and intra-articular bicondylar. Extension to the knee joint is frequently the result of a vertical intercondylar component. “Floating knee” (Fig. 4-33) is a term used when these fractures are associated with tibial shaft fractures, creating a free, large, segmental fragment that includes the knee joint. The incidence of arterial injury is low at 2% in supracondylar fractures.
Knee Dislocations
Knee dislocation is a rare injury. It is classified according to the relationship of the tibia with the femur into anterior, posterior, medial, lateral, and rotational dislocations. Anterior dislocation (Fig. 4-34A and B) is the most common type, resulting from severe hyperextension. The incidence of vascular injuries in knee dislocations varies from 7% to 64% in different series. Clinical evidence of vascular injuries should be sought in every knee dislocation. Knee dislocations are commonly associated with avulsion fractures of the tibial articular surface and with tears of the cruciate ligaments, joint capsule, and extracapsular ligaments. Injuries to these stabilizing structures are best evaluated with MR.
Patellar Dislocations
The patella is normally located in the sulcus of the anterior distal femur. Dislocations of the patella (Fig. 4-34C and D) are caused by excessive internal rotation of the femur on a fixed foot, and are usually relocated prior to clinical presentation. These dislocations are invariably lateral, with disruption of the medial retinaculum. The preferential lateral dislocation occurs because the lateral femoral condyle arc is shallower than the medial condyle. Patellar dislocations may be associated with chondral or osteochondral fractures, which typically arise from the lateral margin of the lateral femoral condyle or from the articular surface of the medial patellar facet. Presence of fragments in the joint space may impair joint motion and lead to locking or degenerative changes of the knee. The classic MR imaging findings of recent patellar dislocation include hemarthrosis, disruption of the medial patellar retinaculum, lateral patellar tilt, and bone contusions in the lateral femoral condyle and medial patellar facet.
Fractures of the Patella
The majority of fractures of the patella are caused by a direct blow from a fall or motor vehicle accident. Approximately 60% of patellar fractures involve the mid-body and are transverse (Fig. 4-35A and B), 25% are stellate or comminuted, and 15% are vertical (Fig. 4-35C). Displacement is present when there is separation of the fragments of more than 3 mm, or articular incongruity of 2 mm or greater. Displaced fractures may occur at the proximal or distal pole. The former may cause a low-lying patella due to the unopposed pull from the patellar tendon inferiorly (patella baja) (Fig. 4-35D). Alternatively, patella baja is seen in patients with quadriceps tendon tear. A high-riding patella (patella alta) (Fig. 4-35E and F) may be seen in distal pole fractures or in patients with patellar tendon tears.
Fractures of the Tibial Plateau
Fractures of the tibial plateau are severe injuries caused by high-speed motor vehicle accidents or falls from high altitudes. Axial loading, valgus stress, or both is the usual mechanism. The fractures may involve the medial plateau, the lateral plateau, or both, with extension into the metadiaphysis of the tibia. Fractures of the lateral plateau are the most common type because of the stronger cancellous trabeculae of the medial plateau. As a result, an isolated medial plateau fracture is the least frequent type. Schatzker’s widely used classification system categorizes tibial plateau fractures into six types according to fracture appearance, location, and extension. Type I is a split fracture of the lateral plateau. The fracture line causes a wedge-shaped fracture fragment. Type II (Fig. 4-36A and B) is a split-depression fracture of the lateral plateau. In addition to a cleavage fracture of the lateral plateau, the articular surface is depressed into the metaphysis. Type III (Fig. 4-36C) is a pure depression fracture of the lateral plateau with an intact osseous rim. Type IV is a medial plateau fracture, subdivided into IVA (split) and IVB (depressed) subtypes. In type V, the fracture line has the configuration of an inverted “Y.” The metaphysis and diaphysis remain intact. Type VI (Fig. 4-36D and E) is a tibial plateau fracture with dissociation between the tibial metaphysis and diaphysis. These fractures may involve either one or both plateaus, and have varying degrees of comminution. The goal of imaging is to demonstrate the number of fracture fragments, degree of displacement, degree of depression, location, and type of plateau fractures. Meniscal injuries, especially of the lateral meniscus, are seen in up to 50% of cases of tibial plateau fractures. Injuries to the medial collateral ligament and anterior cruciate ligament are also common.
Avulsions About the Knee
Avulsion of the Anterior Cruciate Ligament Insertion
The distal attachment of the anterior cruciate ligament (ACL) is at the medial spine of the intercondylar eminence of the tibia. Avulsions of the medial tibial spine usually occur in children or adolescents. Radiographic findings include a fracture at the base of the intercondylar eminence, or of the medial tibial spine (Fig. 4-37A), which indicates that the ACL has been detached. It should be noted that isolated fractures of the lateral tibial spine do not involve either cruciate ligament. In some cases, the presence of avulsed bony fragments in the joint may have an appearance similar to a loose body or an osteochondral fracture.
Avulsion of the Posterior Cruciate Ligament Insertion
The posterior cruciate ligament (PCL) inserts distally on the posterior aspect of the tibial plateau. An avulsed bony fragment from this type of fracture is best visualized on the lateral radiograph as a fragment projecting over the posterior aspect of the plateaus (Fig. 4-37B and C).
Segond fracture is an avulsion fracture at the lateral aspect of the lateral proximal tibia just below the level of the tibial plateau (Fig. 4-37D) and is the result of lateral capsular ligament pull. This fracture should be differentiated from an iliotibial band avulsion of Gerdy’s tubercle, which is located more anteriorly, and should not be confused with an avulsion of the lateral collateral ligament that inserts on the fibular head. There is widening of the lateral tibiofemoral compartment of the knee joint, a small sliver of bone adjacent to the lateral tibial plateau in the mid-coronal section near the joint line, and joint effusion. It is caused by forceful internal rotation of a flexed knee. ACL and meniscal injuries are frequently associated with Segond fractures.
Fractures and Dislocations Around the Ankle
Fractures of the Ankle
Ankle fractures (Box 4-17) are most commonly classified by their appearance on radiographs. Certain radiographic features can be used to determine the mechanism and to understand these complex injuries. Two major mechanisms responsible for ankle fractures are rotational and axial-loading injuries. Rotational malleolar fractures are less severe injuries than axial-loading tibial plafond or pilon fractures.
Box 4-17 Fractures and Dislocations Around the Ankle
STANDARD VIEWS
RELEVANT NORMAL ANATOMY
Rotational malleolar fractures are caused by combinations of different forces: supination, pronation, external rotation, adduction, and abduction. These forces result in either impaction injury or avulsion injury to the malleoli. The characteristic of impaction injury is an oblique fracture line, while the characteristic of an avulsion injury is a horizontal fracture line. These fractures are typically described by the location of malleolar fracture lines (medial, lateral, or posterior malleoli, or more). Bimalleolar or trimalleolar fractures are injuries that involve more than one structure. The detailed description should include the level of the fibular fracture, orientation of the fracture line (horizontal or oblique), possibility of syndesmosis disruption, involvement of the tibial plafond, and size of the various fragments. Several classifications are used in the assessment of ankle fractures. The Weber/AO classification is simple and correlates well with treatment and prognosis. The level of the fibular fracture determines the extent of injury to the syndesmotic complex, and is used to categorize fractures in this classification. In general, the higher the fibular fracture, the more extensive the damage to the syndesmosis and the greater the risk of ankle instability. Type A (Fig. 4-38A) is a supination-adduction injury with the fibular fracture located below the syndesmosis. There is a transverse fracture of the lateral malleolus or lateral collateral ligament rupture and an oblique fracture of the medial malleolus. Type B (Fig. 4-38B) is a supination-external rotation or pronation-abduction injury resulting in a fracture of the fibula at the level of the syndesmosis. There is an oblique fracture of the lateral malleolus and a horizontal fracture of the medial malleolus or deltoid ligament rupture. There is partial disruption of the syndesmosis. Type C is a pronation-external rotation injury with a fracture of the fibula above the level of the syndesmosis. There is a transverse fracture of the medial malleolus or deltoid rupture, and rupture of the syndesmosis. Type C (Fig. 4-38C) is usually treated with syndesmotic fixation in addition to stabilization of the lateral malleolus. Maisonneuve’s fracture (Fig. 4-38D and E) is part of the spectrum of pronation-external rotation injuries, in which the fibular fracture is more proximal and there is a tear of the full length of the interosseous membrane. Fractures of the proximal fibula are easily overlooked because of the distracting painful injuries at the ankle. The diagnosis of Maisonneuve’s fracture should be considered in the following situations: isolated fracture of the posterior lip of the tibia, isolated displaced fracture of the medial malleolus, and widening of the medial or lateral clear spaces without evident fracture of the lateral malleolus or fibula. In these circumstances, full-length views of the tibia and fibula should be obtained to identify associated fractures of the proximal fibula.
Axial loading to the ankle joint drives the talus against the distal tibial articular surface, resulting in tibial plafond fractures (Fig. 4-38F). The malleoli usually maintain an anatomic relationship with the talus. These fractures are more severe than the rotational malleolar fractures. They are comminuted, intra-articular fractures of the distal tibia. Differentiation of these fractures from trimalleolar fractures lies in the profound comminution of the distal tibia, intra-articular extension to involve the dome of the plafond, common association with talar fractures, and preservation of the tibiofibular syndesmosis. Post-traumatic arthritis is a common complication of displaced plafond fractures. Tillaux fracture is an avulsion of the anteroinferior tibiofibular ligament from the lateral distal tibial margin, created by forceful external rotation of the foot. The vertical fracture line extends from the distal articular surface of the tibia superiorly to the lateral tibial cortex. If there is lateral displacement of more than 2 mm, or step-off of the articular surface of the distal tibia, surgical fixation is usually required. In children, this fracture is a Salter Harris type III, since the medial side of the growth plate fuses earlier than the lateral side. In adults, this is usually a ligamentous injury. An avulsion fracture of the medial margin of the fibula at the attachment of the anterior tibiofibular ligament is known as the Wagstaffe-LeFort fracture. Isolated Tillaux fractures and Wagstaffe-LeFort fractures do occur, but are more commonly part of more extensive injuries to the ankle mortise and are associated with malleolar fractures.
Triplane fractures (Fig. 4-39A–C) are distinct fractures of the distal tibial epiphysis, caused by plantar flexion and external rotation. There are fracture lines in three planes: a horizontal plane within the growth plate or physis, a sagittal plane through the epiphysis, and an oblique vertical plane in the posterior distal metaphysis. There are two distinct types of triplane fractures: two-fragment and three-fragment fractures. The appearance of the two-fragment fractures is very similar to that of Tillaux fractures, except that there is a metaphyseal component on the lateral view. In the three-fragment fracture, the entire epiphysis is usually displaced posteriorly, whereas in a two-fragment fracture only a portion of the epiphysis is displaced. It is important to distinguish between the two because three-fragment fractures usually require open reduction and internal fixation to preserve the joint.
Fractures and Dislocations of the Foot (Box 4-18)
Injuries to the Hindfoot
Subtalar or peritalar dislocation is a rare injury characterized by concomitant dislocations of the subtalar and talonavicular joints with normal tibiotalar relationship. Findings are obvious on lateral radiographs, with absence of the talar head in the cup of the navicular. Medial subtalar dislocation (Fig. 4-40A and B) is far more common than lateral subtalar dislocation, while the latter is more severe. Total talar dislocation is a combined complete dislocation of both the tibiotalar (ankle) and subtalar joints. It is the most serious of all talar injuries.
Fractures of the Talus
Talar fractures are the second most common site of tarsal fracture and are usually caused by forced dorsiflexion. Fractures are classified by anatomic location: head, neck, body, posterior process, and lateral process. Fractures of the talar head are rare; they usually involve the talonavicular joint and are associated with talonavicular subluxation. The talar neck is the most vulnerable site. Fractures of the talar neck (Fig. 4-40C) are defined when the fracture line is anterior to the lateral process of the talus. They are usually oriented in a vertical plane and not displaced. Hawkins’s classification divides these fractures into four types. Type I is a nondisplaced fracture. Type II is a fracture with subtalar subluxation or dislocation. Type III is a fracture with subtalar and tibiotalar dislocation. Type IV is a fracture with subtalar subluxation and dislocation of the talonavicular joint. The risk of avascular necrosis is 15%, 40% to 50%, and 100%, respectively, for types I, II, and III/IV. Aviator’s astragalus is an eponym for a displaced fracture of the talar neck that results in medial displacement and rotation of the distal fragment together with the bones of the foot. Fractures extending into, or posterior to, the lateral process of the talus are defined as fractures of the talar body. These are intra-articular fractures and have a high rate of avascular necrosis. Fractures of the posterior process of the talus are uncommon and best seen on the lateral view. An os trigonum is the main differential diagnosis, although this usually has a well-corticated edge and smooth surface. Isolated fractures of the lateral process are frequently overlooked and misinterpreted as a severe sprain. This is usually an avulsion fracture and best visualized on the mortise view.
Fractures of the Calcaneus
The calcaneus is the most common site of tarsal bone fracture. Fractures are classified by their location and the presence of subtalar joint involvement. Calcaneal fractures with subtalar joint involvement (Fig. 4-40D and E) are more common (75% of all calcaneal fractures). They result from axial-loading forces to the body of the calcaneus through the talus. Typically, there is loss of height, increased width, and disruption of the subtalar joint facet. Boehler’s angle is generally decreased. The axial-loading force produces two separate fracture lines: shear and compression lines. The shear fracture line is a sagittal plane fracture through the posterior facet, dividing it into sustentacular and tuberosity fragments. The tuberosity fragment is usually impacted, rotated, and displaced laterally, resulting in loss of calcaneal height and increased calcaneal width. The compression fracture line is a coronal plane fracture that splits the middle facet. When viewed from the lateral side, this line has the shape of an inverted Y. The Sander classification relies on the number of articular pieces of the posterior facet of the subtalar joint; prognosis worsens as the number of fragments increases. Occasionally, there is ligament entrapment between the fragments, or involvement of the sinus tarsi. Calcaneal fractures are frequently bilateral, and 10% are associated with fractures of the thoracolumbar spine.
Tarsometatarsal (Lisfranc) Injuries
Injuries to the tarsometatarsal joint range from a stable sprain to a grossly unstable deformity. These injuries can result in a prolonged recovery period and significant long-term disability, and it is therefore important to recognize and treat them early. Up to 20% are missed on initial presentation. They are commonly the result of indirect forces that cause plantar hyperflexion across the long axis of the foot. This can occur with minimal trauma (e.g., foot entrapment on the car brake pedal, or a minor fall), and as a sports-related injury (e.g., opponent falling on the foot in fixed equinus). A key anatomic structure related to this type of injury is Lisfranc’s ligament. This is the strongest and largest ligament of this joint complex, originating from the lateral plantar aspect of the medial cuneiform and inserting on the medial plantar aspect of the second metatarsal base. It is an indirect link between the first and second metatarsals, and the only ligamentous support between the medial portion of the forefoot and the rest. The nidus of Lisfranc’s injuries is typically at the medial cuneiform and the base of the second metatarsal, where Lisfranc’s ligament is located. This ligament may be torn, without fractures of the medial cuneiform or base of the second metatarsal, or may be intact with a fracture of the base of the second metatarsal. Two major types of these injuries are described. The homolateral type (Fig. 4-41) is a lateral dislocation of the four lateral metatarsals or all metatarsals. The divergent type is a less common, but more severe injury. In this type, there is lateral displacement of the second through fifth metatarsals with medial displacement of the first metatarsal. Rarely, an isolated medial dislocation of the first metatarsal and medial cuneiform may occur. The most common fractures associated with these dislocations are fractures of the base of the second metatarsal. Chip fractures of the distal margin of the cuboid, and base of other metatarsals, are frequent.
Injuries to the Forefoot
Metatarsal Fractures
The majority of injuries to the fifth metatarsal are related to sporting or athletic activities. These fractures are separated into two groups: proximal base fractures and distal spiral or dancer’s fractures. There are three distinct fracture patterns of the proximal fifth metatarsal as defined by the location of the fracture line. Zone 1 injury (Fig. 4-42A) is an avulsion fracture at the base of the fifth metatarsal that usually occurs from an indirect load. Tension is produced along the insertion of the lateral band of the plantar aponeuroses during sudden inversion of the hindfoot, causing disruption of the bony cortex. Zone 2 injuries (Fig. 4-42B) are true Jones fractures and involve the metadiaphyseal junction of this bone. The fracture line propagates from the lateral aspect of the proximal metatarsal toward the articular surface between the fourth and fifth metatarsal bones and may progress into the metatarsocuboid joint. It is the result of tensile stress along the lateral border of the metatarsal. Zone 3 injuries are located in the proximal fifth metatarsal. Relative frequency of these fractures is 93% zone 1, 4% zone 2, and 3% zone 3. An apophysis of the fifth metatarsal should not be mistaken for a fracture as it has an obliquely longitudinal orientation to the axis of the metatarsal. Dancer’s fracture is a spiral, oblique fracture of the distal fifth metatarsal caused by rolling over the outer border of the foot.
SPECIAL CONSIDERATIONS
Brandser E. The pelvis. In: Rogers L.F., editor. Radiology of Skeletal Trauma. 3rd ed. Philadelphia: Churchill Livingstone; 2002:930-1029.
Butters K.P. Fractures of the scapula. In: Bucholz R.W., Heckman J.D., Court-Brown C., editors. Rockwood and Green’s Fractures in Adults. 6th ed. Philadelphia: Lippincott Williams & Wilkins; 2006:1257-1284.
Daftary A., Haims A.H., Baumgaertner M.R. Fractures of the calcaneus: A review with emphasis on CT. Radiographics. 2005;25:1215-1226.
Early J.S. Fractures and dislocations of the midfoot and forefoot. In: Bucholz R.W., Heckman J.D., Court-Brown C., editors. Rockwood and Green’s Fractures in Adults. 6th ed. Philadelphia: Lippincott Williams & Wilkins; 2006:2337-2400.
Egol K.A., Koval K.J. Fractures of the proximal tibia. In: Bucholz R.W., Heckman J.D., Court-Brown C., editors. Rockwood and Green’s Fractures in Adults. 6th ed. Philadelphia: Lippincott Williams & Wilkins; 2006:2000-2029.
Falchi M., Rollandi G.A. CT of pelvic fractures. Eur J Radiol. 2004;50:96-105.
Gaebler C. Fractures and dislocations of the carpus. In: Bucholz R.W., Heckman J.D., Court-Brown C., editors. Rockwood and Green’s Fractures in Adults. 6th ed. Philadelphia: Lippincott Williams & Wilkins; 2006:857-908.
Galatz L.M., Williams R.Jr. Acromioclavicular joint injuries. In: Bucholz R.W., Heckman J.D., Court-Brown C., editors. Rockwood and Green’s Fractures in Adults. 6th ed. Philadelphia: Lippincott Williams & Wilkins; 2006:1331-1364.
Goldfarb C.A., Yin Y., Gilula L.A., et al. Wrist fractures: What the clinician wants to know. Radiology. 2001;219:11-28.
Greenspan A. Orthopedic Imaging: A Practical Approach. Philadelphia: Lippincott Williams & Wilkins; 2004.
Harris J.H., Harris W.H. The Radiology of Emergency Medicine, 4th ed. Philadelphia: Lippincott Williams & Wilkins; 2000.
Harris R.M. Fractures of the patella and injuries to the extensor mechanism. In: Bucholz R.W., Heckman J.D., Court-Brown C., editors. Rockwood and Green’s Fractures in Adults. 6th ed. Philadelphia: Lippincott Williams & Wilkins; 2006:1970-1997.
Helms C.A., Major N.M. The knee and shafts of the tibia and fibula. In: Rogers L.F., editor. Radiology of Skeletal Trauma. 3rd ed. Philadelphia: Churchill Livingstone; 2002:1111-1221.
John S.D., Wherry K., Swischuk L.E., et al. Improving detection of pediatric elbow fractures by understanding their biomechanics. Radiographics. 1996;16:1443-1460.
Jupiter J.B., Axelrod T.S., Belsky M.R. Fractures and dislocations of the hand. In: Browner B.D., Jupiter J.B., Levine A.M., Skeletal Trauma, et al, editors. Basic Science, Management, and Reconstruction. 3rd ed. Philadelphia: WB Saunders; 2003:1153-1265.
Jupiter J.B., Kellam J.F. Diaphyseal fractures of the forearm. In: Browner B.D., Jupiter J.B., Levine A.M., Skeletal Trauma:, et al, editors. Basic Science, Management, and Reconstruction. 3rd ed. Philadelphia: WB Saunders; 2003:1363-1403.
Kasser J.R., Beaty J.H. Supracondylar fractures of the distal humerus. In: Beaty J.H., Kasser J.R., editors. Rockwood and Wilkin’s Fractures in Children. 6th ed. Philadelphia: Lippincott Williams & Wilkins; 2006:543-589.
Koval K.J., Cantu R.V. Intertrochanteric fractures. In: Bucholz R.W., Heckman J.D., Court-Brown C., editors. Rockwood and Green’s Fractures in Adults. 6th ed. Philadelphia: Lippincott Williams & Wilkins; 2006:1793-1825.
Kwon Y.W., Zuckerman J.D. Subluxations and dislocations about the glenohumeral joint. In: Bucholz R.W., Heckman J.D., Court-Brown C., editors. Rockwood and Green’s Fractures in Adults. 6th ed. Philadelphia: Lippincott Williams & Wilkins; 2006:1285-1330.
Lazarus M.D., Seon C. Fractures of the clavicle. In: Bucholz R.W., Heckman J.D., Court-Brown C., editors. Rockwood and Green’s Fractures in Adults. 6th ed. Philadelphia: Lippincott Williams & Wilkins; 2006:1211-1255.
Leighton R.K. Fractures of the neck of the femur. In: Bucholz R.W., Heckman J.D., Court-Brown C., editors. Rockwood and Green’s Fractures in Adults. 6th ed. Philadelphia: Lippincott Williams & Wilkins; 2006:1753-1791.
Leung K. Subtrochanteric fractures. In: Bucholz R.W., Heckman J.D., Court-Brown C., editors. Rockwood and Green’s Fractures in Adults. 6th ed. Philadelphia: Lippincott Williams & Wilkins; 2006:1827-1844.
Levine A.M. Fractures of the sacrum. In: Browner B.D., Jupiter J.B., Levine A.M., Skeletal Trauma:, et al, editors. Basic Science, Management, and Reconstruction. 3rd ed. Philadelphia: WB Saunders; 2003:1031-1051.
Marsh J.L., Saltzman C.L. Ankle fractures. In: Bucholz R.W., Heckman J.D., Court-Brown C., editors. Rockwood and Green’s Fractures in Adults. 6th ed. Philadelphia: Lippincott Williams & Wilkins; 2006:2146-2247.
Matta J.M. Surgical treatment of acetabular fractures. In: Browner B.D., Jupiter J.B., Levine A.M., Skeletal Trauma:, et al, editors. Basic Science, Management, and Reconstruction. 3rd ed. Philadelphia: WB Saunders; 2003:1109-1149.
Miller-Thomas M.M., West O.C., Cohen A.M. Diagnosing traumatic arterial injury in the extremities with CT angiography: Pearls and pitfalls. Radiographics. 2005;25(Suppl 1):S133-S142.
O’Brien P.J., Meek R.N., Blachut P.A., et al. Fractures of the distal femur. In: Bucholz R.W., Heckman J.D., Court-Brown C., editors. Rockwood and Green’s Fractures in Adults. 6th ed. Philadelphia: Lippincott Williams & Wilkins; 2006:1915-1967.
Potok P.S., Hopper K.D., Umlauf M.J. Fractures of the acetabulum: Imaging, classification, and understanding. Radiographics. 1995;15:7-23.
Pretorius E.S., Fishman E.K. Volume-rendered three-dimensional spiral CT: Musculoskeletal applications. Radiographics. 1999;19:1143-1160.
Reilly M.C. Fractures of the acetabulum. In: Bucholz R.W., Heckman J.D., Court-Brown C., editors. Rockwood and Green’s Fractures in Adults. 6th ed. Philadelphia: Lippincott Williams & Wilkins; 2006:1665-1714.
Resnick D., Goergen T. Physical injury: Concepts and terminology. In: Resnick D., Kransdorf M.J., editors. Bone and Joint Imaging. 3rd ed. Philadelphia: Elsevier Saunders; 2004:789-830.
Ring D. Fractures and dislocations of the elbow. In: Bucholz R.W., Heckman J.D., Court-Brown C., editors. Rockwood and Green’s Fractures in Adults. 6th ed. Philadelphia: Lippincott Williams & Wilkins; 2006:989-1049.
Robinson C.M. Fractures of the distal humerus. In: Bucholz R.W., Heckman J.D., Court-Brown C., editors. Rockwood and Green’s Fractures in Adults. 6th ed. Philadelphia: Lippincott Williams & Wilkins; 2006:1052-1116.
Rogers L.F., Ashman C.J., Yu J.S. The hip and femoral shaft. In: Rogers L.F., editor. Radiology of Skeletal Trauma. 3rd ed. Philadelphia: Churchill Livingstone; 2002:1030-1109.
Rogers L.F., Chew F.S., Hendrix R.W. Imaging of fractures: Detection and description. In: Rogers L.F., editor. Radiology of Skeletal Trauma. 3rd ed. Philadelphia: Churchill Livingstone; 2002:41-110.
Rogers L.F., Cox T.D., Sonin A. The elbow and forearm. In: Rogers L.F., editor. Radiology of Skeletal Trauma. 3rd ed. Philadelphia: Churchill Livingstone; 2002:683-778.
Rogers L.F., Keogh C., Bergin D., et al. The wrist. In: Rogers L.F., editor. Radiology of Skeletal Trauma. 3rd ed. Philadelphia: Churchill Livingstone; 2002:779-873.
Rogers L.F., Lenchik L. The shoulder and humeral shaft. In: Rogers L.F., editor. Radiology of Skeletal Trauma. 3rd ed. Philadelphia: Churchill Livingstone; 2002:593-682.
Sanders D.W. Fractures of the talus. In: Bucholz R.W., Heckman J.D., Court-Brown C., editors. Rockwood and Green’s Fractures in Adults. 6th ed. Philadelphia: Lippincott Williams & Wilkins; 2006:2249-2291.
Sanders R.W., Clare M.P. Fractures of the calcaneus. In: Bucholz R.W., Heckman J.D., Court-Brown C., editors. Rockwood and Green’s Fractures in Adults. 6th ed. Philadelphia: Lippincott Williams & Wilkins; 2006:2293-2336.
Schenck R.C.Jr., Stannard J.P., Wascher D.C. Dislocations and fracture-dislocations of the knee. In: Bucholz R.W., Heckman J.D., Court-Brown C., editors. Rockwood and Green’s Fractures in Adults. 6th ed. Philadelphia: Lippincott Williams & Wilkins; 2006:2031-2078.
Schweitzer M.E., Karasick D. The foot. In: Rogers L.F., editor. Radiology of Skeletal Trauma. 3rd ed. Philadelphia: Churchill Livingstone; 2002:1319-1406.
Starr A.J., Malekzadeh A.S. Fractures of the pelvic ring. In: Bucholz R.W., Heckman J.D., Court-Brown C., editors. Rockwood and Green’s Fractures in Adults. 6th ed. Philadelphia: Lippincott Williams & Wilkins; 2006:1583-1664.
Warner J.P., Costouros J.G., Gerber C. Fractures of the proximal humerus. In: Bucholz R.W., Heckman J.D., Court-Brown C., editors. Rockwood and Green’s Fractures in Adults. 6th ed. Philadelphia: Lippincott Williams & Wilkins; 2006:1161-1209.