CHAPTER 63 Experimental Meningioma Models
CELL LINES AND ANIMAL MODELS
Meningiomas arise from the meningothelial (arachnoidal cap) cells. They constitute approximately 20% of all intracranial tumors. About 85% of meningiomas are benign (grade I), while 10% are atypical (grade II), and 3% to 5% are malignant (anaplastic, grade III). Tumor models are critical to understand the function of genetic alterations in cancer, to evaluate their contribution to tumor initiation and progression, and to assess the toxicity and therapeutic efficacy of established and newly developed treatments. A major obstacle to research in meningioma biology and further therapeutic development has been the limited availability of long-term cell lines and animal models, which recapitulate the human clinical scenario. There has been and remains a continuing challenge to establish and maintain benign meningioma cell lines that retain the original phenotype both in vitro and in vivo similar to the original primary tumor specimen. An additional hindrance has been a lack of animal models with sufficiently high frequencies of spontaneous meningioma development and difficulties in implanting intracranial dural-based tumors to accurately model the human condition. These issues are further compounded by the lack of meningeal specific promoters. The LTAg2B was the first human leptomeningeal cell line available, although immortalization by transfection with an SV40 large T antigen gene construct under the control of the Rous sarcoma virus promoter. This cell line (LTAg2B) maintained the morphology of primary cultures and stained positive by immunohistochemistry for arachnoid-specific markers at early passages.1 The loss of intermediate filament staining in later passages may reflect a change in the phenotype of the cells owing to their high proliferative rate.
There are a few human and rat meningioma cell lines that have been established from malignant meningiomas.2–4 These cell lines have been derived from aggressive variants of meningioma, which represent only a small portion of meningiomas. There is a critical need to have additional cell lines that span the entire spectrum of meningioma histologic grades.
Most animal models have been developed by implanting human meningioma cells derived from a primary tumor subcutaneously into athymic mice. Grade I meningiomas grow very slowly with a large amount of variability in this environment.5 Recently, a novel genetic model was devised utilizing Cre recombinase technology to specifically inactivate NF2 in arachnoidal cells, resulting in the formation of intracranial meningothelial hyperplasia and meningiomas in roughly 30% of the mice.6 This powerful new technology significantly improves on prior models and may open avenues of investigation never before possible in meningioma research. This model is discussed in the latter part of this chapter.
CELL CULTURE
Cell culture provides a tool that is reliable, convenient, and, most important, reproducible for investigations into the biology of human cancers. A valid meningioma culture needs to fulfill the following requirements: (1) It must be composed of meningioma cells; (2) the growth rate of the cells should be predictable, reliable, and reproducible; and (3) the therapeutic response of the cells must be similar to that of human meningiomas. Studies clearly show that early passage cell lines (3–5) derived from benign human meningiomas undergo cellular senescence after only a few passages.7–12 Therefore any type of biological studies must be done quickly with the limited number of available cells. Usually the studies conducted on these cells are not reproducible because the cells cannot be frozen and then thawed; thus not allowing studies to be repeated in different laboratories under varied conditions. To overcome this problem, three separate studies have used hTERT to immortalize cells.
hTERT and Meningioma Cell Immortalization
The major reason for the difficulty in generating established cell lines from benign meningiomas is when the cells from these tumors are put into culture they senesce. Many pathways are involved in cellular senescence and disruption of these pathways is required for continued cell growth and immortalization.9 Low or absent telomerase activity is in part one of the reasons for senescence; progressive shortening of telomerase occurs with each subsequent cell cycle. Telomeres are repetitive DNA sequences at the ends of chromosomal DNA. Telomerase is a ribonucleoprotein polymerase enzyme, composed of a integral RNA component (hTR) and several other proteins, including a reverse transcriptase subunit (hTERT). Telomerase activity is thought to be essential for maintaining chromosome ends by adding repetitive TTAGGG sequences to the telomeres; it is linked to cellular immortality.13 Cell cycle checkpoint pathways also control cellular senescence, and successful cell immortalization may also involve disruption of the p53 and/or Rb pathways.14 In meningiomas studies have shown that telomerase activity is directly related to tumor malignancy.7,8,15 Benign tumors have low or absent telomerase activity; whereas, the majority of malignant tumors are positive. In addition, hTERT-positive meningiomas have a higher incidence of recurrence, suggesting it use a potential predictor for recurrence.16 There are three reports, including one from our group, establishing immortalized meningioma cell lines derived from grade I tumors by transduction with hTERT, the catalytic subunit of telomerase.17–19 After immortalization, the morphologic features of the cell lines remained the same as the corresponding nontransduced cells (Fig. 63-1). On seeding of the primary cells, large nuclei and tight cell junctions were observed. Cells remained positive for cytokeratin, vimentin, and S-100 by immunohistochemistry (Fig. 63-2). There is some controversy as to whether transduction with hTERT alone is sufficient for immortalization of benign meningiomas. Baia and colleagues17report that immortalization of two benign meningiomas cell lines required disruption of the p53 and pRb pathways in addition to the expression of hTERT. In contrast, Puttmann and colleagues and Cargioli and colleagues18,19 reported that transduction with hTERT alone is sufficient for immortalization of benign meningioma. This most likely reflects the genetic alterations in the original tumors from which these cell lines were derived. In addition, the doubling time of the cells transduced with both SV40 large T antigen and hTERT was shorter than the cells transduced with hTERT only. Transplantation of the hTERT-immortalized cells subdurally into nude mice resulted in tumors with typical histologic features of meningioma. Both macroscopically and microscopically, the tumors showed features of meningioma (Fig. 63-3A, B). Grossly, the tumors were well-demarcated, firm, nodular masses of tan to white tissue adherent to the dura and leptomeninges on the brain surface. Microscopically, the tumor tissue was densely cellular and was composed of spindled to epithelioid cells with monomorphic round to oval nuclei and moderate amounts of cytoplasm. The tumors showed growth patterns that varied from small nodular meningothelial-like clusters to more fascicular spindled growth. Focal whorls and microcalcifications were present in some tumors. Although nuclei displayed some pleomorphism and had visible nucleoli, other atypical features such as small cells with a high nuclear-to-cytoplasmic ratio, brisk mitotic activity, and sheet-like growth were not observed. One tumor showed focal areas of nuclear condensation and fragmentation consistent with necrosis. Immunohistochemically, the tumors showed weak positive staining for EMA and focal weak staining for S-100 (Fig. 64-3C, D). It should be noted that the histopathologic examination revealed that the cell line transduced with both SV40 large T antigen and hTERT had some atypical changes when compared with the cells transduced with hTERT only. In conclusion, these studies show that genetic manipulation can be used to overcome the senescence of primary benign meningiomas and the cell lines generated can serve as useful model systems for biological studies and evaluation of novel therapies on meningioma.
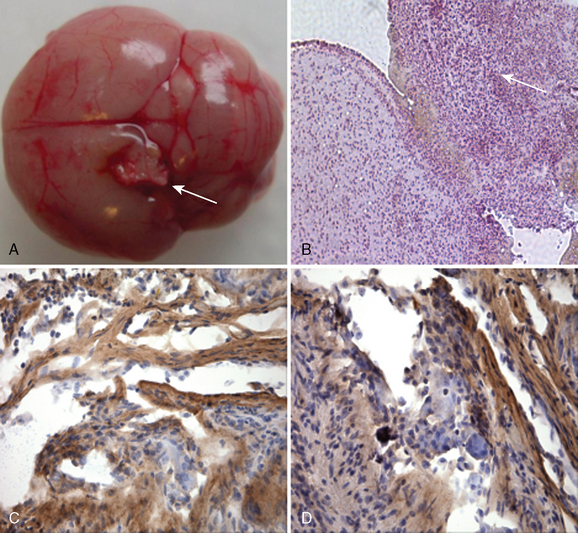
FIGURE 63-3 A, Photograph showing a grossly visible tumor attached to the cortex in the posterior parietal region of the left hemisphere. The tumor is well demarcated, firm, and white, and conforms macroscopically to meningioma characteristics observed in humans. B, Photomicrograph showing tumor tissue that is densely cellular and composed of spindled to epithelioid cells with monomorphic round to oval nuclei and moderate amounts of cytoplasm. The tumor volume was 2.03 mm3 (hematoxylin and eosin; original magnification, ×200). C, Photomicrograph showing that the tumor is weakly positive for EMA; original magnification, ×400. D, Photomicrograph showing that the tumor is weakly positive for S-100; original magnification, ×400.
(From Cargioli TG, Ugur HC, Ramakrishna N, et al. Establishment of an in vivo meningioma model with hTERT. Neurosurgery 2007;60:750–60.)
MALIGNANT MENINGIOMA CELL LINES
There have been several long-term cell lines, which have been established from malignant meningiomas.20 The most commonly used line was initiated from a tumor of a 61–year-old man with repeated recurrent primary intraosseous malignant meningioma of the skull.2