CHAPTER 313 Evaluation and Management of Craniocervical Dissociation
The CVJ is often injured in patients who die after trauma to the head or neck (or both).1,2 Craniocervical dissociation, traditionally referred to as atlanto-occipital dislocation (AOD), is associated with 6% to 8% of all traumatic motor vehicle fatalities and is the most common cervical spine injury found at autopsy in those who have died as a result of a motor vehicle accident.1,2 Conversely, reports of the successful management of AOD with good neurological recovery emphasize the importance of prompt diagnosis and management. One can expect craniocervical dissociation to account for about 1% of traumatic cervical spine injuries seen at an emergency treatment center.3,4
Anatomy and Biomechanics
The ligaments that maintain the craniocervical articulation can be divided into two groups (Fig. 313-1). The first set attaches the skull to the atlas and includes the articular capsule ligaments, the anterior and posterior atlanto-occipital ligaments, and two lateral atlanto-occipital ligaments. The anterior atlanto-occipital ligament is a continuation of the anterior longitudinal ligament, and the posterior atlanto-occipital ligament courses between the posterior border of the foramen magnum and the posterior arch of the atlas. The cruciate ligament (which includes the longitudinally oriented extensions of the transverse ligament of the atlas) also contributes to the stability of this articulation.
The primary source of stability across the CVJ is provided by a second set of ligaments that secure the cranium to the axis. These ligaments include the apical dental ligament, the alar ligaments, the tectorial membrane, and the ligamentum nuchae.5,6 The alar ligaments are paired structures with two components each: the atlantal alar and the occipital alar. These ligaments connect the tip of the odontoid to the occipital condyles and the lateral masses of the atlas, respectively.7 The alar ligaments are the main restraints to axial rotation, which occurs mostly across the C1-2 articulation. The alar ligaments also limit lateral flexion and anteroposterior translation.5
The tectorial membrane is the continuation of the posterior longitudinal ligament. It begins at the dorsal surface of the odontoid and inserts on the ventral surface of the foramen magnum.8,9 The tectorial membrane primarily resists hyperextension, although if incompetent, contact between the posterior arch of the atlas and the occiput will limit hyperextension.5,10 Flexion is restricted by contact of the odontoid process with the anterior foramen magnum.5 The apical dental ligament and the ligamentum nuchae contribute only modestly to stability of the CVJ.
Mechanisms of Injury
Craniocervical dissociation is the consequence of complete or nearly complete disruption of the ligamentous structures between the occiput and upper cervical spine. The extreme forces produced by hyperextension, hyperflexion, lateral flexion, or a combination of these forces lead to ligamentous failure.11–13 The primary force responsible for producing AOD appears to be hyperextension, which leads to rupture of the tectorial membrane.14,15 Another theory focuses on damage to the alar ligaments by extreme lateral flexion.16–18 Incompetence of the alar ligaments and tectorial membrane allows anterior dislocation of the cranium with respect to the upper cervical spine.5 Other authors have suggested that hyperflexion forces may also be involved in some cases of AOD based on the observation that the posterior elements of C1 and C2 are commonly separated in the setting of craniocervical dissociation.12
The increased incidence of craniocervical dissociation in children3,12,14 versus adults may be related to the relatively high incidence of automobile-pedestrian accidents, the pediatric anatomy of the CVJ, or both.5,12 The immature shallow, horizontally oriented surfaces at O-C1 are less biomechanically stable than the deep-seated, vertically oriented articulation in adults. Moreover, a child’s ligaments are not as stiff as an adult’s, and they are subjected to greater stress because of the need to support a proportionately larger head than in an adult for a given body size.
Clinical Findings
High-speed motor vehicle accidents and striking of pedestrians by motor vehicles are the two most common causes of craniocervical dissociation.3,12 Brainstem or upper cervical spinal cord injury at the time of dislocation probably accounts for the high mortality rate seen with AOD.19 Patients who survive may be neurologically intact or have dysfunction of the brainstem, cranial nerves, spinal cord, or cervical nerve roots.18–20 The neurological deficits range from minor to severe. Many patients also suffer a head injury, which can confound the clinical examination.
Patients with craniocervical dissociation may have normal results on motor examination, or they may display deficits, including vegetative posturing responses, cruciate paralysis, or quadriparesis. At the level of C1, the normal spinal canal is capacious. As described by Steel and codified as the Steel rule of thirds, a third of the axial spinal canal diameter is filled by the dens, a third is filled by the spinal cord, and a third is space typically occupied by cerebrospinal fluid.21 Cruciate paralysis, described by Bell, refers to weakness in the hands and arms with relative sparing of leg strength.22 This mirrors the cervical injury pattern seen clinically as described by Schneider and colleagues.23 Weakness often recovers, with improvement seen first proximally and then distally in the hands. Long held to be due to injury to the somatotopically organized crossing lateral corticospinal tract, recent evidence refutes this theory.24,25
Radiology
Although the radiographic findings in patients with craniocervical dissociation can be quite dramatic, they may be subtle or even absent on initial films.16–19 When alignment of the bony structures of the CVJ appears normal, one must appreciate indirect signs that craniovertebral dislocation may have occurred. Abnormally prominent prevertebral soft tissues should always increase suspicion for serious spinal injury. In the case of AOD, retropharyngeal hematoma is invariably present. Other signs include retropharyngeal emphysema14,26 or an increase in the interval between the posterior elements of C1 and C2.12
Several measurement protocols using plain lateral cervical radiographs to diagnose craniocervical dissociation have been developed. The basilar line of Wackenheim is drawn as a caudal extension of the posterior surface of the clivus in the midsagittal plane (Fig. 313-2A).27 Normally, Wackenheim’s line lies tangential to the posterior tip of the odontoid process and is not altered by flexion or extension. The line will intersect the dens if the occiput is displaced anterior to the atlantoaxial segment. If the skull is displaced posteriorly, the line will not intersect any portion of the odontoid process.
The interval between the odontoid tip and the basion remains constant when the CVJ is in the neutral position (Fig. 313-2B).28 A distance of greater than 5 mm in adults and 10 mm in infants has been suggested as abnormal.24,25 The sensitivity and specificity of the dens-basion interval, however, have been found to be suboptimal. Powers and coworkers reviewed 100 adult and 50 pediatric cervical spine radiographs and reported that 85% of individuals exceeded the proposed limits. With a reported average distance of 9 mm, as many as 50% of patients with known AOD might fall within the range spanned by the normal population.4
Subsequently, Powers and colleagues suggested that the ratio of two measurements could be used to define normal craniovertebral relationships (Fig. 313-2C).4 The first distance is measured between the basion (B) and the inner aspect of the posterior atlantal arch (C), and the other represents the distance between the opisthion (O) and the inner aspect of the anterior atlantal arch (A). The mean BC/OA ratio in normal subjects is 0.77, and a value greater than 1.0 may indicate AOD. Precise identification of the anatomic landmarks is necessary to properly determine the Powers ratio, and this factor may be inaccurate when congenital anomalies are present or the atlas is fractured. Additionally, in select situations of posterior or pure longitudinal craniovertebral disassociation, a value of less than 1 may be obtained.29–31
Lee and associates developed the X-line method to detect craniovertebral dissociation.29 The first line courses the distance between the basion and the midpoint of the C2 spinolaminar line (BC2Sl), and the second runs from the opisthion to the posteroinferior edge of the axis body (C2O). The BC2Sl line should tangentially intersect the posterosuperior aspect of the dens, and the C2O line should tangentially cross the most rostral edge of the C1 spinolaminar line in normal individuals older than 5 years. Although one of these linear associations may be disturbed in normal subjects, alteration of both of these relationships should lead one to be highly suspicious of the presence of AOD. Appropriate use of this method requires a normal atlantoaxial configuration, which is problematic because this segment may be abnormal in more than half of those with craniovertebral dissociation.12
Harris and associates described two measurements, the basion–posterior axial line (BAI) and the basion-dental interval (BDI), that may be used to assess the CVJ (Fig. 313-3).32 Their study of 400 adult radiographs showed that the BAI measurement did not exceed 12 mm 98% of the time and that the 95% accuracy range of the BDI was 11.8 mm. Thus, the CVJ can be considered normal when both the BAI and BDI are less than 12 mm. Although the BDI in children younger than 13 years is not reliable because of the variable age until complete ossification of the dens, the BAI measurement held true.
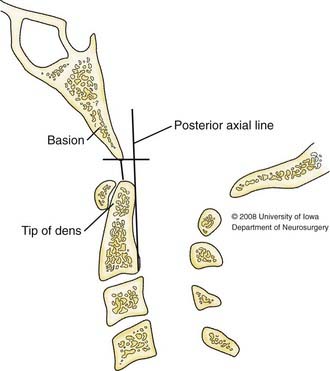
FIGURE 313-3 Schematic diagram depicting the basion–posterior axial line (BAI), and the basion-dental interval (BDI).
In a subsequent publication, Harris and colleagues compared this “rule-of-12 technique” with other methods of CVJ assessment.33 The BAI/BDI method detected craniocervical dissociation accurately regardless of the magnitude and direction of the dissociation. The Powers ratio and X-line method were unusable 46% of the time because of imaging shortcomings or C1 developmental problems. When present, AOD was correctly determined with the Powers ratio and the X-line method with much lower accuracy rates, 60% and 20%, respectively. Fisher, Deliganis, and their coworkers validated the BAI/BDI technique and found these measurements to be the most useful for diagnosing occipitoatlantal dissociation.34,35 Although probably superior to other methods of detecting AOD, interobserver and intraobserver reproducibility of the BAI/BDI methods still needs to be addressed.36
High-resolution computed tomography (CT) is an accurate means of diagnosing AOD12,29,37,38 and should include sagittal and coronal reconstructed images. A strong case can be made that fine-cut CT should be the study of choice for diagnosing AOD. In blinded tests comparing plain films and CT, with the evaluators being neurosurgeons, orthopedists, radiologists, and emergency department staff, the sensitivity, specificity, and positive and negative predictive values of AOD detection methods were improved, regardless of the measurement techniques used.39 The relevant anatomy was visualized 99.75% of the time with CT versus 39% to 84% of the time with plain films. With the ubiquity of CT scanning and shifting of the standard of care regarding AOD diagnosis, normal anatomic relationships may need to be reevaluated. Rojas and associates found that on CT the normal BDI was less than 8.5 mm 95% of the time as opposed to 12 mm on plain films.40 In addition, the BAI was difficult to interpret on CT. The accepted normal adult value for the BDI is significantly different between CT and plain films.
Additional findings on CT that may suggest craniovertebral dissociation include occipitoatlantal joint incongruity, focal hematoma, vertebral artery injury, capsular swelling, cranial nerve canal fracture, and infratentorial hematoma.35,41
A new criterion for AOD in children was described by Pang and coauthors.42 The actual occipital condyle–C1 lateral mass articulation is assessed by CT imaging, and the distance between the two articular surfaces is referred to as the condylar-C1 interval (CCI). The normal value obtained by studying 89 children was 1.28 (standard deviation [SD], 0.26 mm). Left and right normal measurements were essentially symmetrical. The CCI was then measured in a series of 16 patients who had previous radiologic criteria for AOD.43 Its diagnostic sensitivity was 100%, which was much better than that of the other standard tests: Whorley’s, 50%; Powers’, 37.5%; Harris’, 31%. The diagnostic specificity of the CCI was 100%, once again bettering the standard tests. Once the O-C1 joint is injured, it does not return to its preinjury interval. The benefits of using the CCI include essentially no logistic or technical distortions, assessment of dislocation in all three planes, and no concealment by postinjury head and neck relationships.
C1-2 distraction frequently occurs in conjunction with craniocervical dissociation.12 The lateral mass interval (LMI) between C1 and C2 was ascertained in 92 control patients undergoing CT angiography by Gonzalez and colleagues.44 The LMI, typically seen best on coronal CT images, is defined as the widest gap between the C1 and C2 lateral masses when measured perpendicular to the joint space. Control group LMIs averaged 1.77 mm (SD, 0.48; range, 0.7 to 3.3 mm). The 95th percentile range in normal patients was 0.7 to 2.6 mm. Left and right averages were essentially identical. Cup-shaped lateral mass spaces were measured at the narrowest gap interval to avoid false-positive results. When measured in patients with known distraction injuries, the mean LMI was 5.5 mm. The BDI was also calculated, with the normal group averaging 4.7 mm (SD, 1.7 mm). The average BDI in the injured group was significantly increased to 11.9 mm. Magnetic resonance imaging (MRI) was performed in the injured group and showed markedly increased signal bilaterally at the C1-2 lateral mass articulations.
There are generalized MRI findings of injury that can aid in interpreting studies to assess injury at the CVJ. Injury is usually seen as increased T2 signal in the atlantoaxial and atlanto-occipital joints, craniocervical ligaments, prevertebral soft tissues, and spinal cord.35 Injury to the anterior or posterior atlanto-occipital ligaments or the supraspinal ligament may be seen as loss of the black stripe sign and can be reliably diagnosed with MRI.45,46 In a limited number of cases, craniocervical dissociation has been diagnosed by MRI findings alone.3 Additionally, spinal cord signal abnormalities can be correlated with the results of clinical examination, and vertebral artery injury or thrombosis, or both, may be discovered.
Three specific types of craniocervical dissociation have been identified that can easily be classified on lateral cervical radiographs or sagittal CT images (Fig. 313-4).12 Type I dislocation is defined as anterior displacement of the occiput with respect to the atlas. Type II is primarily longitudinal distraction with separation of the occiput from the atlas. Type III AOD exists when the occiput is positioned in a posterior direction relative to C1. Rotatory and lateral atlanto-occipital dislocation has also been described.3,47,48
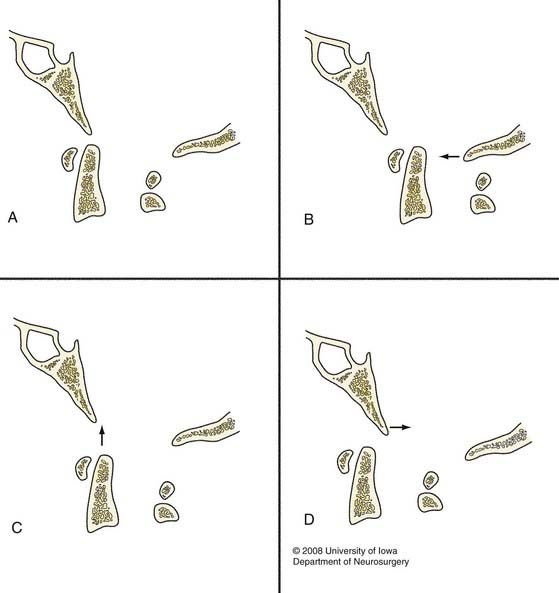
FIGURE 313-4 Classification of atlanto-occipital dislocation as proposed by Traynelis and associates.12 The normal craniovertebral junction is represented in A. Dislocations may occur as a result of anterior displacement (B), longitudinal distraction (C), or posterior displacement (D) of the occiput relative to the atlas (arrows).
Treatment Considerations
The use of traction is controversial when treating patients with craniocervical dissociation. The goal of traction, if used, is to decompress the neural elements by realigning the bony structures. Some authors have concluded that traction should not be used because the injury is highly unstable.3,17,20,31 Others have documented its benefits in achieving a successful outcome.4,16,18,19,49
The type of dislocation (I, II, or III) has been suggested as a means of guiding the decision to use traction.12 Implementation of traction is best reserved for type I (anterior), type III (posterior), and lateral craniocervical dissociation associated with neurological deficit.12,48 Traction in the setting of a type II dislocation is contraindicated because the pathology is primarily a result of longitudinal distraction, which would only be worsened by traction. Even with rotatory subluxation, small amounts of traction have resulted in a dangerous amount of distraction.3
AOD frequently heals poorly with strictly conservative measures because it is primarily a ligamentous injury with severe instability. Although external immobilization in a halo vest may allow the injury to heal, this is unlikely and maintenance of reduction may prove difficult.3,15,16,50 A more definitive approach is to proceed with posterior fusion once the patient is medically stable.3,12,20,51 In fact, Dickman and colleagues recommend bypassing traction and performing acute internal reduction and fixation.3 Dorsal fusion with rigid internal fixation that incorporates at least the occiput to C2 is required for an adequate construct.
Whether caused by immediate neurological devastation or trauma to other organ systems, AOD remains a highly lethal injury; however, patients surviving 48 hours after the traumatic event may have a good outcome. Up to a quarter of surviving patients are ultimately intact neurologically, and in another quarter only minor neurological deficits remain.12
Bono CM, Vaccaro AR, Fehlings M, et al. Measurement techniques for upper cervical spine injuries: consensus statement of the spine trauma study group. Spine. 2007;32:593-600.
Bucholz RW, Burkhead WZ. The pathological anatomy of fatal atlanto-occipital dislocations. J Bone Joint Surg Am. 1979;61:248-250.
Bucholz RW, Burkhead WZ, Graham W, et al. Occult cervical spine injuries in fatal traffic accidents. J Trauma Inj Infect Crit Care. 1979;19:768-771.
Deliganis AV, Baxter AB, Hanson JA, et al. Radiologic spectrum of craniocervical distraction injuries. Radiographics. 2000;20:S237-S250.
Dickman CA, Papadopoulos SM, Sonntag VK, et al. Traumatic occipitoatlantal dislocations. J Spinal Disord. 1993;6:300-313.
Emery SE, Pathri MN, Wilber RG, et al. Magnetic resonance imaging of the post traumatic spinal ligament injury. J Spinal Disord. 1989;2:229-233.
Gerlock AJJr, Mirfakhraee M, Benzel EC. Computed tomography of traumatic atlantooccipital dislocation. Neurosurgery. 1983;13:316-319.
Haba H, Taneichi H, Kotani Y, et al. Diagnostic accuracy of magnetic resonance imaging for detecting posterior ligamentous complex injury associated with thoracic and lumbar fractures. J Neurosurg. 2003;99(suppl):20-26.
Harris JH, Carson GC, Wagner LK, et al. Radiologic diagnosis of traumatic occipitovertebral dissociation: 2. Comparison of three methods of detecting occipitovertebral relationships on lateral radiographs of supine subjects. AJR Am J Roentgenol. 1994;162:887-892.
Harris JH, Carson GC, Wagner LK. Radiologic diagnosis of traumatic occipitovertebral dissociation: 1. Normal occipitovertebral relationships on lateral radiographs of supine subjects. AJR Am J Roentgenol. 1994;162:881-886.
Harris MB, Duval MJ, Davis JAJr, et al. Anatomical and roentgenographic features of atlantooccipital instability. J Spinal Disord. 1993;6:5-10.
Kaufman RA, Carroll CD, Buncher CR. Atlantooccipital junction: standards for measurement in normal children. AJNR Am J Neuroradiol. 1987;8:995-999.
Lee C, Woodring JH, Goldstein SJ, et al. Evaluation of traumatic atlantooccipital dislocations. AJNR Am J Neuroradiol. 1987;8:19-26.
Pang D, Nemzek WR, Zovickian J. Atlanto-occipital dislocation—part 2: the clinical use of (occipital) condyle-C1 interval, comparison with other diagnostic methods, and the manifestation, management, and outcome of atlanto-occipital dislocation in children. Neurosurgery. 2007;61:995-1015.
Pang D, Nemzek WR, Zovickian J. Atlanto-occipital dislocation: part 1—normal occipital condyle-C1 interval in 89 children. Neurosurgery. 2007;61:514-521.
Pang D, Wilberger JEJr. Traumatic atlanto-occipital dislocation with survival: case report and review. Neurosurgery. 1980;7:503-508.
Powers B, Miller MD, Kramer RS, et al. Traumatic anterior atlanto-occipital dislocation. Neurosurgery. 1979;4:12-17.
Steel HH. Anatomical and mechanical considerations of the atlanto-axial articulations. J Bone Joint Surg Am. 1968;50:1481-1482.
Traynelis VC, Marano GD, Dunker RO, et al. Traumatic atlanto-occipital dislocation. Case report. J Neurosurg. 1986;65:863-870.
Wackenheim A. Roentgen Diagnosis of the Craniovertebral Region. Berlin: Springer-Verlag; 1974.
1 Bucholz RW, Burkhead WZ, Graham W, et al. Occult cervical spine injuries in fatal traffic accidents. J Trauma Inj Infect Crit Care. 1979;19:768-771.
2 Alker GJJr, Oh YS, Leslie EV. High cervical spine and craniocervical junction injuries in fatal traffic accidents: a radiological study. Orthop Clin North Am. 1978;9:1003-1010.
3 Dickman CA, Papadopoulos SM, Sonntag VK, et al. Traumatic occipitoatlantal dislocations. J Spinal Disord. 1993;6:300-313.
4 Powers B, Miller MD, Kramer RS, et al. Traumatic anterior atlanto-occipital dislocation. Neurosurgery. 1979;4:12-17.
5 Werne S. Studies in spontaneous atlas dislocation. Acta Orthop Scand Suppl. 1957;23:1-150.
6 White AAIII, Panjabi MM. The clinical biomechanics of the occipitoatlantoaxial complex. Orthop Clin North Am. 1978;9:867-878.
7 Dvorak J, Schneider E, Saldinger P, et al. Biomechanics of the craniocervical region: the alar and transverse ligaments. J Orthop Res. 1988;6:452-461.
8 Wiesel S, Kraus D, Rothman RH. Atlanto-occipital hypermobility. Orthop Clin North Am. 1978;9:969-972.
9 Wiesel SW, Rothman RH. Occipitoatlantal hypermobility. Spine. 1979;4:187-191.
10 Harris MB, Duval MJ, Davis JAJr, et al. Anatomical and roentgenographic features of atlantooccipital instability. J Spinal Disord. 1993;6:5-10.
11 Davis D, Bohlman H, Walker AE, et al. The pathological findings in fatal craniospinal injuries. J Neurosurg. 1971;34:603-613.
12 Traynelis VC, Marano GD, Dunker RO, et al. Traumatic atlanto-occipital dislocation. Case report. J Neurosurg. 1986;65:863-870.
13 Bohlman HH, Ducker TB, Lucas JT, 2nd ed. Spine and Spinal Cord Injuries, Vol 2. Philadelphia: WB Saunders. 1982.
14 Bucholz RW, Burkhead WZ. The pathological anatomy of fatal atlanto-occipital dislocations. J Bone Joint Surg Am. 1979;61:248-250.
15 Page CP, Story JL, Wissinger JP, et al. Traumatic atlantooccipital dislocation. Case report. J Neurosurg. 1973;39:394-397.
16 Gabrielsen TO, Maxwell JA. Traumatic atlanto-occipital dislocation; with case report of a patient who survived. Am J Roentgenol Radium Ther Nucl Med. 1966;97:624-629.
17 Pang D, Wilberger JEJr. Traumatic atlanto-occipital dislocation with survival: case report and review. Neurosurgery. 1980;7:503-508.
18 Woodring JH, Selke ACJr, Duff DE. Traumatic atlantooccipital dislocation with survival. AJR Am J Roentgenol. 1981;137:21-24.
19 Eismont FJ, Bohlman HH. Posterior atlanto-occipital dislocation with fractures of the atlas and odontoid process. J Bone Joint Surg Am. 1978;60:397-399.
20 Levine AM, Edwards CC. Traumatic lesions of the occipitoatlantoaxial complex. Clin Orthop Relat Res. 1989;239:53-68.
21 Steel HH. Anatomical and mechanical considerations of the atlanto-axial articulations. J Bone Joint Surg Am. 1968;50:1481-1482.
22 Bell HS. Paralysis of both arms from injury to the upper portion of the pyramidal decussation: “cruciate paralysis.”. J Neurosurg. 1970;33:376-380.
23 Schneider RC, Cherry G, Pantek H. The syndrome of acute cervical spinal cord injury, with special reference to the mechanisms involved in hyperextension injuries of the cervical spine. J Neurosurg. 1954;11:546-577.
24 Pappas CT, Gibson AS, Sonntag VKH. Decussation of hind-limb and fore-limb fibers in the monkey corticospinal tract: relevance to cruciate paralysis. J Neurosurg. 1991;75:935-940.
25 Nathan PW, Smith MC. Long descending tracts in man. I. Review of present knowledge. Brain. 1955;78:248-303.
26 Alker GJ, Oh YS, Leslie EV, et al. Postmortem radiology of head neck injuries in fatal traffic accidents. Radiology. 1975;114:611-617.
27 Wackenheim A. Roentgen Diagnosis of the Craniovertebral Region. Berlin: Springer-Verlag; 1974.
28 Wholey JH, Bruwer AJ, Baker HL Jr. The lateral roentgenogram of the neck (with comments on the atlanto-odontoid-basion relationship). Radiology. 1958;71:350-356.
29 Lee C, Woodring JH, Goldstein SJ, et al. Evaluation of traumatic atlantooccipital dislocations. AJNR Am J Neuroradiol. 1987;8:19-26.
30 Kaufman RA, Carroll CD, Buncher CR. Atlantooccipital junction: standards for measurement in normal children. AJNR Am J Neuroradiol. 1987;8:995-999.
31 Kaufman RA, Dunbar JS, Botsford JA, et al. Traumatic longitudinal atlanto-occipital distraction injuries in children. AJNR Am J Neuroradiol. 1982;3:415-419.
32 Harris JH, Carson GC, Wagner LK. Radiologic diagnosis of traumatic occipitovertebral dissociation: 1. Normal occipitovertebral relationships on lateral radiographs of supine subjects. AJR Am J Roentgenol. 1994;162:881-886.
33 Harris JH, Carson GC, Wagner LK, et al. Radiologic diagnosis of traumatic occipitovertebral dissociation: 2. Comparison of three methods of detecting occipitovertebral relationships on lateral radiographs of supine subjects. AJR Am J Roentgenol. 1994;162:887-892.
34 Fisher CG, Sun JC, Dvorak M. Recognition and management of atlanto-occipital dislocation: improving survival from an often fatal condition. Can J Surg. 2001;44:412-420.
35 Deliganis AV, Baxter AB, Hanson JA, et al. Radiologic spectrum of craniocervical distraction injuries. Radiographics. 2000;20:S237-S250.
36 Bono CM, Vaccaro AR, Fehlings M, et al. Measurement techniques for upper cervical spine injuries: consensus statement of the spine trauma study group. Spine. 2007;32:593-600.
37 DiBenedetto T, Lee CK. Traumatic atlanto-occipital instability. A case report with follow-up and a new diagnostic technique. Spine. 1990;15:595-597.
38 Gerlock AJJr, Mirfakhraee M, Benzel EC. Computed tomography of traumatic atlantooccipital dislocation. Neurosurgery. 1983;13:316-319.
39 Dziurzynski K, Anderson PA, Bean DB, et al. A blinded assessment of radiographic criteria for atlanto-occipital dislocation. Spine. 2005;30:1427-1432.
40 Rojas CA, Bertozzi JC, Martinez CR, et al. Reassessment of the craniocervical junction: normal values on CT. AJNR Am J Neuroradiol. 2007;28:1819-1821.
41 Przybylski GJ, Clyde BL, Fitz CR. Craniocervical junction subarachnoid hemorrhage associated with atlanto-occipital dislocation. Spine. 1996;21:1761-1768.
42 Pang D, Nemzek WR, Zovickian J. Atlanto-occipital dislocation: part 1—normal occipital condyle-C1 interval in 89 children. Neurosurgery. 2007;61:514-521.
43 Pang D, Nemzek WR, Zovickian J. Atlanto-occipital dislocation—part 2: the clinical use of (occipital) condyle-C1 interval, comparison with other diagnostic methods, and the manifestation, management, and outcome of atlanto-occipital dislocation in children. Neurosurgery. 2007;61:995-1015.
44 Gonzalez LF, Fiorella D, Crawford NR, et al. Vertical atlantoaxial distraction injuries: radiological criteria and clinical implications. J Neurosurg Spine. 2004;3:273-280.
45 Emery SE, Pathri MN, Wilber RG, et al. Magnetic resonance imaging of the post traumatic spinal ligament injury. J Spinal Disord. 1989;2:229-233.
46 Haba H, Taneichi H, Kotani Y, et al. Diagnostic accuracy of magnetic resonance imaging for detecting posterior ligamentous complex injury associated with thoracic and lumbar fractures. J Neurosurg. 2003;99(suppl):20-26.
47 Watridge CB, Orrison WW, Arnold H, et al. Lateral atlantooccipital dislocation: case report. Neurosurgery. 1985;17:345-347.
48 Jevtich V. Traumatic lateral atlanto-occipital dislocation with spontaneous bony fusion. A case report. Spine. 1989;14:123-124.
49 Evarts CM. Traumatic occipito-atlantal dislocation. J Bone Joint Surg Am. 1970;52:1653-1660.
50 Ramsay AH, Waxman BP, O’Brien JF. A case of traumatic atlanto-occipital dislocation with survival. Injury. 1986;17:412-413.
51 Collalto PM, DeMuth WW, Schwentker EP, et al. Traumatic atlanto-occipital dislocation. Case report. J Bone Joint Surg Am. 1986;68:1106-1109.