20 Endovascular Therapies for Cerebral Revascularization
Introduction
Stroke is the third leading cause of death in the United States and the second leading cause of death worldwide. The AHA estimates that the direct and indirect costs of stroke in the United States in 2009 at $69.9 billion.1 Stroke has been recognized as a clinical entity for millennia, dating at least to ancient Greece. Hippocrates first described and documented the syndrome of stroke, which he called “apoplexy” (from the Greek apoplēssein, to strike down and incapacitate). Prior to the advent and widespread accessibility of high-resolution cross-sectional imaging, neurologists and neurosurgeons could only establish the location of strokes on the basis of clinicopathological correlation. Little was known about the pathophysiology of stroke for much of the 20th century, with in situ thrombosis being the presumed etiology for most ischemic strokes.2 The recognition of thromboembolic phenomena to the brain originating from the carotid artery and the description of lacunar syndromes allowed the development of strategies for stroke prevention.3,4 However, therapies for acute stroke were not developed until the latter part of the 20th century.
The first three trials evaluating the use of intravenous streptokinase for the treatment of acute stroke were terminated prematurely due to an increase in mortality rate, primarily related to the hemorrhagic conversion of ischemic infarcts.5,6 The European Cooperative Acute Stroke Study (ECASS),7 which tested recombinant tissue plasminogen activator (r-tPA) at a dose of 1.1 mg/kg, demonstrated no overall benefit to the use of intravenous (IV) r-tPA for the treatment of acute stroke. Subgroup analysis, however, revealed significant improvement after IV t-PA administration in patients with moderate to severe stroke without extended signs of infarction on CT. This study showed the therapeutic potential for thrombolytic agents in the treatment of acute stroke while underscoring the need to refine indications and contraindications for therapy.
The first trial to demonstrate significant clinical benefit for intravenous r-tPA with an acceptable risk of intracranial hemorrhage was the National Institute of Neurological Diseases and Stroke (NINDS) study.8 The NINDS study used a lower dose of intravenous r-tPA (0.9 mg/kg), which was administered within 3 hours of stroke onset. The results of the NINDS study led to the approval of intravenous r-tPA by the United States Food and Drug Administration (FDA) for use in acute stroke within 3 hours of onset of stroke symptoms. The safety and efficacy of the 3-hour therapeutic window was confirmed by the Alteplase Thrombolysis for Acute Noninterventional Therapy in Ischemic Stroke (ATLANTIS) trial.9 The ATLANTIS trial also failed to demonstrate benefit to patients who received r-tPA in a 3- to 5-hour window.
Numerous studies have subsequently demonstrated the efficacy of intravenous r-tPA when given within the 3-hour window.10–12 Although the use of IV r-tPA was initially controversial, it has now been endorsed as class IA level of evidence by the major national guidelines development organizations.13 Pooled results from the six major randomized, placebo-controlled IV r-tPA stroke trials8,9,14 (ATLANTIS I & II, ECASS I & II, and NINDS I & II) included 2775 patients who were treated with IV r-tPA or a placebo. Treatment up to 3 hours after symptom onset benefitted patients, and findings suggested a benefit in treatment beyond 3 hours in certain subsets of patients. The ECASS III trial demonstrated the benefit of IV r-tPA in the 3.5- to 4-hour window in a more select group of patients.15
Recanalization rates, which are related to the diameter of the affected vessel, range from 20% for the ICA to 50% for occlusion of a distal branch of the MCA.16 Ischemic stroke related to large vessel (i.e., >2 mm) occlusion carries a high risk of mortality (53% to 92%) and severe morbidity.17,18 The presence of a hyperdense MCA sign on CT in the presence of a moderate stroke (National Institute of Health Stroke Scale [NIHSS] stroke score of >10) portends a less favorable therapeutic outcome with IV r-tPA. This finding suggests that large proximal clots are resistant to IV r-tPA.19 Endovascular therapies for acute stroke, particularly those related to large vessel occlusion, may overcome some limitations of systemic thrombolysis by providing superior recanalization rates in large vessel occlusions, reducing the incidence of intracranial hemorrhage, and expanding the therapeutic window.
Recanalization strategies
Recanalization aims to restore antegrade blood flow by removing or dissolving occlusive thrombus, thereby allowing reperfusion of the ischemic penumbra, the functionally impaired but still viable brain tissue. Endovascular techniques for recanalization include mechanical thrombectomy, chemical thrombolysis, stenting (either temporary or permanent), or a combination thereof (Figure 20–1).
The rationale for restoring antegrade flow as rapidly as possible after stroke is intuitive. The aphorism “time is brain” accurately reflects the ephemeral nature of the ischemic penumbra and underscores the importance of rapid restoration of blood flow. The ultimate outcome of stroke is not solely dependent on the time of occlusion of the target vessel. It also depends on a number of factors and their complex degree of interactions. These factors include the site of arterial occlusion, the extent of the core of infarction, the size of the ischemic penumbra, the quality and quantity of collateral arterial supply, and the integrity of the blood-brain barrier (BBB). However, on an epidemiological level, a number of studies have documented the correlation between radiographic recanalization and improved clinical outcome as well as the critical role of rapid restoration of flow.20
Intra-Arterial Thrombolysis
Intra-arterial recanalization is occasionally used as a primary treatment modality in patients in whom IV r-tPA is contraindicated or patients who fail to demonstrate clinical improvement after administration of IV r-tPA. Primary intra-arterial thrombolysis can benefit clinical outcomes of patients who receive treatment 3 to 6 hours after symptom onset.21,22 Compared to systemic IV thrombolysis, intra-arterial thrombolysis offers several theoretical advantages. Coaxial microcatheter techniques allow superselective access to the occluded vessel, thereby allowing infusion of thrombolytic agents directly into the thrombus. By infusing thrombolytic agents directly at the site of the thrombus, a smaller dose of fibrinolytic agent can result in more complete recanalization compared to systemic administration. Theoretically, rates of complications from systemic fibrinolysis such as ICH should be reduced.
Merci Retrieval System
The Merci Retrieval System was approved by the FDA in 2004 for the treatment of acute stroke due to thromboembolic occlusion of the intracranial vertebral artery, basilar artery, intracranial ICA, or M1 division of the MCA. The Mechanical Embolus Removal in Cerebral Ischemia (MERCI) trial was a prospective, nonrandomized, single-arm, multicenter trial that investigated the use of a novel mechanical thrombectomy device in patients with occluded large intracranial vessels within 8 hours of the onset of stroke symptoms.23 The primary endpoint of the trial was radiographic recanalization of the target vessel with a low rate of serious adverse events. The trial enrolled 151 patients and demonstrated recanalization rates of 48% with a complication rate of 7.1%. Morbidity and mortality rates were lower in patients who demonstrated radiographic recanalization.
The Merci Retrieval System consists of the Merci Retriever, the Merci Balloon Guide Catheter (BGC), and the Merci microcatheter through which the actual retrieval device is deployed (Figure 20–2). The BGC is a 9-French catheter with a large 2.1-mm lumen and a balloon located at the distal tip. The Merci Retriever itself consists of a tapered wire with five helical loops of decreasing diameter (from 2.8 to 1.1 mm) at the distal end. The loops are constructed from memory-shaped nitinol (nickel titanium) and therefore exploit the superelastic properties of this alloy. Once the microcatheter is advanced distal to the clot, the Merci Retriever is advanced through the microcatheter in its straight configuration. Once deployed, the retriever reforms into its pre-established helical shape thereby ensnaring the thrombus. During this procedure, the balloon at the tip of the BGC (usually located in the common carotid artery or ICA) is inflated to minimize distal flow. A complete description of the technique can be found in the original description of the MERCI Phase I Study.24
On the basis of the results of the MERCI trial, the Merci Retrieval System was approved by the FDA for use in the treatment of acute stroke up to 8 hours after symptom onset. The approval of this device without a randomized trial has drawn some criticism, most notably due to the apparent increase in deaths associated with its use compared to the Prolyse in Acute Cerebral Thromboembolism (PROACT II—an earlier study evaluating the use of intra-arterial prourokinase) from 27% to 44%.25,26 However, the MERCI investigators argued that the presence of a higher percentage of distal ICA and basilar artery occlusions may have biased their results toward worse outcomes. The discrepancies in clinical outcomes underscore the need to accurately identify which patients are most likely to benefit from aggressive intervention.
Penumbra System
The PS is composed of three main components: a reperfusion catheter, a separator, and a thrombus removal ring (Figure 20–3). For aspiration, the reperfusion catheter is used coaxially with the separator while attached to an aspiration (suction) source to separate the thrombus from the vessel wall. If residual thrombus is still present after revascularization with aspiration, the thrombus removal ring can be used to directly engage and remove the residual thrombus. A complete description of the device and its use can be found in the original trial.27
Penumbra System Technical Comments
Early recanalization and reperfusion have been identified as good prognostic indicators in a number of clinical trials. However, the lack of contemporaneous controls in these studies precludes the conclusion that thrombectomy definitively improves stroke outcomes. Randomized controlled trials evaluating the clinical benefit and cost-effectiveness of these invasive treatment modalities will help answer these questions and are underway.28,29 The Phenox CRC Mechanical Thrombectomy Device (Phenox, Bochum, Germany) and the Catch Mechanical Thrombus Retriever (Balt, Montmorency, France) are approved for use in Europe.
Frequently, mechanical thrombectomy is used in conjunction with chemical thrombolysis to restore flow. Adjuvant intra-arterial thrombolysis has been evaluated in Phase I and II trials,30 and randomized trials are ongoing.28
AngioJet Catheter
The AngioJet (Possis Medical, Inc., Minneapolis, MN) thrombectomy catheter uses high-pressure, pulsatile saline to fragment and aspirate clot into the lumen of the catheter. The AngioJet is used primarily in peripheral and interventional cardiac procedures, but successful intracranial use has been reported.31,32 This device could be considered for off-label use in patients with large clot burden.
Stenting for Acute Stroke
Several case reports involving the use of self-expanding stents in the setting of recalcitrant thromboses led to interest in evaluating the use of stents for the treatment of acute stroke. The Stent-Assisted Recanalization in Acute Ischemic Stroke (SARIS) trial was a prospective, single-arm trial to evaluate intracranial stenting as a first-line intra-arterial acute stroke treatment.33 Primary outcome measures were safety, which is defined by the occurrence of symptomatic (>4 point worsening on the NIHSS or neurological decline in the presence of intracranial hemorrhage) or asymptomatic intracranial hemorrhage, or neurological deterioration and evidence of recanalization (evaluated by the TIMI score of the target vessel). In this small investigational study, results were encouraging and the authors recommended further analysis to evaluate the utility of intra-arterial stenting as a primary treatment modality for acute stroke.
Clinical trials
Endovascular Revascularization Techniques for Intracranial Stenosis
Large vessel atherosclerotic disease is a significant cause of intracranial ischemic events, accounting for about 10% of strokes. Important demographic disparities have been identified as well; African Americans, Asians, and Latinos are more likely to suffer from stroke related to intracranial stenosis than Caucasians, who tend to suffer from extracranial stenoses.34
The Warfarin versus Aspirin for Symptomatic Intracranial Disease (WASID) Trial demonstrated that certain subgroups of patients remain at a high risk of developing a stroke despite adequate medical therapy.35 The WASID trial and the smaller Groupe d’ Étude des Sténoses Intra-Crâniennes Athéromateuses symptomatiques (GESICA) both identified subgroups of patients with intracranial stenosis who are at high risk of suffering from stroke. The WASID trial studies found that the 1- and 2-year risk of stroke in patients with 50% to 69% stenosis was 6% and 10%, respectively. However, in patients with 70% to 99% stenosis, the 1- and 2-year risk rose to 18% and 20%, respectively. The GESICA trial did not quantify the degree of stenosis but identified patients with hemodynamic features (i.e., symptoms exacerbated by physical activity, change in body position, or the addition or increase in an antihypertensive medication) that increased the risk of developing recurrent vascular events.
Since its initial description by Dotter and Judkins in 1964, percutaneous transluminal angioplasty (PTA) has been used successfully to treat peripheral and coronary arterial disease.36 Initially, coronary angioplasty balloons were used off-label to perform intracranial PTA alone. As balloon-mounted stents became the mainstay of endoluminal treatment of coronary artery disease, these devices were adopted for the treatment of intracranial atherosclerotic disease. Technological modifications were necessary to allow navigation of the more tortuous intracranial circulation with more flexible catheters and improved stent delivery systems. Initial attempts at treatment of intracranial atherosclerotic disease with stenting resulted in acceptable periprocedural rates of morbidity and mortality, but with relatively high rates of technical failure.
Initial reports of high complication rates (vessel dissection, distal embolization, vasospasm, and arterial thrombosis)37 were probably related to inadequate technology. Angioplasty with modern over-the-wire microcatheter-guided balloons alone appears to compare favorably to medical therapy alone,38 but is associated with higher restenosis rates than modern intracranial stenting (50% compared to 7.5%).39
Complications
Major cerebrovascular complications related to angioplasty and stenting of intracranial vessels are related primarily to vessel rupture and thromboembolic phenomena. The Wingspan Intracranial Stent Registry Study Group identified possible risk factors for major complications due to angioplasty and stenting in patients with posterior circulation stenosis, low-volume centers, stenting within 10 days of a qualifying event, or stroke as a qualifying event.40
Restenosis represents a major source of long-term failure of endoluminal stent therapy. Restenosis occurs as a result of the elastic recoil of the vessel, neointimal hyperplasia, and vascular remodeling. In-stent stenosis occurs in approximately one third of patients who receive the Wingspan stent41 and is most common in the anterior circulation, particularly in the supraclinoid ICA segment.42 Revascularization by angioplasty with or without stenting in patients with restenosis is safe and effective in approximately 50% of patients with in-stent stenosis, although the durability of this therapy is unknown.43
Intracranial Stenting Technical Comments
All patients are placed under general anesthesia for percutaneous transluminal angioplasty and stenting (PTAS) treatment of intracranial stenoses using the Wingspan system (Figure 20–4). Intraoperative neurophysiological monitorings (somatosensory evoked potential and electroencephalography) are used throughout the procedure. Arterial access is typically achieved through the common femoral artery. In rare instances, other arterial sites such as transradial, transbrachial, or direct carotid puncture are required. Heparinization is instituted with the goal of activated coagulation times between 250 and 300 seconds after arterial access is achieved. All interventions are performed through a 6-French system guide catheter.
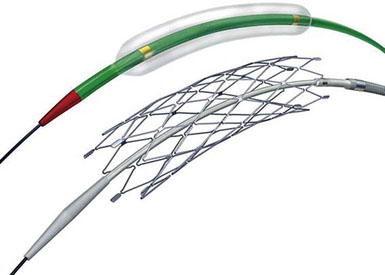
Figure 20–4 The Gateway balloon and Wingspan intracranial stent.
(Used with permission from Boston Scientific.)
Next, the Wingspan delivery system is prepared and advanced over the exchange wire across the target lesion. The stent diameter is sized to exceed the diameter of the normal parent vessel by 0.5 to 1.0 mm. The stent length is selected to equal or exceed the length of the angioplasty balloon. In addition, the stent length is selected to completely cover the entire diseased segment and to allow the proximal end of the stent to be positioned so as not to preclude future endovascular access into the stented segment. The diameter of the stenotic lesion is measured using biplanar angiography and compared with a reference diameter of the normal vessel (usually proximal to the lesion), according to the technique used in the WASID study. Angiography is performed after the stent is deployed (Figure 20–5). Post-stenting angioplasty may be required if residual stenosis is present. Heparinization is typically neither reversed nor continued postoperatively.
Extracranial carotid stenosis
Occlusive disease of the extracranial ICA is assumed to be the cause of one-fourth of all strokes.44 Based on numerous randomized trials, carotid artery endarterectomy (CEA) reduces the risk of stroke in patients with moderate (>50%) symptomatic or severe (>60%) asymptomatic carotid stenosis.45,46 CEA is considered to be the best surgical treatment for carotid atherosclerotic disease, and CEA has subsequently become one of the most commonly performed surgical procedures in the United States. Certain groups of patients, however, are at high risk for complications related to CEA. Anatomic considerations (high carotid bifurcations, prior CEA, radiation-induced stenosis) can increase the technical difficulty of the operation. Patients with a history of myocardial infarction, angina, or hypertension are at increased risk of sustaining procedure-related complications. The benefits of CEA are lost if the 30-day rate of stroke or death exceeds 6% for symptomatic stenosis or 3% for asymptomatic stenosis.
Carotid artery stenting had emerged as a less invasive and effective alternative to CEA. Several randomized controlled trials comparing CEA with carotid artery stenting have yielded conflicting results. While some studies have demonstrated that carotid artery stenting was not inferior to CEA,47,48 others have concluded that carotid artery stenting was inferior to CEA.49 Meta-analysis and pooled risk estimates resulted in wide confidence intervals, making generalizations based on these studies very difficult.
Strong evidence recommends carotid artery stenting for treating specific subsets of patients, including restenosis after CEA, radiation-induced stenosis, those with anatomically high lesions, and those at high risk for undergoing general anesthesia.50,51
Extracranial Carotid Stenting Technical Comments
All carotid artery stenting performed at our practice is executed on a monorail system with a distal protection device (DPD) (Figure 20–6). The monorail guidewire with the DPD is navigated gently across the stenosis. The DPD is deployed in the distal ICA but may require more proximal deployment if the vessel is extremely tortuous to navigate. Prestenting angioplasty with an undersize, noncompliant balloon may be required if the lesion is severely stenotic. Before angioplasty, we notify the anesthesiologist of potential vasovagal response associated with angioplasty. The vasovagal response can be dramatic, sometimes resulting in hemodynamically significant bradycardia or even asystole.52 Occasionally, the anesthesiologist may elect to pretreat the patient with an anticholinergic agent to blunt the vasovagal reflex.
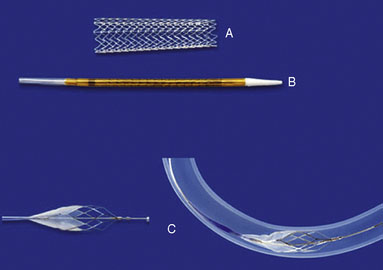
Figure 20–6 The Acculink stent (A), stent delivery device (B), and the Accunet embolic protection device (C).
(Used with permission from Abbott Vascular.)
The balloon is inflated slowly with pure contrast for maximal visualization and subsequently deflated after reaching the desired inflation (6 to 10 atmospheres). A carotid angiogram is then obtained to ensure the dilation is sufficient to pass the stent. A self-expandable stent is navigated over the targeted lesion length. Using the cervical bony landmarks on a lateral angiography, the stent is deployed using a left-to-right unsheathing technique over the stent stabilizer. Poststent angioplasty may be required if residual stenosis is present. A noncompliant balloon that is approximately the diameter of the vessel is inflated slowly and under continuous fluoroscopic visualization for poststenting dilatation. An angiogram is then obtained after poststent dilatation. The DPD is recaptured with a retrieval device. Extracranial and intracranial carotid angiography is performed to evaluate for potential thromboembolic complications (Figure 20–7). In most cases, heparinization is neither reversed nor continued postoperatively.
1 Heart Disease and Stroke Statistics. American Heart Association, 2009. Available at: www.americanheart.org/presenter.jhtml?identifier=1200026
2 Caplan L.R. Stroke: A Clinical Approach. Boston: Butterworth-Heinemann, 1993.
3 Fisher M. Occlusion of the internal carotid artery. AMA Arch Neurol Psychiatry. 1951;65:346-377.
4 Fisher C.M. Lacunes: Small, deep cerebral infarcts. Neurology. 1965;15:774-784.
5 Hommel M., Boissel J.P., Cornu C., et al. Termination of trial of streptokinase in severe acute ischaemic stroke. MAST Study Group. Lancet. 1995;345:57.
6 Thrombolytic therapy with streptokinase in acute ischemic stroke: The Multicenter Acute Stroke Trial-Europe Study Group. N Engl J Med. 1996;335:145-150.
7 Hacke W., Kaste M., Fieschi C., et al. Intravenous thrombolysis with recombinant tissue plasminogen activator for acute hemispheric stroke. The European Cooperative Acute Stroke Study (ECASS). JAMA. 1995;274:1017-1025.
8 Tissue plasminogen activator for acute ischemic stroke: The National Institute of Neurological Disorders and Stroke rt-PA Stroke Study Group. N Engl J Med. 1995;333:1581-1587.
9 Clark W.M., Wissman S., Albers G.W., et al. Recombinant tissue-type plasminogen activator (Alteplase) for ischemic stroke 3 to 5 hours after symptom onset. The ATLANTIS Study: a randomized controlled trial. Alteplase Thrombolysis for Acute Noninterventional Therapy in Ischemic Stroke. JAMA. 1999;282:2019-2026.
10 Albers G.W., Bates V.E., Clark W.M., et al. Intravenous tissue-type plasminogen activator for treatment of acute stroke: the Standard Treatment with Alteplase to Reverse Stroke (STARS) study. JAMA. 2000;283:1145-1150.
11 Asimos A.W., Norton H.J., Price M.F., et al. Therapeutic yield and outcomes of a community teaching hospital code stroke protocol. Acad Emerg Med. 2004;11:361-370.
12 Dick A.P., Straka J. IV tPA for acute ischemic stroke: results of the first 101 patients in a community practice. Neurologist. 2005;11:305-308.
13 Adams H.P.Jr, Brott T.G., Furlan A.J., et al. Guidelines for thrombolytic therapy for acute stroke: a supplement to the guidelines for the management of patients with acute ischemic stroke. A statement for healthcare professionals from a Special Writing Group of the Stroke Council, American Heart Association. Circulation. 1996;94:1167-1174.
14 Hacke W., Donnan G., Fieschi C., et al. Association of outcome with early stroke treatment: pooled analysis of ATLANTIS, ECASS, and NINDS rt-PA stroke trials. Lancet. 2004;363(9411):768-774.
15 Hacke W., Kaste M., Bluhmki E., et al. Thrombolysis with alteplase 3 to 4.5 hours after acute ischemic stroke. N Engl J Med. 2008;359:1317-1329.
16 Wolpert S.M., Bruckmann H., Greenlee R., et al. Neuroradiologic evaluation of patients with acute stroke treated with recombinant tissue plasminogen activator. The rt-PA Acute Stroke Study Group. AJNR Am J Neuroradiol. 1993;14:3-13.
17 Brandt T., von K.R., Muller-Kuppers M., et al. Thrombolytic therapy of acute basilar artery occlusion. Variables affecting recanalization and outcome. Stroke. 1996;27:875-881.
18 Bruckmann H., Ferbert A., del Zoppo G.J., et al. Acute vertebral-basilar thrombosis. Angiologic-clinical comparison and therapeutic implications. Acta Radiol Suppl. 1986;369:38-42.
19 Tomsick T., Brott T., Barsan W., et al. Prognostic value of the hyperdense middle cerebral artery sign and stroke scale score before ultraearly thrombolytic therapy. AJNR Am J Neuroradiol. 1996;17:79-85.
20 Gado-Mederos R., Rovira A., Varez-Sabin J., et al. Speed of tPA-induced clot lysis predicts DWI lesion evolution in acute stroke. Stroke. 2007;38:955-960.
21 Furlan A., Higashida R., Wechsler L., et al. Intra-arterial prourokinase for acute ischemic stroke. The PROACT II study: a randomized controlled trial. Prolyse in Acute Cerebral Thromboembolism. JAMA. 1999;282:2003-2011.
22 Ogawa A., Mori E., Minematsu K., et al. Randomized trial of intraarterial infusion of urokinase within 6 hours of middle cerebral artery stroke: the middle cerebral artery embolism local fibrinolytic intervention trial (MELT) Japan. Stroke. 2007;38:2633-2639.
23 Smith W.S., Sung G., Starkman S., et al. Safety and efficacy of mechanical embolectomy in acute ischemic stroke: results of the MERCI trial. Stroke. 2005;36:1432-1438.
24 Gobin Y.P., Starkman S., Duckwiler G.R., et al. MERCI 1: a phase 1 study of Mechanical Embolus Removal in Cerebral Ischemia. Stroke. 2004;35:2848-2854.
25 Becker K.J., Brott T.G. Approval of the MERCI clot retriever: a critical view. Stroke. 2005;36:400-403.
26 Wechsler L.R. Does the Merci Retriever work? Against. Stroke. 2006;37:1341-1342.
27 Bose A., Henkes H., Alfke K., et al. The Penumbra System: a mechanical device for the treatment of acute stroke due to thromboembolism. AJNR Am J Neuroradiol. 2008;29:1409-1413.
28 Broderick J.P., Tomsick T.A. Interventional Management of Stroke (IMS) III Trial. Clinical Trials.gov, 2008. Available at www.clinicaltrials.gov
29 Patil C.G., Long E.F., Lansberg M.G. Cost-effectiveness analysis of mechanical thrombectomy in acute ischemic stroke. J Neurosurg. 2009;110:508-513.
30 The Interventional Management of Stroke (IMS) II Study. Stroke. 2007;38:2127-2135.
31 Bellon R.J., Putman C.M., Budzik R.F., et al. Rheolytic thrombectomy of the occluded internal carotid artery in the setting of acute ischemic stroke. AJNR Am J Neuroradiol. 2001;22:526-530.
32 Mayer T.E., Hamann G.F., Schulte-Altedorneburg G., et al. Treatment of vertebrobasilar occlusion by using a coronary waterjet thrombectomy device: a pilot study. AJNR Am J Neuroradiol. 2005;26:1389-1394.
33 Levy E.I., Siddiqui A.H., Crumlish A., et al. First Food and Drug Administration–approved prospective trial of primary intracranial stenting for acute stroke: SARIS (stent-assisted recanalization in acute ischemic stroke). Stroke. 2009;40:3552-3556.
34 Sacco R.L., Kargman D.E., Gu Q., et al. Race-ethnicity and determinants of intracranial atherosclerotic cerebral infarction. The Northern Manhattan Stroke Study. Stroke. 1995;26:14-20.
35 Chimowitz M.I., Lynn M.J., Howlett-Smith H., et al. Comparison of warfarin and aspirin for symptomatic intracranial arterial stenosis. N Engl J Med. 2005;352:1305-1316.
36 Dotter C.T., Judkins M.T. Transluminal treatment of arteriosclerotic obstruction. Description of a new technic and a preliminary report of its application. Circulation. 1964;30:654-670.
37 Alazzaz A., Thornton J., Aletich V.A., et al. Intracranial percutaneous transluminal angioplasty for arteriosclerotic stenosis. Arch Neurol. 2000;57:1625-1630.
38 Marks M.P., Wojak J.C., Al-Ali F., et al. Angioplasty for symptomatic intracranial stenosis: clinical outcome. Stroke. 2006;37:1016-1020.
39 Mazighi M., Yadav J.S., Bou-Chebl A. Durability of endovascular therapy for symptomatic intracranial atherosclerosis. Stroke. 2008;39:1766-1769.
40 Nahab F., Lynn M.J., Kasner S.E., et al. Risk factors associated with major cerebrovascular complications after intracranial stenting. Neurology. 2009;72:2014-2019.
41 Levy E.I., Turk A.S., Albuquerque F.C., et al. Wingspan in-stent restenosis and thrombosis: incidence, clinical presentation, and management. Neurosurgery. 2007;61:644-650.
42 Turk A.S., Levy E.I., Albuquerque F.C., et al. Influence of patient age and stenosis location on wingspan in-stent restenosis. AJNR Am J Neuroradiol. 2008;29:23-27.
43 Fiorella D.J., Levy E.I., Turk A.S., et al. Target lesion revascularization after wingspan: assessment of safety and durability. Stroke. 2009;40:106-110.
44 Dyken M.L. Prevention of Stroke. New York: Springer-Verlag, 1991.
45 Barnett H.J., Taylor D.W., Eliasziw M., et al. Benefit of carotid endarterectomy in patients with symptomatic moderate or severe stenosis. North American Symptomatic Carotid Endarterectomy Trial Collaborators. N Engl J Med. 1998;339:1415-1425.
46 Halliday A., Mansfield A., Marro J., et al. Prevention of disabling and fatal strokes by successful carotid endarterectomy in patients without recent neurological symptoms: randomised controlled trial. Lancet. 2004;363:1491-1502.
47 Endovascular versus surgical treatment in patients with carotid stenosis in the Carotid and Vertebral Artery Transluminal Angioplasty Study (CAVATAS): a randomised trial. Lancet. 2001;357:1729-1737.
48 Yadav J.S., Wholey M.H., Kuntz R.E., et al. Protected carotid-artery stenting versus endarterectomy in high-risk patients. N Engl J Med. 2004;351:1493-1501.
49 Janjigian M.P., Shah N.R. Endarterectomy is superior to stenting in patients with symptomatic severe carotid stenosis. J Clin Outcomes Mgt. 2007;14:89-92.
50 Hobson R.W., Mackey W.C., Ascher E., et al. Management of atherosclerotic carotid artery disease: clinical practice guidelines of the Society for Vascular Surgery. J Vasc Surg. 2008;48:480-486.
51 Veith F.J., Amor M., Ohki T., et al. Current status of carotid bifurcation angioplasty and stenting based on a consensus of opinion leaders. J Vasc Surg. 2001;33(Suppl 2):S111-S116.
52 Criado E., Doblas M., Fontcuberta J., et al. Carotid angioplasty with internal carotid artery flow reversal is well tolerated in the awake patient. J Vasc Surg. 2004;40:92-97.