CHAPTER 376 Endovascular Stenting of Intracranial Aneurysms
Overview: Conceptual Evolution and History of Intracranial Stenting for Aneurysms
The impetus for stent-supported aneurysm embolization was the recognition that many wide-necked aneurysms were not amenable to endovascular treatment simply because embolization coils could not be reliably retained within the aneurysm fundus. Although many of these lesions could be successfully treated using a balloon-remodeling technique,1,2 this approach was perceived to be technically demanding, risky, and without an adequate “bail-out” strategy should the coils begin to prolapse into the parent artery during treatment. Intracranial stenting can overcome many of these limitations. These devices can be placed into the parent arteries to provide a durable mesh barrier across the aneurysm neck, which prevents coils from prolapsing out of the aneurysm after detachment.
Initial Applications of Stents for Aneurysm Therapy
Early Experience with Balloon-Expandable Stents
Several years later, Higashida and associates6 described the first successful transstent coiling of a circumferential, fusiform basilar artery aneurysm that could not be coiled using conventional techniques. During the ensuing 5 years, several additional case reports and small case series appeared in the literature describing the off-label applications of balloon-mounted coronary stents (BMCSs) to support the coiling of wide-necked intracranial aneurysms.7–13 During this initial era, stent-supported aneurysm treatment was greatly limited by the rigid nature of the available balloon-expandable coronary stents and the subsequent challenges involved with the delivery and deployment of these devices within the tortuous cerebrovasculature.
Self-Expanding Intracranial Microstents
In 2003, Neuroform (Boston Scientific, Fremont, CA), the first intracranial, microcatheter-delivered, self-expanding nitinol microstent was released in the United States for use in treating wide-necked aneurysms under a Humanitarian Device Exemption. Neuroform and its delivery system are far more flexible than the predicated BMCSs. These characteristics greatly facilitated delivery and deployment within tortuous segments of the cerebrovasculature.14 In 2007, Enterprise (Cordis Neurovascular/Johnson and Johnson, Warren, NJ) was introduced in the United States, providing a second self-expanding intracranial microstent (SEIM) for the treatment of intracranial aneurysms. The Enterprise stent presents several benefits over Neuroform, including ability to be reconstrained, a lower-profile delivery system, and a technically less-complicated deployment mechanism. The introduction of these devices led to a marked increase in the number of stent-assisted aneurysm treatments performed and greatly broadened the scope of lesions amenable to endovascular therapy. As practitioners gained experience with SEIMs, novel approaches to more complex lesions were innovated and the sophistication of endovascular reconstruction increased.15–22 During the past decade, stenting has become a standard adjunctive technique used to facilitate the treatment of wide-necked and complex aneurysms.
Parent Vessel Remodeling
Experimental and Histopathologic Evidence
Given the relative flexibility and low metal surface area coverage (e.g., Neuroform, 6% to 9.5%) of the commercially available SEIMs, the ability of these devices to produce “physiologically significant” parent vessel remodeling could be questioned. Canton and associates23,24 performed a series of experiments to assess the impact of the Neuroform stent on intra-aneurysmal flow. These authors demonstrated that two stents placed in a Y configuration reduced the velocity of the inflow jet by 11% and reduced residual intra-aneurysmal vorticity and shear stress by more than 40%. With respect to biologic remodeling, only a single case is available in the literature describing the histopathologic appearance of an implanted Neuroform stent at autopsy.25 The aneurysm in this case was treated solely by stenting because subsequent attempts to place coils were unsuccessful. After this patient died of unrelated causes 4 months later, an evaluation of the explanted aneurysm demonstrated de novo fibroelastic tissue growing across the aneurysm neck and moderate intimal thickening along the stented segment of the parent vessel.
Clinical Evidence
The available data from clinical case series have provided some additional, albeit preliminary, evidence that stenting may improve the durability of endovascular aneurysm therapy. Aneurysms treated with stents are wide necked, typically larger, and often in a terminal location. These characteristics would predict a very high rate of recurrence with standard coiling techniques. Evaluation of mid- and long-term follow-up results from the collaborative Barrow Neurological Institute–Cleveland Clinic database demonstrated a surprising level of long-term durability in aneurysms treated with stent-support.14,26,26a At an average follow-up of 12.9 months, 72% of aneurysms demonstrated either stability or progressive thrombosis (Fig. 376-4). Of the 28% aneurysms that demonstrated recanalization, defined as any amount of increased filling in comparison to the immediate postcoiling result, many were either very large or giant aneurysms. Small (<10 mm) aneurysms actually recanalized at a very low rate (9.3%), with only 3.1% requiring retreatment.
Several investigators have reported success with stents alone for the treatment of small dissecting and blood blister–like aneurysms, providing additional evidence in support of “physiologically significant” stent-induced remodeling of the parent vessel–aneurysm complex after self-expanding stent implantation.27–33 In the largest series, 10 patients with these types of aneurysms were treated with the Neuroform.29 Nine patients demonstrated interval regression or complete resolution after stent monotherapy (Fig. 376-5), and one was stable after only 1 month of follow-up. No patient experienced aneurysm rerupture during the follow-up period.
The observation of in-stent stenosis (ISS) (Fig. 376-6) in about 5% of patients after Neuroform placement (either as the sole or an adjunctive therapy) further suggests that these devices have the capability to stimulate a significant biologic response after implantation.34 Although the nature of the material accounting for the stenosis observed in these patients remains unknown, the angiographic appearance and time of onset are compatible with the intimal hyperplasia that has been encountered in other vascular beds after stenting for atherostenosis.34
High Metal Surface Area Stents (Flow Diverters)
The concept of parent vessel reconstruction is quickly advancing with the recent development of dedicated flow-diverting endovascular constructs designed for intracranial use. These devices primarily target parent vessel reconstruction, rather than endosaccular occlusion, as the means by which to achieve definitive aneurysm treatment. Currently, these flow-diverting devices are high metal surface area coverage, stent-like constructs (Fig. 376-7) that are designed to provide enough flow redirection and endovascular remodeling to induce aneurysm thrombosis without the use of additional endosaccular occlusive devices (i.e., coils). At the same time, the pore size of the constructs is large enough to allow for the continued perfusion of branch vessels and perforators arising from the reconstructed segment of the parent vessel.35
Commercially Available Devices
Balloon-Expandable Stents
Device Delivery and Deployment
The BMCSs consist of an angioplasty balloon catheter on which a collapsed (but expandable) cylindrical metal cage is mounted. The balloon catheter on which the device is mounted may either be designed for over-the-wire delivery, whereby the microwire exits the proximal hub of the delivery catheter, or monorail delivery, whereby the microwire exits the side wall of the distal aspect of the catheter. Over-the-wire delivery allows for the transmission of greater forward pressure from the proximal microcatheter to the distal aspect. The monorail delivery provides a system that is, in general, easier to use, particularly for a single operator. In most cases, access across the targeted landing zone for stent deployment is achieved with a standard microcatheter and 0.014-inch microwire combination under high-magnification fluoroscopic roadmap control. The microcatheter is manipulated well beyond the targeted landing zone into the distal intracranial vasculature and the microwire is removed. An injection of contrast material through the microcatheter or guiding catheter can be performed to verify an adequate intravascular position of the microcatheter. An exchange-length, 300-cm, 0.014-inch microwire is then placed through the microcatheter, which is then removed. The BMCS is then placed over the microwire and manipulated over this wire and across the targeted landing zone. When the stent is in adequate position, an angiographic run may be performed to verify optimal positioning and to assess for any complications of stent navigation or wire manipulation. When the operator is satisfied with the stent positioning, the stent is deployed through balloon inflation. Inflation of the angioplasty balloon is achieved using a calibrated insufflator. As contrast material is introduced into the balloon, the balloon expands to dilate the stent into the form of a rigid cylinder that is pressed into the adjacent vessel wall (Fig. 376-8). The balloon catheter can then be completely deflated and removed, leaving the deployed stent behind within the artery. At this point, stent-supported aneurysm coiling can be performed.
Device Characteristics
A newer generation of balloon-mounted stents are being designed for intracranial use. These devices have been engineered to be more deliverable and less traumatic than the predicate devices designed for coronary applications. These devices may have indications for the treatment of both cerebral aneurysms and intracranial atherosclerosis.36–38
Current Applications
Clinical Experience
Because of issues with delivery and deployment, most reported experiences with balloon-expandable stents have been limited to relatively small series.7,8,11,39,40 Even the highest-volume centers performed only small numbers of aneurysm embolizations using these devices during the late 1990s and into the next decade. Han and associates7 reported technical success in 10 of 13 patients undergoing attempted coronary stent–assisted aneurysm treatment over 8 years at the Barrow Neurological Institute. In the three cases in which attempted stent placement failed, the stent could not be navigated through the tortuous anatomy of the carotid siphon. Two patients in this series sustained permanent neurological injury as a result of attempted stent placement. Aneurysm recurrence was noted in two of the five patients in this series for whom angiographic follow-up was available.
Lylyk and colleagues31 reported the largest series of BMCS used for cerebral aneurysm treatment, composed of 62 wide-necked saccular aneurysms and 10 dissecting or fusiform aneurysms. By effectively selecting cases, these operators were able to place these balloon-mounted stents with a technical success rate of 90%. At the 3- to 6-month follow-up evaluation, these authors observed a 92% rate of complete or near-complete occlusion for saccular aneurysms and a 100% rate of complete or near-complete occlusion for fusiform aneurysms. Incidentally, this series included 13 aneurysms that were treated with stents alone, of which 5 progressed to complete thrombosis without coiling, demonstrating the effectiveness of endovascular remodeling in achieving aneurysm occlusion even without endosaccular embolization coils.
Self-Expanding Intracranial Microstents
Neuroform
Stent delivery is achieved in a manner similar to that of the BMCS. Access into the cerebrovasculature distal to the targeted landing zone is achieved with a standard microcatheter and 0.014-inch microwire. The microcatheter is then removed over a floppy 300-cm, 0.014-inch wire (e.g., Transcend, Boston Scientific, Fremont, CA; or Xcelerator, ev3 Endovascular, Irvine, CA), which maintains access within the distal vasculature. The entire Neuroform delivery apparatus is then navigated over the exchange microwire to the targeted landing zone within the intracranial vasculature. Stent deployment is achieved by fixing the stabilizer in position and withdrawing the microcatheter to release the stent, which expands to its preset cylindrical morphology (Video 376-1, Part 1).
The devices come in sizes ranging between 2.5 and 4.5 mm in diameter and 10 and 30 mm in length. The recommended diameter for placement is 0.5 mm greater than the largest diameter of the parent artery to be stented. The length is chosen such that the stent extends for at least 5 mm proximal and distal to the aneurysm neck. The struts composing the stent measure about 60 µm in thickness. The cell size is large enough to accommodate the passage of a 2F microcatheter through the interstices, allowing trans-stent coiling of the aneurysm after placement. The ends of the stent are demarcated by four radiopaque markers (Fig. 376-9). The body of the stent is essentially radiolucent using standard fluoroscopy. After stent deployment, the operator is left to intuit the position and configuration of the stent by approximating a cylindrical construct within the confines of the vascular anatomy. Often, resistance is felt when traversing the stent with the microcatheter, leaving the operator to perform the trans-stent catheterization primarily by feel.
The stent structure is so delicate that individual cells can sometimes be disrupted or displaced during microcatheter traversal. In addition, the chronic outward radial force holding the stent in position is sometimes inadequate to prevent migration during microcatheter traversal of the device. When the stent is placed in curved anatomy, the stent cells are prone to opening, producing gaps in stent coverage along the outer curvature of the vessel.41,42 These gaps can conceivably lead to incomplete coverage of the aneurysm neck and coil prolapse from the aneurysm into the parent artery during embolization.
Enterprise Vascular Reconstruction Device and Delivery System
The Enterprise measures 4.5 mm in diameter when unconstrained and as such is only indicated for use in vessels measuring between 2.5 and 4 mm in diameter. The device comes in 14-, 22-, 28-, and 37-mm lengths. The struts of the Enterprise, like those of the Neuroform, are about 60 µm thick. The stent ends are demarcated by four radiopaque markers; the cage portion of the stent is also entirely radiolucent. Enterprise is a closed-cell stent. This provides several key advantages. First, before full deployment, the device is reconstrainable—if the operator judges the deployment of the stent to have started in a suboptimal position, the stent can be reconstrained and repositioned. Second, the closed-cell design prevents the stent from splaying open along the outer curvature of vascular bends. Third, the closed-cell design results in the incorporation of each cell into the entire device structure, making the individual cells more durable and less likely to become damaged during attempted traversal. At the same time, the loss of segmental flexibility created by the continuous closed-cell structure may result in the device kinking or forming a “cobra head” configuration around tight vascular curves (Fig. 376-10), potentially resulting in poor vessel wall apposition and suboptimal parent vessel protection.15,41 The interstices between stent struts are also smaller and less deformable in some anatomic configurations, making the traversal of the device with a microcatheter more difficult in some situations. Finally, although the closed-cell structure provides higher radial resistive force (i.e., resistance to outward compression once deployed), it also exerts less chronic outward force (i.e., outward pressure on the vessel wall), potentially making it more prone to migration during attempted catheterization of the aneurysm or, in some anatomic configurations, spontaneously.43
Current Indications
The SEIMs have become incorporated into routine clinical practice for the treatment of wide-necked intracranial aneurysms. The availability of these devices has increased the scope of lesions amenable to endovascular therapies.14,44 As they have become available, an increasing number of different applications of these devices have been innovated.
Stent-Assisted Coil Embolization
There are two general approaches to standard stent-assisted coil embolization—trans-stent coiling and “jailing”45 (Video 376-1, Part 2).
Rescue and Salvage
During coil embolization, it is possible for detached coils or the entire coil mass to become inadvertently displaced from the aneurysm into the parent artery. In these cases, a SEIM can be navigated across the herniated coil and deployed to either displace the coil mass back into the aneurysm fundus or tack up the displaced strands of coil against the vessel wall to prevent further migration and decrease their intravascular surface area available to function as a source of distal emboli44,46 (Fig. 376-11).
Balloon-Assisted Coiling Followed by Stenting
Some operators prefer to begin the coiling of wide-necked aneurysms using a balloon-assisted technique, with stent placement occurring later during the case. In these cases, the stent is placed after coiling to stabilize the coil mass and avoid delayed migration of coils into the parent vessel, to act as a scaffolding within the parent artery to facilitate endovascular remodeling of the aneurysm neck, and potentially to induce some degree of flow redirection (Fig. 376-12).
Advanced Techniques
Y Stent
In some cases, a single stent is insufficient to provide parent vessel protection for coil embolization. This situation is most commonly encountered with bifurcation aneurysms arising from the basilar apex or carotid terminus. In these instances, two stents can be placed, the first extending out one limb of the bifurcation and the second introduced through the interstices of the first stent, extending into the other limb of the bifurcation. This configuration forms a Y-shaped construct at the bifurcation and provides robust support for the coil embolization of terminal aneurysms.16,21,22 This Y-stent technique has also been applied to treat middle cerebral artery aneurysms21 and anterior communicating artery aneurysms (Henry H. Woo MD, personal communication, 2006) (Figs. 376-13 and 376-14).
Stenting across the Circle of Willis
In some cases, the creation of a Y construct is challenging or impossible owing to the anatomy of the vessels as they arise from the distal bifurcation. In these cases, it is sometimes possible to accomplish a reconstruction of a terminal bifurcation aneurysm by placing a stent across the circle of Willis—such as from P1 to P1 through the posterior communicating artery, from A1 to M1 through the anterior communicating artery, or from the ipsilateral A1 to contralateral A1 through the anterior communicating artery.19 This technique provides a means by which to achieve protection of both limbs of the bifurcation with a single SEIM (Fig. 376-15).
Waffle-Cone Technique
Rarely, Y-stent reconstruction and stenting across the circle of Willis are not technically feasible for the treatment of a wide-necked terminal aneurysm. In these situations, some investigators have deployed a stent from the parent artery directly into the aneurysm (i.e., “intra-extra-aneurysmal stent placement”) to achieve parent artery protection. Using this technique, a single stent can be used to stabilize an intra-aneurysmal coil mass.47 However, the final construct sets up a vector of flow redirection directly into the terminal aneurysm sac and actually disrupts flow into the bifurcation branches. One might expect that such a construct could lead to high rates of recanalization. In addition, if this technique fails or leads to recanalization, other available means of treatment (surgical clipping, endovascular therapy with balloon remodeling, or Y-stent reconstruction) are made considerably more difficult, if not impossible.
Balloon-in-Stent Technique
Very wide-necked, circumferential aneurysms represent the most challenging lesions to treat using constructive endovascular techniques. In these cases, the goal of therapy is to essentially reconstruct a de novo parent vessel through the center of the aneurysm using one or more stents and coils. This de novo vessel can often become impossible to visualize, being at least partially obscured from all vantage points by either contrast material or embolization coils within the aneurysm. In these cases, a balloon-in-stent technique has been used to facilitate therapy.18 Following the placement of one or more stents across the aneurysmal segment of the vessel, a balloon catheter is placed within the reconstructed parent vessel, and one or more microcatheters are placed within the aneurysm. With the balloon inflated, the image intensifiers can be oriented to best advantage to separate the saccular component of the aneurysm (demarcated by the embolization coils) from the reconstructed parent vessel (demarcated by the inflated balloon). In the other projection, it is useful to try and achieve a “down-the-barrel” view of the inflated balloon within the de novo stent-reconstructed vessel that depicts the balloon centrally surrounded circumferentially by the coil mass.18
Although this technique can be achieved with the SEIMs, it works best when the BMCS are used primarily to reconstruct the de novo vessel. The SEIMs tend to overexpand into the saccular component of the aneurysm, and it can become challenging to ascertain whether the embolization coils are being placed within the parent artery or within the saccular component of the aneurysm. The balloon deflation test (deflating the balloon on a blank roadmap to observe for coil migration into the parent artery before detachment) can be helpful in making this determination; however, it becomes less reliable as the aneurysm becomes more densely packed with coils and the parent artery becomes increasingly obscured. In addition, the SEIMs are easily damaged and displaced; as such, they can easily migrate distally or proximally during the multiple balloon manipulations and inflations that occur during the course of the embolization. When coiling these wide-necked and circumferential aneurysms, it is entirely possible that such migration can result in displacement of one end of the stent into the saccular component of the aneurysm. The BMCSs provide a more rigid, durable construct that is less prone to either of these problems. Fortunately, the development of flow-diverting devices will likely obviate the need for these types of complex reconstructive endovascular treatments, providing a more straightforward, technically easier, and more definitive option (see later) (Fig. 376-16).
Stent Monotherapy
Occasionally, small, dissecting, blood blister–like pseudoaneurysms that are not amenable to either coil embolization or surgical clipping can be effectively treated with one or sometimes multiple overlapping SEIMs, without embolization coils.29 The application of one or more telescoping or overlapping SEIMs represents an attempt to elicit endovascular reconstruction of the parent artery by repairing continuity to the dissected or damaged segment, by diverting flow away from the aneurysmal segment and along the course of the normal parent artery, and ultimately by providing a scaffolding on which subsequent endothelial and neointimal overgrowth can occur to create a fully healed and remodeled parent artery.
The balloon-expandable stents provide a greater degree of metal surface area coverage and may be more effective in diverting flow, redirecting arterial shear forces by changing the configuration of the native artery and ultimately providing scaffolding for tissue overgrowth. However, the trauma induced during the manipulation of these rigid coronary devices to the targeted landing zone and the relatively high-pressure balloon inflation required for their deployment risks perforation of the fragile intracranial vessels that typically give rise to these dissecting and blood blister–like aneurysms. The previously mentioned Pharos balloon-expandable stent has been used as monotherapy for these fusiform and dissecting aneurysms with some success.38 Although this device is not yet available within the United States, it is possible that a balloon-expandable stent specifically engineered to possess the flexibility to be delivered within the cerebral vasculature and to deploy with a minimal amount of vascular trauma could represent a feasible option for these types of lesions. Again, the development of dedicated flow-diverting devices will likely obviate the need to attempt to treat these types of lesions using the available SEIMs or emerging balloon-expandable stent technology (Fig. 376-17; see Fig. 376-5).
Covered Stents
The first successful cases in which a covered stent was applied for the treatment of intracranial aneurysms were reported by Chiaradio and colleagues48 and Islak and associates49 in 2002. Saatci and coworkers50 later reported a series of 24 patients with 25 ICA aneurysms treated with the JoStent coronary stent graft. Twenty-one of the 25 aneurysms in this series involved the extradural carotid artery. All patients were treated without complications, and of the 21 patients with angiographic follow-up, all showed complete exclusion of the aneurysms. Occasionally in this series, the initial stent deployment did not lead to aneurysm obliteration owing to leakage of blood around the outside of the stent and into the aneurysm. However, in most cases, this “endoleak” phenomenon could typically be resolved with additional angioplasty to achieve better apposition of the stent and completely exclude the aneurysm.
Newer generations of balloon-expandable covered, partially covered, and semiporous covered stents are under development, with modifications designed to overcome the aforementioned limitations of the predicate devices. The Willis covered stent (Micro-port, Shanghai, China) is built on a cobalt chromium platform that incorporates a very thin layer of PTFE into its structure, designed to maximize its flexibility and deliverability. The limited clinical data available for the use of this stent in humans has been encouraging.51–53 The endovascular clip system (eCLIPs) is a partially covered, balloon-expandable stent that is designed such that the covered portion can be oriented to selectively cover an aneurysm neck54 while leaving the remainder of the endoluminal surface of the parent artery uncovered, thus potentially allowing continued perfusion of regional perforators while occluding the aneurysm.
Pharmacology
Platelet Inhibition
When placing any type of intracranial stent or stent-like device, dual antiplatelet therapy is an absolute requirement. Placement of a Neuroform in the absence of adequate platelet inhibition is associated with a risk for thromboembolic complications that approaches 50%.44 This requirement for dual antiplatelet medications has essentially limited the use of these devices (almost exclusively) to the treatment of unruptured aneurysms.
Two general classes of agents are required to achieve platelet inhibition for stenting—aspirin and one of the thienopyridine derivatives (either clopidogrel or ticlopidine). This recommendation represents an extrapolation from studies reported in the cardiology literature that demonstrated that periprocedural and postprocedural dual-agent platelet inhibition reduced the risk for adverse events, including stent thrombosis, compared with aspirin alone or in combination with warfarin.57,58
The most commonly used thienopyridine, clopidogrel, is a prodrug that must be metabolized by the liver to become active. Ticlopidine is a second agent that may be useful in patients who are intolerant of clopidogrel. Clopidogrel is typically the agent of choice, however, because it has a superior safety protocol and a more rapid onset of action.59 The drug may be administered for several days (75 mg) or as a loading dose (300 to 600 mg) 6 to 24 hours before the procedure.
Patient responsiveness to both aspirin and clopidogrel varies dramatically, with a significant percentage of patients being nonresponsive to one or both agents. Aspirin resistance is reported in 5% to 40% of patients treated with standard doses.60,61 Clopidogrel resistance is reported in 5% to 50% of patients treated with standard doses.62,63 Although the definitions of antiplatelet medication resistance have been criticized for being arbitrary and remain controversial,64 patients with aspirin and clopidogrel resistance have been noted to have a higher rates of adverse events after percutaneous coronary interventions.65–67 This has led some institutions to begin performing routine laboratory or point-of-care testing for platelet inhibition in an attempt to determine the importance of these values in predicting events during cerebrovascular interventions and guiding pharmacotherapy for these procedures.68,69
Routine Antiplatelet Regimens
Elective Stenting
Most patients who require stent-supported aneurysm treatment can be identified prospectively on the basis of the available preprocedural imaging and treated electively. Given the rapid onset of activity for aspirin, this agent can be started as soon as the morning of the procedure with the expectation that therapeutic levels will be reached quickly after administration (within minutes). Typically, aspirin is administered for at least 2 days before the procedure to allow for the preprocedural outpatient verification of adequate levels of platelet inhibition. Clopidogrel can be administered as either a daily dose for 3 to 7 days before the procedure or as a bolus dose. Preferably, the clopidogrel is administered as a 600-mg bolus 48 hours before the procedure, followed by a daily dose of 75 mg. This regimen also facilitates outpatient verification of adequate levels of platelet inhibition. The 600-mg clopidogrel loading dose is preferred because of its faster onset of action, greater levels of platelet inhibition in comparison to lower loading doses, lower levels of resistance and of poor response after administration, and cardiology data demonstrating lower rates of adverse events 30 days after percutaneous coronary interventions.70–73
Unanticipated Stenting (Unruptured Aneurysm)
Occasionally, it becomes necessary to use a stent-assisted embolization technique during the course of a procedure in which it was not prospectively anticipated. This situation most commonly arises when embolization coils prolapse from the aneurysm into the parent artery during coiling without an adjunctive device. In these cases, it is often possible to place a stent as a rescue maneuver.44,46
Typically, because no stent placement was anticipated in these cases, these patients are not on dual antiplatelet medications at the time of the procedure. To achieve immediate platelet inhibition after stent deployment, a IIb/IIIa antagonist can be administered. Abciximab (ReoPro, Eli Lilly and Company, Indianapolis) is a useful agent for this purpose. The loading dose of 0.25 mg/kg of abciximab can be administered intravenously; or, if the herniated coil mass is associated with angiographically visible thrombus, intra-arterially.74 Platelet inhibition with the IIb/IIIa antagonists can also be monitored closely with point-of-care testing, with the goal of achieving 80% inhibition of platelet activity.75 After the administration of the loading dose of abciximab, therapeutic levels of platelet inhibition are maintained for 4 to 6 hours. This window allows enough time to administer the loading doses of aspirin (325 mg) and clopidogrel (600 mg) orally after the procedure, obviating the need for a continuous postprocedure infusion. This longer duration of action after a single dose is the primary reason that some investigators have chosen abciximab over the shorter-acting competitive IIb/IIIa receptor antagonists.
Stenting in the Setting of Aneurysmal Subarachnoid Hemorrhage
The requirement for dual antiplatelet therapy almost precludes the application of intracranial stents in the context of acute subarachnoid hemorrhage (SAH). The treatment of ruptured aneurysms is associated with a much higher risk for procedural rerupture, which often becomes catastrophic if antiplatelet medications have been administered for stent prophylaxis. In addition, other invasive procedures that are routinely required by many SAH patients (e.g., ventriculostomy placement, conversion to a permanent ventriculoperitoneal shunt, tracheostomy, percutaneous gastrostomy) are made considerably more complex in the setting of dual antiplatelet therapy.76 For these reasons, stenting in the setting of SAH is typically considered to be a procedure of last resort that is reserved for saccular lesions that absolutely cannot be treated (totally or subtotally) using either a staged (partial coiling followed by subacute stent-supported coiling) or balloon-assisted technique. If noninvasive imaging findings suggest that stenting may be necessary to accomplish aneurysm treatment, it is best to prospectively place a ventriculotomy catheter before the embolization procedure. Computed tomography (CT) should be performed to verify placement and to exclude any hemorrhagic complications.
There are scant data available to indicate the duration of antiplatelet medications required for optimal stent prophylaxis. If invasive procedures are required after stent placement, it is best to delay these as much as possible to allow the stent to become passivated and less thrombogenic.77 The available cardiology literature has indicated that surgical procedures performed early after coronary stent placement are associated with poor outcomes, related either to stent thrombosis or hemorrhagic complications.78,79 Each day, the risk for reversing platelet inhibition lessens significantly. The available cardiology literature suggests that it is possible to stop clopidogrel and convert to aspirin monotherapy, particularly after 2 weeks have elapsed, with much less risk for stent thrombosis.80 If interventions are necessary, the effects of aspirin and clopidogrel can be reversed by platelet transfusions. As soon as possible after the procedure has been completed, the loading doses of both agents should be administered to restore adequate levels of inhibition.
Anticoagulation
All neuroendovascular procedures involving stenting for aneurysm treatment are performed under full therapeutic heparinization targeting an activated coagulation time in the range of 250 to 300 seconds. This is achieved with an initial bolus dose of heparin along with maintenance doses administered throughout the case. The initial bolus of heparin should be given after angiography is performed over the puncture site to exclude any free extravasation or iatrogenic injury encountered during access. At the conclusion of the procedure, the heparin does not need to be reversed with protamine and should be allowed to wear off gradually.77 A postprocedural heparin drip is not necessary to avoid thromboembolic complications with SEIMs in most cases. However, when multiple stents are placed, for example, with Y-stenting procedures, or when the higher metal surface area flow diverters or covered stents are placed, many operators choose to administer heparin for 12 to 24 hours after the procedure.16,22,56
Complications Related to Stenting
Difficult Stent System Delivery
A final issue that can be encountered during the delivery of these bulkier systems over a 0.014-inch exchange microwire is herniation of the delivery system into the aneurysm. System delivery is particularly difficult if a wide-necked aneurysm arises from an acute angle in the vessel. During manipulation of the stent system over the 0.014-inch microwire in these situations, it is possible for the entire delivery device to drive the exchange wire into the aneurysm fundus. If this occurs with enough force, this can result in catastrophic aneurysm perforation. Several steps can be taken to avoid this problem if it is anticipated from the anatomy. First, during exchanges with larger and more rigid systems (balloon-mounted devices or Neuroform), stable, distal 0.014-inch exchange microwire access is required to provide the necessary support for device navigation. A second protective measure is to first coil the aneurysm with a balloon-assisted technique and then exchange the temporary occlusion balloon for the stent delivery system. With this technique, the coil mass acts as a buttress to prevent herniation of the microcatheter system into the aneurysm fundus. If anatomy allows, it is also possible in some cases to achieve stent reconstruction across the circle of Willis,19 avoiding the necessity to make these acute turns with the stent delivery system.
Because patients are given dual antiplatelet medications before treatment and receive full heparinization during the procedure, angiographically evident thromboembolic complications are uncommon during stent-supported aneurysm treatment. If such a complication is encountered, the operator should suspect that the patient has not been appropriately pretreated with antiplatelet medications ( Fig. 376-E1); that the patient is noncompliant, a nonresponder, or a poor responder to the administered medications; or, if the anatomy of the parent vessel–aneurysm complex is difficult to visualize, that the coils are somehow creating luminal compromise.
Angiographically undetectable thromboembolic phenomena are also less common during stent-supported coiling, again likely secondary to the antiplatelet medications in place during the procedure. Brooks and associates81 reported a 13% (6 of 45) rate of diffusion-weighted imaging lesions after stent-supported coiling, compared with 32% (21 of 66) with coiling alone and 24% (8 of 33) with balloon-assisted coiling. In addition to a lower rate of postprocedural diffusion lesions, a lower rate of clinically evident stroke was noted in patients who were treated adjunctive devices (stent or balloon-assisted technique).
Stent Misplacement or Migration
When an SEIM has been placed in a suboptimal position, there are several options to proceed. First, if the original stent does not provide adequate coverage of the aneurysm neck, a second device can be manipulated into position and deployed to provide more complete coverage. Second, if the original stent provides partial coverage of the aneurysm neck, it is usually possible to proceed with aneurysm coiling. Partial coverage most often reduces the functional neck width to a size that can be managed with appropriate microcatheter positioning and coil selection. If it is decided that the neck protection is inadequate during the course of the case, a second stent can be placed. In some cases, the misplaced or displaced stent may have partially herniated into the aneurysm fundus. It is frequently possible to proceed with coiling in these situations. In some cases, the embolization coils may displace the SEIM from the aneurysm fundus back into the parent artery. Even if this does not occur, the portion of the stent that covers the aneurysm neck is often sufficient to provide enough protection to allow for safe coil embolization (Fig. 376-E2). Finally, if the stent becomes displaced into a hazardous position, it is possible, but not advisable, to retrieve these devices using commercially available foreign body retrieval devices (e.g., Alligator, Chestnut Medical; or Amplatz Goose Neck snare, ev3 Endovascular). This maneuver has only been successfully reported with the Neuroform device; and although successful in each of three occasions attempted, it typically resulted in the device shredding into fragments during retrieval (Turner R, Gonugunta V, Kelly M, et al. Successful retrieval of errant Neuroform stents: technique and discussion of four cases. Presentation, Fourth Annual American Society of Interventional Therapeutic Neuroradiology (ASITN) Course and Workshops; Dana Point, CA; July 30–August 3, 2007).
Spontaneous migration of a self-expanding intracranial microstent to our knowledge, has been only observed with the Enterprise.43 The closed-cell structure of the device results in its ability to migrate when placed into vessels of discrepant caliber. For example, when stenting from the ICA into the middle cerebral artery or the basilar artery into the posterior cerebral artery, the caliber of the distal limb tends to be smaller than the proximal parent artery. In these cases, the stent is more constrained distally than proximally. Hypothetically, because of the open-cell structure, the force on the distal stent is transmitted retrograde, and the device can “watermelon seed” backward into the larger, more proximal parent artery (Fig. 376-E3).
If coil stretching is sensed, the operator should immediately stop the coil manipulation and consider the options for safe retrieval. In those cases in which a coil becomes stretched during delivery, the safest and most effective means of retrieval is the “monorail snare” technique,82 in which a second catheter bearing a 2-mm gooseneck snare is manipulated side by side with the microcatheter bearing the coil. The snare is placed around the distal aspect of the coil-bearing microcatheter and gently cinched down and then advanced, using the coil-bearing microcatheter as a monorail to guide the loop of the snare along the catheter and, ultimately, to the stretched coil exiting the microcatheter tip. The snare can then be further cinched down around the damaged coil, and both catheters can be removed along with the damaged coil. By using the coil-bearing microcatheter as a monorail, navigation to the coil is facilitated, and the loop of the snare can be kept free of the stent. This technique can also be used if the coil is broken but still partially contained within the microcatheter.
As discussed previously, in some anatomic configurations, the cells of the SEIMs splay open along the outer curvature of the vessels in which they are deployed. Because the SEIM cage structure cannot be visualized with conventional fluoroscopy, the degree to which this occurs is not evident to the operator. In this scenario, it is possible for embolization coils placed near the aneurysm neck to herniate out of the aneurysm and into the parent artery after detachment (Fig. 376-E4). The herniated coil loops present a long-term thromboembolic risk and, if large enough, can act as a windsock to carry the rest of the coil from the aneurysm into the distal vasculature. The optimal management of this situation is to place a second stent within the parent artery to secure and tack up the prolapsed coil against the parent artery wall (
Video 376-2).
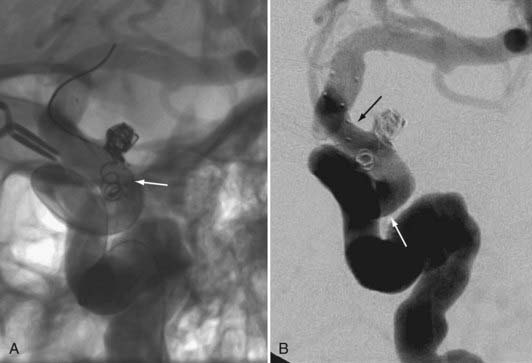
FIGURE 376-E4 Coil herniation through an in situ Neuroform stent (Boston Scientific, Fremont, CA). A, Native image in the working angle for the coil embolization of a small wide-necked left carotid-ophthalmic segment aneurysm. Despite the in situ Neuroform stent across the aneurysm neck, a coil loop prolapsed through the stent after detachment and was noted to be freely pulsating in the parent artery. A second Neuroform stent delivery catheter was manipulated across the aneurysm and deployed to secure the prolapsed coil against the vessel wall (see Video 376-2). B, Subtracted angiographic image after placement of the second stent shows the coil pinned peripherally against the vessel wall.
Ischemic Stroke
Thromboembolic
Most thromboembolic events related to aneurysm treatment occur during the procedure or in the immediate (<48 hours) postprocedural period.83 Although no reliable clinical data exist, the thrombogenicity of an intravascular stent-coil mass construct likely persists for a longer period of time.
Studies of BMCSs explanted from humans have demonstrated only matrix-poor, incomplete intimal coverage by 2 weeks, with complete coverage of the stent at 4 weeks.84–86 This time course may be longer with the SEIMs given that they are deployed around curves and over branch vessels, often with incomplete apposition of struts against the vessel wall. For these reasons, most operators maintain dual antiplatelet medications for 6 to 12 weeks after stenting, with aspirin continued indefinitely thereafter.
In-Stent Stenosis
The incidence of delayed ISS following the implantation of stents for aneurysm treatment is defined only for Neuroform. Delayed ISS following the implantation of Neuroform represents a relatively uncommon (~5%) event.34 Neuroform-induced ISS is usually asymptomatic and, in more than half of the cases reported, spontaneously regressed at angiographic follow-up. The incidence of symptomatic ISS is low (<1.5% of patients) but should be at least a consideration when contemplating stent placement. We hypothesize that ISS represents an exuberant manifestation of the biologic remodeling (endothelial and neointimal overgrowth) that is typically induced after stent placement. It is likely that, in most cases, this reaction is actually beneficial, leading to durable aneurysm occlusion, and that only in rare cases does it progress to the point at which it can actually produce significant luminal compromise, perfusion failure, and clinical symptoms that may lead to ischemic stroke.
Hemorrhage
In a small number of patients, the administration of antiplatelet medications can exacerbate (or potentially lead to) spontaneous cerebral hemorrhage ( Fig. 376-E5), but this is uncommon in the absence of cerebral interventions (e.g., ventriculostomy catheter manipulation). Extracerebral bleeding complications (e.g., gastrointestinal bleeding) are also a potential issue but, again, are minimized to some extent by the limited duration of the required dual antiplatelet therapy.
Conventional Stents not Sufficient
Some categories of aneurysms cannot be treated with conventional intracranial or coronary stents. This is commonly the case with aneurysms arising from dolichoectatic vertebrobasilar vessels in which the parent arteries measure more than 5 mm in diameter. In these unusual circumstances, no SEIMs are large enough to accommodate the parent artery. When other surgical approaches are not feasible, we have opted to treat these lesions with the larger coronary and biliary stenting systems (Fig. 376-E6). The manipulation of the stenting system into the intracranial vertebrobasilar circulation in these cases is extremely challenging and requires direct surgical access into the vertebral artery at the level of C1. After the stent-supported coiling procedure, the access site can be surgically closed.
Imaging Follow-Up
Magnetic Resonance Angiography
Short echo time MRA sequences have been designed specifically to assess aneurysms after endovascular therapy.87 This sequence is designed to minimize susceptibility artifact related to the coil mass and stents. Although effective for imaging of the coil mass and assessing residual aneurysm, MRA is limited to some extent for evaluation of the parent artery after stenting.
Computed Tomographic Angiography
Although modified computed tomographic angiography (CTA) techniques are becoming very effective for the noninvasive follow-up of aneurysms after surgical clipping,88 the data are typically so degraded by streak artifact related to the coil mass that neither the parent artery or aneurysm can be evaluated. CTA is of use in those cases treated with stenting alone because the stents themselves produce much less artifact than the embolization coils.
Protocol
Three to 6 months after stent-supported coiling, we perform a follow-up conventional angiogram and specialized short echo time MRA sequences designed specifically to minimize the susceptibility artifact related to implanted stents and coils.87 Provided that the examinations correlate, this MRA functions as a baseline for subsequent comparison at 12 to 15 months and at 3 and 5 years. Should the coil mass appear stable, it is possible that all of these follow-up evaluations can be performed with MRA alone, with any evidence of increasing flow or contrast enhancement within the coil mass prompting conventional angiography.
Future of Stenting Of Aneurysms: Flow Diversion and Definitive Parent-Vessel Reconstruction
The PED is a self-expanding, microcatheter-delivered, cylindrical mesh device composed of 48 individual braided cobalt chromium and platinum strands. The device has a 30% to 35% metal surface area when fully deployed. This is far greater than the 6.5% to 9% metal surface area coverage provided by the commercially available SEIMs described previously (see Fig. 376-7). The longest devices available are 20 mm; however, multiple devices can be deployed within each other (telescoped) to create a composite endovascular construct that can cover larger diseased vascular segments, as necessary. The degree of metal surface area coverage can be manipulated by varying the technique of device deployment as well as by choosing the number of overlapping devices placed within a particular vascular segment.
Preclinical Data
In vivo animal data35 derived using PED in a rabbit elastase aneurysm model demonstrated that a single device placed over the aneurysm neck was sufficient to achieve complete angiographic occlusion at 6 months. Follow-up histology of these specimens typically demonstrated complete incorporation of the device into the vessel wall with contiguous circumferential neoendothelialization along the entire length of the construct. Endothelialization occurred to the extent that the aneurysm neck frequently could not be visually differentiated from the adjacent parent vessel (Fig. 376-18). Moreover, the actual aneurysm sac in these cases was often found to be not only thrombosed but also atretic at follow-up.
In the same series, Kallmes and colleagues35 placed a single PED within the abdominal aorta, covering the ostia of the lumbar arteries. The rabbit aorta provides an animal model for the intracranial vasculature and its perforator branches because the vessel approximates the caliber of human intracranial arteries and the lumbar artery branches approximate the size of the cerebral perforators. Angiographic and histologic findings showed that none of the lumbar arteries covered by the PEDs demonstrated either immediate or delayed occlusion (up to 6 months). These data indicate that branch vessels remain patent, even when their ostia are partially occluded by the in situ PED construct (see Fig. 376-18).
The ability of the PED to occlude an aneurysm sac while allowing patency of the perforating vessels is believed to stem from the different mechanisms by which flow is maintained into each structure. No pressure gradient exists between the parent artery and aneurysm sac. Flow into the aneurysm is governed completely by the geometry of the parent vessel–aneurysm complex, the orientation of the inflow jet at the aneurysm neck, and the presence of an available, organized outflow. As such, with reconstruction of the parent artery–aneurysm complex, these inflow and outflow pathways become disrupted, and the aneurysmal sac progresses to thrombosis. On the contrary, flow into perforating vessels is governed almost entirely by the presence of a pressure gradient. This gradient functions to drive flow from the larger parent arteries into these smaller perforating vessels and functions to maintain flow, even when the orifices of the perforators are partially (<50%) compromised by stent struts or the strands of a flow-diverting device.55
Clinical Experience
The PITA trial, a single-arm 31-patient clinical safety study, included wide-necked saccular aneurysms and aneurysms that had failed prior endovascular coiling.89 Most aneurysms in the study were large and wide-necked, with an average aneurysm sac diameter of 11.5 mm and an average neck width of 5.8 mm. The device was delivered successfully in 100% of the cases with a 6% rate of periprocedural complications (two strokes, no deaths). At 6-month follow-up, 93% (28 of 30) of the lesions demonstrated complete angiographic occlusion (Figs. 376-19 and 376-20). This unprecedented rate of complete angiographic occlusion at follow-up surpasses any of the reported occlusion rates for aneurysms after endovascular therapy and far exceeds those reported for large or wide-necked lesions.
The PED has also been used to successfully treat nonsaccular (fusiform or circumferential) aneurysms (Fig. 376-21). Three such cases have been performed by Fiorella and coworkers in North America under a U.S. Food and Drug Administration compassionate-use exemption.56 All three lesions, which were judged to be untreatable with existing endovascular or open surgical technologies, were angiographically cured with PED, without technical or periprocedural neurological complications.56 Two of these patients now have more than 1 year of clinical and angiographic follow-up and remain angiographically cured of their lesions and without neurological symptoms.56 However, the third patient experienced a delayed thrombosis of the reconstructed parent artery and subsequently had a fatal stroke. While the etiology of this thrombosis remains unclear, the event underscores how preliminary our experience is with respect to the application of these types of devices to treat complex aneurysms.
Potential Limitations
Although the available data for the PED have been extremely encouraging, there remain several theoretical limitations of the device with respect to its application in the treatment of cerebral aneurysms. First and foremost, data describing both the periprocedural and long-term safety and efficacy of these devices remain quite preliminary. As a larger volume of clinical experience is accrued, the appropriate clinical applications of these devices (as well as their limitations) will become more evident. Second, as with any intracranial stent-like device, a course of dual antiplatelet medications is required for prophylaxis against thrombosis while the construct is becoming endothelialized and incorporated into the parent artery. The optimal duration of dual antiplatelet therapy remains uncertain, but the current recommendation is for aspirin in conjunction with clopidogrel for 6 months and aspirin alone thereafter.56 For this reason, the use of the PED in the setting of aneurysmal SAH represents a relative contraindication, given the potential for complications related to the invasive procedures frequently required during the periprocedural period in these patients (e.g., ventriculostomy catheters, percutaneous gastrostomy tubes, and tracheostomies). Third, the efficacy and safety of the device for the treatment of bifurcation aneurysms remains uncertain. In fact, the safety of crossing or jailing major bifurcation or branch vessels with the device (regardless of the location of the treated aneurysm) remains uncertain. Although this has not been an issue with the current generation of commercially available SEIMS, which are routinely deployed across major vascular bifurcations, these devices have far less metal surface area coverage than the PED. Finally, the safety of the PED in vessels rich with eloquent perforators remains to some extent unknown. The available data, both in experimental animal models and in human patients, suggest that coverage of these segments with a single PED is safe. However, until a larger data set is available, the amount of coverage that can be safely applied in these anatomic locations remains uncertain.
Canton G, Levy DI, Lasheras JC, et al. Flow changes caused by the sequential placement of stents across the neck of sidewall cerebral aneurysms. J Neurosurg. 2005;103:891.
Canton G, Levy DI, Lasheras JC. Hemodynamic changes due to stent placement in bifurcating intracranial aneurysms. J Neurosurg. 2005;103:146.
Chow MM, Woo HH, Masaryk TJ, et al. A novel endovascular treatment of a wide-necked basilar apex aneurysm by using a Y-configuration, double-stent technique. AJNR Am J Neuroradiol. 2004;25:509.
Derdeyn CP, Cross DT3rd, Moran CJ, et al. Postprocedure ischemic events after treatment of intracranial aneurysms with Guglielmi detachable coils. J Neurosurg. 2002;96:837.
Ebrahimi N, Claus B, Lee CY, et al. Stent conformity in curved vascular models with simulated aneurysm necks using flat-panel CT: an in vitro study. AJNR Am J Neuroradiol. 2007;28:823.
Fiorella D, Albuquerque FC, Deshmukh VR, et al. Monorail snare technique for the recovery of stretched platinum coils: technical case report. Neurosurgery. 2005;57:E210.
Fiorella D, Albuquerque FC, Deshmukh VR, et al. Usefulness of the Neuroform stent for the treatment of cerebral aneurysms: results at initial (3-6-mo) follow-up. Neurosurgery. 2005;56:1191.
Fiorella D, Albuquerque FC, Deshmukh VR, et al. Endovascular reconstruction with the Neuroform stent as monotherapy for the treatment of uncoilable intradural pseudoaneurysms. Neurosurgery. 2006;59:291.
Fiorella D, Albuquerque FC, Han P, et al. Preliminary experience using the Neuroform stent for the treatment of cerebral aneurysms. Neurosurgery. 2004;54:6.
Fiorella D, Albuquerque FC, Han P, et al. Strategies for the management of intraprocedural thromboembolic complications with abciximab (ReoPro). Neurosurgery. 2004;54:1089.
Fiorella D, Albuquerque FC, Masaryk TJ, et al. Balloon in-stent technique for the constructive endovascular treatment of “ultra-wide necked” circumferential aneurysms. Neurosurgery. 2005;57:1218.
Fiorella D, Albuquerque FC, McDougall CG. The impact of the Neuroform stent on the durability of aneurysm embolization with Matrix detachable coils (abstract) (paper 53 presented at the Joint Annual Meeting of the AANS/CNS Cerebrovascular Section and the American Society of Interventional & Therapeutic Neurology; Orlando, FL; February 17-20, 2006). Neurosurgery. 2006;58:412.
Fiorella D, Albuquerque FC, Woo H, et al. Neuroform in-stent stenosis: incidence, natural history, and treatment strategies. Neurosurgery. 2006;59:34.
Fiorella D, Thiabolt L, Albuquerque FC, et al. Antiplatelet therapy in neuroendovascular therapeutics. Neurosurg Clin N Am. 2005;16:517.
Fiorella D, Woo HH, Albuquerque FC, et al. Definitive reconstruction of circumferential, fusiform intracranial aneurysms with the Pipeline embolization device. Neurosurgery. 2008;62:1115.
Gum PA, Kottke-Marchant K, Poggio ED, et al. Profile and prevalence of aspirin resistance in patients with cardiovascular disease. Am J Cardiol. 2001;88:230.
Gum PA, Kottke-Marchant K, Welsh PA, et al. A prospective, blinded determination of the natural history of aspirin resistance among stable patients with cardiovascular disease. J Am Coll Cardiol. 2003;41:961.
Hsu SW, Chaloupka JC, Feekes JA, et al. In vitro studies of the Neuroform microstent using transparent human intracranial arteries. AJNR Am J Neuroradiol. 2006;27:1135.
Lopes DK, Ringer AJ, Boulos AS, et al. Fate of branch arteries after intracranial stenting. Neurosurgery. 2003;52:1275.
Lopes D, Sani S. Histological postmortem study of an internal carotid artery aneurysm treated with the Neuroform stent. Neurosurgery. 2005;56:E416.
Lylyk P, Ceratto R, Hurvitz D, et al. Treatment of a vertebral dissecting aneurysm with stents and coils: technical case report. Neurosurgery. 1998;43:385.
Lylyk P, Cohen JE, Ceratto R, et al. Endovascular reconstruction of intracranial arteries by stent placement and combined techniques. J Neurosurg. 2002;97:1306.
Moret J, Cognard C, Weill A, et al. Reconstruction technic in the treatment of wide-neck intracranial aneurysms. Long-term angiographic and clinical results. Apropos of 56 cases. J Neuroradiol. 1997;24:30.
Nelson PK, Levy DI. Balloon-assisted coil embolization of wide-necked aneurysms of the internal carotid artery: medium-term angiographic and clinical follow-up in 22 patients. AJNR Am J Neuroradiol. 2001;22:19.
Phatouros CC, Sasaki TY, Higashida RT, et al. Stent-supported coil embolization: the treatment of fusiform and wide-neck aneurysms and pseudoaneurysms. Neurosurgery. 2000;47:107.
Saatci I, Cekirge HS, Ozturk MH, et al. Treatment of internal carotid artery aneurysms with a covered stent: experience in 24 patients with mid-term follow-up results. AJNR Am J Neuroradiol. 2004;25:1742.
Szikora I, Guterman LR, Wells KM, et al. Combined use of stents and coils to treat experimental wide-necked carotid aneurysms: preliminary results. AJNR Am J Neuroradiol. 1994;15:1091.
Wakhloo AK, Lanzino G, Lieber BB, et al. Stents for intracranial aneurysms: the beginning of a new endovascular era? Neurosurgery. 1998;43:377.
Wakhloo AK, Schellhammer F, de Vries J, et al. Self-expanding and balloon-expandable stents in the treatment of carotid aneurysms: an experimental study in a canine model. AJNR Am J Neuroradiol. 1994;15:493.
Wakhloo AK, Tio FO, Lieber BB, et al. Self-expanding nitinol stents in canine vertebral arteries: hemodynamics and tissue response. AJNR Am J Neuroradiol. 1995;16:1043.
1 Moret J, Cognard C, Weill A, et al. Reconstruction technic in the treatment of wide-neck intracranial aneurysms. Long-term angiographic and clinical results. Apropos of 56 cases. J Neuroradiol. 1997;24:30.
2 Nelson PK, Levy DI. Balloon-assisted coil embolization of wide-necked aneurysms of the internal carotid artery: medium-term angiographic and clinical follow-up in 22 patients. AJNR Am J Neuroradiol. 2001;22:19.
3 Szikora I, Guterman LR, Wells KM, et al. Combined use of stents and coils to treat experimental wide-necked carotid aneurysms: preliminary results. AJNR Am J Neuroradiol. 1994;15:1091.
4 Wakhloo AK, Schellhammer F, de Vries J, et al. Self-expanding and balloon-expandable stents in the treatment of carotid aneurysms: an experimental study in a canine model. AJNR Am J Neuroradiol. 1994;15:493.
5 Wakhloo AK, Tio FO, Lieber BB, et al. Self-expanding nitinol stents in canine vertebral arteries: hemodynamics and tissue response. AJNR Am J Neuroradiol. 1995;16:1043.
6 Higashida RT, Smith W, Gress D, et al. Intravascular stent and endovascular coil placement for a ruptured fusiform aneurysm of the basilar artery. Case report and review of the literature. J Neurosurg. 1997;87:944.
7 Han PP, Albuquerque FC, Ponce FA, et al. Percutaneous intracranial stent placement for aneurysms. J Neurosurg. 2003;99:23.
8 Lanzino G, Wakhloo AK, Fessler RD, et al. Efficacy and current limitations of intravascular stents for intracranial internal carotid, vertebral, and basilar artery aneurysms. J Neurosurg. 1999;91:538.
9 Lylyk P, Ceratto R, Hurvitz D, et al. Treatment of a vertebral dissecting aneurysm with stents and coils: technical case report. Neurosurgery. 1998;43:385.
10 Malek AM, Halbach VV, Phatouros CC, et al. Balloon-assist technique for endovascular coil embolization of geometrically difficult intracranial aneurysms. Neurosurgery. 2000;46:1397.
11 Mericle RA, Lanzino G, Wakhloo AK, et al. Stenting and secondary coiling of intracranial internal carotid artery aneurysm: technical case report. Neurosurgery. 1998;43:1229.
12 Sekhon LH, Morgan MK, Sorby W, et al. Combined endovascular stent implantation and endosaccular coil placement for the treatment of a wide-necked vertebral artery aneurysm: technical case report. Neurosurgery. 1998;43:380.
13 Wilms G, van Calenbergh F, Stockx L, et al. Endovascular treatment of a ruptured paraclinoid aneurysm of the carotid siphon achieved using endovascular stent and endosaccular coil placement. AJNR Am J Neuroradiol. 2000;21:753.
14 Fiorella D, Albuquerque FC, Deshmukh VR, et al. Usefulness of the Neuroform stent for the treatment of cerebral aneurysms: results at initial (3-6-mo) follow-up. Neurosurgery. 2005;56:1191.
15 Benndorf G, Klucznik RP, Meyer D, et al. “Cross-over” technique for horizontal stenting of an internal carotid bifurcation aneurysm using a new self-expandable stent: technical case report. Neurosurgery. 58, 2006. ONS
16 Chow MM, Woo HH, Masaryk TJ, et al. A novel endovascular treatment of a wide-necked basilar apex aneurysm by using a Y-configuration, double-stent technique. AJNR Am J Neuroradiol. 2004;25:509.
17 Cross DT3rd, Moran CJ, Derdeyn CP, et al. Neuroform stent deployment for treatment of a basilar tip aneurysm via a posterior communicating artery route. AJNR Am J Neuroradiol. 2005;26:2578.
18 Fiorella D, Albuquerque FC, Masaryk TJ, et al. Balloon in-stent technique for the constructive endovascular treatment of “ultra-wide necked” circumferential aneurysms. Neurosurgery. 2005;57:1218.
19 Kelly ME, Turner R, Gonugunta V, et al. Stent reconstruction of wide-necked aneurysms across the circle of Willis. Neurosurgery. 2007;61:249.
20 Moret J, Ross IB, Weill A, et al. The retrograde approach: a consideration for the endovascular treatment of aneurysms. AJNR Am J Neuroradiol. 2000;21:262.
21 Sani S, Lopes DK. Treatment of a middle cerebral artery bifurcation aneurysm using a double Neuroform stent “Y” configuration and coil embolization: technical case report. Neurosurgery. 2005;57:E209.
22 Thorell WE, Chow MM, Woo HH, et al. Y-configured dual intracranial stent-assisted coil embolization for the treatment of wide-necked basilar tip aneurysms. Neurosurgery. 2005;56:1035.
23 Canton G, Levy DI, Lasheras JC. Hemodynamic changes due to stent placement in bifurcating intracranial aneurysms. J Neurosurg. 2005;103:146.
24 Canton G, Levy DI, Lasheras JC, et al. Flow changes caused by the sequential placement of stents across the neck of sidewall cerebral aneurysms. J Neurosurg. 2005;103:891.
25 Lopes D, Sani S. Histological postmortem study of an internal carotid artery aneurysm treated with the Neuroform stent. Neurosurgery. 2005;56:E416.
26 Fiorella D, Albuquerque FC, McDougall CG. The impact of the Neuroform stent on the durability of aneurysm embolization with Matrix detachable coils (abstract) (paper 53 presented at the Joint Annual Meeting of the AANS/CNS Cerebrovascular Section and the American Society of Interventional & Therapeutic Neurology; Orlando, FL; February 17-20, 2006). Neurosurgery. 2006;58:412.
26a Fiorella D, Albuquerque FC, Woo H, et al. Neuroform stent-supported embolization of cerebral aneurysms: impact on recanalization rate. Presented at the 15th ABC Seminar/25th Working Group in Interventional Neuroradiology; Val D’Isère, France; January 15-20. 2006.
27 Ansari SA, Lassig JP, Nicol E, et al. Thrombosis of a fusiform intracranial aneurysm induced by overlapping Neuroform stents: case report. Neurosurgery. 2007;60:E950.
28 Brassel F, Rademaker J, Haupt C, et al. Intravascular stent placement for a fusiform aneurysm of the posterior cerebral artery: case report. Eur Radiol. 2001;11:1250.
29 Fiorella D, Albuquerque FC, Deshmukh VR, et al. Endovascular reconstruction with the Neuroform stent as monotherapy for the treatment of uncoilable intradural pseudoaneurysms. Neurosurgery. 2006;59:291.
30 Geremia G, Haklin M, Brennecke L. Embolization of experimentally created aneurysms with intravascular stent devices. AJNR Am J Neuroradiol. 1994;15:1223.
31 Lylyk P, Cohen JE, Ceratto R, et al. Endovascular reconstruction of intracranial arteries by stent placement and combined techniques. J Neurosurg. 2002;97:1306.
32 Mehta B, Burke T, Kole M, et al. Stent-within-a-stent technique for the treatment of dissecting vertebral artery aneurysms. AJNR Am J Neuroradiol. 2003;24:1814.
33 Vanninen R, Manninen H, Ronkainen A. Broad-based intracranial aneurysms: thrombosis induced by stent placement. AJNR Am J Neuroradiol. 2003;24:263.
34 Fiorella D, Albuquerque FC, Woo H, et al. Neuroform in-stent stenosis: incidence, natural history, and treatment strategies. Neurosurgery. 2006;59:34.
35 Kallmes DF, Ding YH, Dai D, et al. A new endoluminal, flow-disrupting device for treatment of saccular aneurysms. Stroke. 2007;38:2346.
36 Freitas JM, Zenteno M, Aburto-Murrieta Y, et al. Intracranial arterial stenting for symptomatic stenoses: a Latin American experience. Surg Neurol. 2007;68:378.
37 Kurre W, Berkefeld J, Sitzer M, et al. Treatment of symptomatic high-grade intracranial stenoses with the balloon-expandable Pharos stent: initial experience. Neuroradiology. 2008;50:701-708.
38 Zenteno MA, Santos-Franco JA, Freitas-Modenesi JM, et al. Use of the sole stenting technique for the management of aneurysms in the posterior circulation in a prospective series of 20 patients. J Neurosurg. 2008;108:1104.
39 Phatouros CC, Sasaki TY, Higashida RT, et al. Stent-supported coil embolization: the treatment of fusiform and wide-neck aneurysms and pseudoaneurysms. Neurosurgery. 2000;47:107.
40 Wakhloo AK, Lanzino G, Lieber BB, et al. Stents for intracranial aneurysms: the beginning of a new endovascular era? Neurosurgery. 1998;43:377.
41 Ebrahimi N, Claus B, Lee CY, et al. Stent conformity in curved vascular models with simulated aneurysm necks using flat-panel CT: an in vitro study. AJNR Am J Neuroradiol. 2007;28:823.
42 Hsu SW, Chaloupka JC, Feekes JA, et al. In vitro studies of the Neuroform microstent using transparent human intracranial arteries. AJNR Am J Neuroradiol. 2006;27:1135.
43 Kelly ME, Turner RI, Moskowitz S, et al. Delayed migration of a self-expanding intracranial microstent. AJNR Am J Neuroradiol. 2008;29:1959-1960.
44 Fiorella D, Albuquerque FC, Han P, et al. Preliminary experience using the Neuroform stent for the treatment of cerebral aneurysms. Neurosurgery. 2004;54:6.
45 Hanel RA, Boulos AS, Sauvageau EG, et al. Stent placement for the treatment of nonsaccular aneurysms of the vertebrobasilar system. Neurosurg Focus. 2005;18:E8.
46 Lavine SD, Larsen DW, Giannotta SL, et al. Parent vessel Guglielmi detachable coil herniation during wide-necked aneurysm embolization: treatment with intracranial stent placement: two technical case reports. Neurosurgery. 2000;46:1013.
47 Horowitz M, Levy E, Sauvageau E, et al. Intra/extra-aneurysmal stent placement for management of complex and wide-necked-bifurcation aneurysms: eight cases using the waffle cone technique. Neurosurgery. 58, 2006. ONS
48 Chiaradio JC, Guzman L, Padilla L, et al. Intravascular graft stent treatment of a ruptured fusiform dissecting aneurysm of the intracranial vertebral artery: technical case report. Neurosurgery. 2002;50:213.
49 Islak C, Kocer N, Albayram S, et al. Bare stent-graft technique: a new method of endoluminal vascular reconstruction for the treatment of giant and fusiform aneurysms. AJNR Am J Neuroradiol. 2002;23:1589.
50 Saatci I, Cekirge HS, Ozturk MH, et al. Treatment of internal carotid artery aneurysms with a covered stent: experience in 24 patients with mid-term follow-up results. AJNR Am J Neuroradiol. 2004;25:1742.
51 Li MH, Li YD, Gao BL, et al. A new covered stent designed for intracranial vasculature: application in the management of pseudoaneurysms of the cranial internal carotid artery. AJNR Am J Neuroradiol. 2007;28:1579.
52 Li MH, Zhu YQ, Fang C, et al. The feasibility and efficacy of treatment with a Willis covered stent in recurrent intracranial aneurysms after coiling. AJNR Am J Neuroradiol. 2008;29:1395-1400.
53 Wang JB, Li MH, Fang C, et al. Endovascular treatment of giant intracranial aneurysms with Willis covered stents: technical case report. Neurosurgery. 2008;62:E1176.
54 Marotta TR, Gunnarsson T, Penn I, et al. A novel endovascular clip system for the treatment of intracranial aneurysms: technology, concept, and initial experimental results. Laboratory investigation. J Neurosurg. 2008;108:1230.
55 Lopes DK, Ringer AJ, Boulos AS, et al. Fate of branch arteries after intracranial stenting. Neurosurgery. 2003;52:1275.
56 Fiorella D, Woo HH, Albuquerque FC, et al. Definitive reconstruction of circumferential, fusiform intracranial aneurysms with the Pipeline embolization device. Neurosurgery. 2008;62:1115.
57 Schomig A, Neumann FJ, Kastrati A, et al. A randomized comparison of antiplatelet and anticoagulant therapy after the placement of coronary-artery stents. N Engl J Med. 1996;334:1084.
58 Urban P, Macaya C, Rupprecht HJ, et al. Randomized evaluation of anticoagulation versus antiplatelet therapy after coronary stent implantation in high-risk patients: the multicenter aspirin and ticlopidine trial after intracoronary stenting (MATTIS). Circulation. 1998;98:2126.
59 Bertrand ME, Rupprecht HJ, Urban P, et al. Double-blind study of the safety of clopidogrel with and without a loading dose in combination with aspirin compared with ticlopidine in combination with aspirin after coronary stenting: the clopidogrel aspirin stent international cooperative study (CLASSICS). Circulation. 2000;102:624.
60 Gum PA, Kottke-Marchant K, Poggio ED, et al. Profile and prevalence of aspirin resistance in patients with cardiovascular disease. Am J Cardiol. 2001;88:230.
61 McKee SA, Sane DC, Deliargyris EN. Aspirin resistance in cardiovascular disease: a review of prevalence, mechanisms, and clinical significance. Thromb Haemost. 2002;88:711.
62 Gurbel PA, Bliden KP, Hiatt BL, et al. Clopidogrel for coronary stenting: response variability, drug resistance, and the effect of pretreatment platelet reactivity. Circulation. 2003;107:2908.
63 Muller I, Besta F, Schulz C, et al. Prevalence of clopidogrel non-responders among patients with stable angina pectoris scheduled for elective coronary stent placement. Thromb Haemost. 2003;89:783.
64 Patrono C. Aspirin resistance: definition, mechanisms and clinical read-outs. J Thromb Haemost. 2003;1:1710.
65 Chen WH, Lee PY, Ng W, et al. Aspirin resistance is associated with a high incidence of myonecrosis after non-urgent percutaneous coronary intervention despite clopidogrel pretreatment. J Am Coll Cardiol. 2004;43:1122.
66 Gum PA, Kottke-Marchant K, Welsh PA, et al. A prospective, blinded determination of the natural history of aspirin resistance among stable patients with cardiovascular disease. J Am Coll Cardiol. 2003;41:961.
67 Matetzky S, Shenkman B, Guetta V, et al. Clopidogrel resistance is associated with increased risk of recurrent atherothrombotic events in patients with acute myocardial infarction. Circulation. 2004;109:3171.
68 Lee DH, Arat A, Morsi H, et al. Dual antiplatelet therapy monitoring for neurointerventional procedures using a point-of-care platelet function test: a single-center experience. AJNR Am J Neuroradiol. 2008;29:1389-1394.
69 Prabhakaran S, Wells KR, Lee VH, et al. Prevalence and risk factors for aspirin and clopidogrel resistance in cerebrovascular stenting. AJNR Am J Neuroradiol. 2008;29:281.
70 Cuisset T, Frere C, Quilici J, et al. Benefit of a 600-mg loading dose of clopidogrel on platelet reactivity and clinical outcomes in patients with non-ST-segment elevation acute coronary syndrome undergoing coronary stenting. J Am Coll Cardiol. 2006;48:1339.
71 Lotrionte M, Biondi-Zoccai GG, Agostoni P, et al. Meta-analysis appraising high clopidogrel loading in patients undergoing percutaneous coronary intervention. Am J Cardiol. 2007;100:1199.
72 Montalescot G, Sideris G, Meuleman C, et al. A randomized comparison of high clopidogrel loading doses in patients with non-ST-segment elevation acute coronary syndromes: the ALBION (Assessment of the Best Loading Dose of Clopidogrel to Blunt Platelet Activation, Inflammation and Ongoing Necrosis) trial. J Am Coll Cardiol. 2006;48:931.
73 Patti G, Colonna G, Pasceri V, et al. Randomized trial of high loading dose of clopidogrel for reduction of periprocedural myocardial infarction in patients undergoing coronary intervention: results from the ARMYDA-2 (Antiplatelet therapy for Reduction of MYocardial Damage during Angioplasty) study. Circulation. 2005;111:2099.
74 Fiorella D, Albuquerque FC, Han P, et al. Strategies for the management of intraprocedural thromboembolic complications with abciximab (ReoPro). Neurosurgery. 2004;54:1089.
75 Steinhubl SR. Assessing the optimal level of platelet inhibition with GPIIb/IIIa inhibitors in patients undergoing coronary intervention. Rationale and design of the GOLD study. J Thromb Thrombolysis. 2000;9:199.
76 Tumialan LM, Zhang YJ, Cawley CM, et al. Intracranial hemorrhage associated with stent-assisted coil embolization of cerebral aneurysms: a cautionary report. J Neurosurg. 2008;108:1122.
77 Fiorella D, Thiabolt L, Albuquerque FC, et al. Antiplatelet therapy in neuroendovascular therapeutics. Neurosurg Clin N Am. 2005;16:517.
78 Kaluza GL, Joseph J, Lee JR, et al. Catastrophic outcomes of noncardiac surgery soon after coronary stenting. J Am Coll Cardiol. 2000;35:1288.
79 Wilson SH, Fasseas P, Orford JL, et al. Clinical outcome of patients undergoing non-cardiac surgery in the two months following coronary stenting. J Am Coll Cardiol. 2003;42:234.
80 Berger PB, Bell MR, Hasdai D, et al. Safety and efficacy of ticlopidine for only 2 weeks after successful intracoronary stent placement. Circulation. 1999;99:248.
81 Brooks NP, Turk AS, Niemann DB, et al. Frequency of thromboembolic events associated with endovascular aneurysm treatment: retrospective case series. J Neurosurg. 2008;108:1095.
82 Fiorella D, Albuquerque FC, Deshmukh VR, et al. Monorail snare technique for the recovery of stretched platinum coils: technical case report. Neurosurgery. 2005;57:E210.
83 Derdeyn CP, Cross DT3rd, Moran CJ, et al. Postprocedure ischemic events after treatment of intracranial aneurysms with Guglielmi detachable coils. J Neurosurg. 2002;96:837.
84 Farb A, Burke AP, Kolodgie FD, et al. Pathological mechanisms of fatal late coronary stent thrombosis in humans. Circulation. 2003;108:1701.
85 Farb A, Sangiorgi G, Carter AJ, et al. Pathology of acute and chronic coronary stenting in humans. Circulation. 1999;99:44.
86 Grewe PH, Deneke T, Machraoui A, et al. Acute and chronic tissue response to coronary stent implantation: pathologic findings in human specimen. J Am Coll Cardiol. 2000;35:157.
87 Wallace RC, Karis JP, Partovi S, et al. Noninvasive imaging of treated cerebral aneurysms. Part I: MR angiographic follow-up of coiled aneurysms. AJNR Am J Neuroradiol. 2007;28:1001.
88 Wallace RC, Karis JP, Partovi S, et al. Noninvasive imaging of treated cerebral aneurysms, Part II: CT angiographic follow-up of surgically clipped aneurysms. AJNR Am J Neuroradiol. 2007;28:1207.
89 Nelson PK. Stent for treatment of intracranial aneurysms. Presented at AANS/CNS Cerebrovascular Section Meeting, International Stroke Conference; New Orleans; February 20-22. 2008.