Chapter 17 Endovascular Neurosurgery
• Endovascular neurosurgery has undergone tremendous expansion in the past two decades and is becoming a mainstay of treatment of cerebrovascular disease and other vascular disease of the head and neck as a primary or alternative treatment to open microvascular treatment.
• Endovascular techniques are used to treat a wide variety of cerebrovascular conditions that include severe symptomatic vasospasm after subarachnoid hemorrhage (SAH), arteriovenous malformation (AVM) and tumor embolization, cerebral aneurysm occlusion, and temporary or permanent balloon occlusion during parent vessel sacrifice.
• Endovascular therapy is expanding the time-window after stroke symptom onset for revascularization in patients with acute ischemic stroke. Clinical trials have established a benefit of intra-arterial thrombolysis (IAT) up to 6 hours after stroke symptom onset, with an increase in recanalization rates. The mechanical device trials show effectiveness of mechanical revascularization therapy up to 8 hours after stroke symptom onset.
• Endovascular treatment of intracranial aneurysms has undergone and continues to undergo improvements since the introduction of Guglielmi detachable coils for endosaccular occlusion of cerebral aneurysms in 1994. Current adjunctive techniques include balloon-assisted and stent-assisted coiling to improve the success rate of long-term occlusion of the aneurysm. Flow diversion for giant aneurysms may be a promising technology.
• Preoperative endovascular embolization of feeding arteries has rendered many previously difficult AVMs much easier to remove surgically. Only a minority of AVMs can be cured by endovascular embolization despite the significant advances in embolic material such as Onyx. Most intracranial tumor embolization procedures have been performed on neoplasms with robust vascular pedicles in order to decrease the blood loss during resection.
• Endovascular therapy is evolving and adds a plethora of tools and opens up multiple options with which to treat complex vascular lesions, in addition to microsurgical approaches. Currently, neurosurgeons who desire to be trained in treating the entire spectrum of cerebrovascular disease may pursue both microsurgery and endovascular neurosurgery training.
Introduction (Box 17.1)
The treatment of neurovascular diseases has been completely revolutionized with the introduction and growth of endovascular neurosurgery. Endovascular neurosurgery is becoming a primary treatment alternative to conventional open surgery for multiple neurovascular pathological conditions, including carotid and vertebral atherosclerotic stenosis, cervical vessel dissections, acute and chronic cerebral ischemia, intracranial aneurysms, arteriovenous malformations (AVMs), and dural arteriovenous fistulas (dAVFs). Endovascular revascularization has resulted in recanalization rates as high as 70% to 100% for acute large vessel occlusions responsible for ischemic stroke in patients in whom intravenous (IV) tissue plasminogen activator (t-PA) therapy has failed or was contraindicated. Endovascular techniques are used to treat severe symptomatic vasospasm after subarachnoid hemorrhage (SAH), for tumor embolization, and for temporary or permanent balloon occlusion during parent vessel sacrifice. Neuroendovascular surgery is being increasingly integrated into the neurosurgical training curriculum. It is essential for future neurosurgeons to have a basic understanding of endovascular techniques and tools and have them as an option for treating cerebrovascular pathological conditions. In this chapter, we discuss the basics of arterial access, catheters for supra-aortic and intracranial access, access site management, and the rationale and techniques for the treatment of various cerebrovascular diseases.
BOX 17.1 Abbreviations Used
ACAS, Asymptomatic Carotid Atherosclerosis Study
ACST, Asymptomatic Carotid Surgery Trial
AVM, arteriovenous malformation
CAS, carotid angioplasty with stenting
COCOA, Complete Occlusion of Coilable Intracranial Aneurysms Study
DAC, distal access catheter(s)
dAVF, dural arteriovenous fistula
DSMB, Data and Safety Monitoring Board
ECST, European Carotid Surgery Trial
EVIDENCE, Endovascular Treatment of Intracranial Aneurysms with Pipeline versus Coils with or without Stents
FDA, Food and Drug Administration
GESICA Groupe d’Etude des Sténoses Intra-Crâniennes Athéromateuses symptomatiques
IAT, intra-arterial thrombolysis
IDE, Investigational Device Exemption
MERCI, Mechanical Embolus Removal in Cerebral Ischemia
NASCET, North American Symptomatic Carotid Endarterectomy Trial
NIH, National Institutes of Health
NIHSS, National Institutes of Health Stroke Scale
NINDS, National Institute of Neurological Disorders and Stroke
PED, Pipeline Embolization Device
PGLA, polyglycolic-polylactic acid
PITA, Pipeline Embolization Device in the Intracranial Treatment of Aneurysms
PROACT, Prolyse in Acute Cerebral Thromboembolism
PUFS, Pipeline for Uncoilable or Failed Aneurysms Study
SAMMPRIS, Stenting vs. Aggressive Medical Management for Preventing Recurrent Stroke in Intracranial Stenosis
SAPPHIRE, Stenting and Angioplasty with Protection in Patients at High Risk for Endarterectomy
SARIS, Stent-Assisted Recanalization in Acute Ischemic Stroke
SWIFT, Solitaire FR with the Intention for Thrombectomy
TIA, transient ischemic attack
TIMI, Thrombolysis in Myocardial Infarction
TLR, target lesion revascularization
t-PA, tissue plasminogen activator
WASID, Warfarin vs. Aspirin for Symptomatic Intracranial Disease
General Principles
Contrast Agents
Iohexol, a nonionic contrast agent, is the agent most commonly used for cerebral angiography. Patients with normal renal function can tolerate 400 to 800 mL of iohexol administered at a concentration of 300 mg/mL without adverse effects. Nonionic, low-osmolality contrast agents, such as iodixanol and iopromide, have been shown to be less renal-toxic when compared to iohexol.
Femoral Artery Puncture
Radial or brachial artery access can be attempted if femoral artery access is not feasible.
Carotid and Vertebral Artery Catheterization
For carotid artery catheterization, the innominate artery is engaged, and the wire is advanced superiorly into the right CCA, followed by the catheter. To engage the left CCA, the wire is brought inside the catheter, and the catheter is withdrawn and pointed to the left. If selective ICA catheterization is planned, angiography of the cervical carotid arterial system should be done to check for ICA stenosis. Once the CCA is catheterized, turning the head away from the side being catheterized facilitates catheterization of the ICA and turning toward the ipsilateral side facilitates ECA catheterization.
Ischemic Stroke Intervention
Stroke remains the third most common cause of death in industrialized nations and the single most common reason for permanent adult disability.1 Each year, approximately 795,000 Americans experience a new or recurrent stroke.2 The estimated direct and indirect cost of stroke for 2010 is $73.7 billion.2 The incidence of new or recurrent strokes per year is projected to rise to 1.2 million per year by 2025.3
The only medical therapy approved by the Food and Drug Administration (FDA) for acute ischemic stroke treatment until recently was IV recombinant t-PA (rt-PA) administered within 3 hours of symptom onset to patients eligible for thrombolysis.4,5 The American Heart Association/American Stroke Association guidelines6 recommend evaluating patients for consideration of IV t-PA therapy 3 to 4.5 hours after stroke symptom onset (on the basis of data from the European Cooperative Acute Stroke Study III).7 Unfortunately, less than 1% of acute ischemic stroke patients in the United States receive t-PA, primarily because of a delay in presentation for treatment.8 Early reocclusion following thrombolysis has been demonstrated by transcranial Doppler imaging to occur in 34% of patients receiving IV t-PA and may result in neurological worsening in many of these patients.9–11 Recanalization rates after IV t-PA therapy for proximal, large vessel arterial occlusions are poor and range from only 10% for ICA occlusion to 30% for middle cerebral artery (MCA) occlusion.12 IV thrombolysis (IVT) is not as effective in thromboembolic obstruction of these large, proximal vessels, as compared with more distal smaller vessels.13 The outcome after large intracranial vessel thromboembolic occlusion currently remains dismal and is associated with high rates of morbidity and mortality.14–17 Patients who do not meet the eligibility criteria for thrombolytic therapy, who fail to improve neurologically after thrombolytic therapy, or who improve and then worsen (patients with reocclusion) are currently candidates for endovascular revascularization therapies.
Endovascular Stroke Revascularization Techniques
Time Window for Endovascular Stroke Therapy
Endovascular therapy is expanding the time window after stroke symptom onset for revascularization in patients with acute ischemic stroke The Prolyse in Acute Cerebral Thromboembolism (PROACT) trials18,19 established a benefit of intra-arterial thrombolysis (IAT) up to 6 hours after stroke symptom onset, with an increase in recanalization rates. Flow is reestablished faster with mechanical revascularization strategies than with thrombolytics; thus, such strategies may increase the benefit of treatment, even when there is a delay in presentation for treatment. The Mechanical Embolus Removal in Cerebral Ischemia (MERCI),20,21 Multi-MERCI,22 and Penumbra23 trials show effectiveness of mechanical revascularization therapy up to 8 hours after stroke symptom onset. There is increasing evidence that identification of potentially salvageable brain tissue with advanced magnetic resonance (MR) and computed tomography (CT) imaging may allow the selection of patients who can be effectively and safely treated more than 8 hours post ictus.7,24–29
Higher Recanalization Rate and Improved Outcome after Stroke Intervention
In MERCI,20,21 Multi-MERCI,22 and the combined analysis of data from the Interventional Management of Stroke I and II studies,30 functional outcome (measured by modified Rankin Scale [mRS] score of ≤2 at 3 months) was significantly better and the 3-month mortality rate was significantly lower in patients who had thrombolysis in myocardial infarction (TIMI) scores 2 or 3 recanalization than in patients in whom vessels failed to recanalize after endovascular therapy. In a review of 53 studies that included 2066 patients, good functional outcome (mRS score ≤2) at 3 months was identified more frequently in patients with vessel recanalization than without vessel recanalization.31 The 3-month mortality rate was reduced in patients in whom vessels were recanalized. Higher rates of recanalization were achieved with endovascular methods, particularly mechanical therapies, and consequently were associated with better outcomes.
Merci Device (Fig. 17.1 and Video 17.1)
The Merci retriever system has a flexible nitinol wire that assumes a helical shape once it emerges from the tip of the microcatheter. A microcatheter containing this wire is passed distal to the thrombus, the catheter is withdrawn, and the wire, in its helical configuration, ensnares the clot for removal from the vasculature. Vessels amenable to embolectomy with the Merci device include the ICA, M1 and M2 segments of the MCA, VA, basilar artery, and the posterior cerebral artery. The retriever is then retracted into the guide catheter under proximal flow arrest. FDA approval of the Merci device in 2004 was based on a review of data obtained in the multicenter MERCI trial that involved 141 patients (mean age, 60 years; mean National Institutes of Health Stroke Scale [NIHSS] score, 20) ineligible for standard thrombolytic therapy.20,21 The Multi-MERCI trial22 is a prospective, multicenter, single-arm registry that included 164 patients who were treated with different Merci retrieval systems (X5, X6, and L5).
Penumbra Device (Fig. 17.2 and Video 17.2)
The Penumbra System (Penumbra, Inc., San Leandro, CA) is the second FDA-approved mechanical treatment for acute stroke. The system primarily involves clot aspiration using a microcatheter attached to a powered aspiration pump that is capable of producing 25 mm Hg of suction. The separator is a soft wire with a 6-mm teardrop-shaped enlargement proximal to its tip and is inserted via the microcatheter to fragment the clot and keep the microcatheter tip from clogging. A major advantage over the Merci system is that the Penumbra System works at the proximal face of the clot, eliminating the need to blindly position the microcatheter distal to the occlusion. A prospective, single-arm, independently monitored trial was performed to assess the efficiency and safety of the Penumbra System.23 In a prospective multicenter single-arm trial of 125 patients with acute stroke who underwent revascularization with the Penumbra device, TIMI 2 or 3 recanalization was achieved in 81.6% patients, with a symptomatic intracranial hemorrhage (ICH) rate of 11.2% and only 25% of patients achieving an mRS score of 2 or less.32
Stent-Assisted Thrombolysis
Self-expanding stents (SES) designed specifically for the cerebrovasculature are available. These stents can be delivered to intracranial target areas with a greater than 95% technical success rate with an increased safety profile because they are deployed at significantly lower pressures than balloon-mounted coronary stents.33 With the stent-for-stroke technique (using Wingspan [Boston Scientific] and Enterprise [Codman Neurovascular] SES), vessel recanalization is instantaneous, and the chance of early reocclusion after treatment is decreased. Reocclusion after IVT (34%) and pharmacological IAT (17%) has been shown and is associated with poor outcome.34
For stent-assisted thrombolysis, standard femoral access is obtained, and a 6F (or larger) guide catheter is placed in the target vessel proximal to the occlusion. To minimize the release of distal emboli, the occlusion is crossed in a fashion similar to that used for the Merci clot retriever. First, a .014-inch steerable wire is softly advanced through the clot. A low-profile catheter is then advanced over the wire distal to the occlusion. Following microangiographic confirmation that the microcatheter is distal to the occlusion, an exchange wire is brought through the microcatheter and anchored distal to the occlusion. The microcatheter is removed, and the stent delivery catheter is delivered over the exchange wire. To minimize the release of debris, the stent is deployed first distal to the occlusion (thus trapping any debris that may be later released between the stent and the vessel wall), then through the occlusion, and finally just proximal to the occlusion.
Several retrospective case series reported successful use of SES for acute stroke treatment, with higher rates of recanalization than other recanalization modalities.35–37 Presently, SES are used only off-label under a humanitarian device exemption as a salvage therapy when current FDA-approved thrombectomy devices fail. On the basis of these preliminary data, we received FDA approval for a pilot study, Stent-Assisted Recanalization in Acute Ischemic Stroke (SARIS),38 to evaluate the Wingspan stent for revascularization in patients who did not improve after IVT or had a contraindication to IVT. Average presenting NIHSS score was 14. Seventeen patients presented with a TIMI score of 0 and three patients with a TIMI score of 1. Intracranial SES were placed in 19 of 20 enrolled patients. One patient experienced recanalization of the occluded vessel during positioning of the Wingspan stent delivery system, prior to stent deployment. In two patients, the tortuous vessel did not allow tracking of the Wingspan stent. The more navigable Enterprise stent was used in both these cases. Twelve patients had other adjunctive therapies. TIMI 2 or 3 recanalization was achieved in 100% of patients; 65% of patients improved more than 4 points in NIHSS score after treatment. One patient (5%) had symptomatic ICH, and two had asymptomatic ICH. At the time of the 1-month follow-up evaluation, 12 of 20 (60%) patients had mRS scores of 2 or less and nine (45%) had mRS scores of 1 or less. Mortality rate at 1 month was 25%. None of these patients died because of stent-placement-related causes; all deaths were due to the severity of the initial stroke and associated comorbid conditions.
Stent-Platform-Based Thrombectomy Devices (Stentrievers)
The Solitaire FR Revascularization Device (ev3) (Figs. 17.3 and 17.4) and Trevo device (Concentric Medical Inc.) are recoverable self-expanding thrombectomy devices that are being tested clinically in the United States and Europe, respectively, for acute ischemic stroke revascularization. The advantage of these devices is that they are fully recoverable, SES-platform-based devices that can be used for both temporary endovascular bypass and thrombectomy. The devices restore flow immediately and avoid the placement of a permanent stent, thus obviating the need for antithrombotic therapy and the risk of in-stent stenosis. The Solitaire can be electrolytically detached like a coil in case a permanent stent is necessary, such as in the setting of an atherothrombotic lesion. The Solitaire FR With the Intention for Thrombectomy (SWIFT) trial is an ongoing multicenter randomized controlled trial that is testing the ability of the Solitaire FR device versus the Merci device to achieve TIMI 2 or 3 recanalization without symptomatic ICH. Two small preliminary studies with this device have shown promising results.39,40
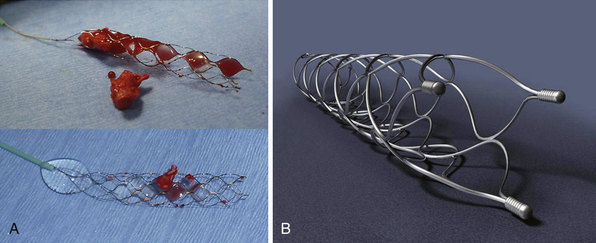
FIGURE 17.4 A, Solitaire FR device with retrieved clot (actual size). B, Solitaire FR.
(A from Natarajan SK, Siddiqui AH, Hopkins LN, Levy EI. Retrievable, detachable stent-platform-based clot-retrieval device (Solitaire FR) for acute stroke revascularization: first demonstration of feasibility in a canine stroke model. Vasc Dis Management 2010;7:E120-E125; B courtesy of ev3, Irvine, CA.)
Perfusion Imaging for Complication Avoidance and Patient Selection
Endovascular revascularization is an invasive procedure and is associated with acceptable complications in light of the morbidity and mortality risks associated with a major stroke if recanalization is not achieved. Despite aggressive revascularization with mechanical therapies, only up to 45% of patients recover to an mRS score of 0 to 2 at 3 months, and there is approximately an 8% to 10% procedure-related risk of symptomatic ICH, a potentially detrimental complication.19–23,32,38 CT and MR perfusion imaging are utilized in attempts to differentiate the three zones of postischemia brain tissue that are critical for patient selection and complication avoidance, and thus the ability to discriminate among them with these modalities is envisioned to lead to an improvement in outcomes after endovascular stroke therapy in the future. The three zones are core (tissue that is dead), penumbra (tissue at risk of death if not revascularized/reperfused), and benign oligemia (tissue that will tolerate the reduced blood flow and will survive even without reperfusion, mainly dependent on collaterals). Currently, no imaging modality is capable of differentiating between penumbra and benign oligemia. MR-diffusion-weighted imaging and cerebral blood flow maps obtained with CT perfusion imaging are able to identify core tissue with acceptable false positive and false negative rates. At our center, we have found that core tissue in the basal ganglia region or a core occupying more than 30% of occluded territory presages a higher risk of symptomatic ICH after endovascular stroke intervention. Sophisticated and precise imaging is needed to identify these three territories after cerebral ischemia.
Intracranial Atherosclerosis Treatment
Approximately 8% to 10% of ischemic strokes are attributable to intracranial atherosclerosis.41,42 An estimated 40,000 to 60,000 new strokes per year in the United States are due to intracranial atherosclerosis.43 In a study of patients with intracranial stenosis undergoing repeat angiography at an average interval of 26.7 months, 40% of lesions had stabilized, 20% had regressed, and 40% had progressed.44 Stenosis progression, as detected by transcranial Doppler imaging, was an independent predictor of stroke recurrence.45
Need for Treatment
The most definitive study of symptomatic intracranial stenosis thus far is the prospective Warfarin versus Aspirin for Symptomatic Intracranial Disease (WASID) trial, which found an 11% to 12% first-year risk of ischemic stroke in territory attributable to the patient symptoms.46 The majority of strokes (73%) in WASID patients were in the territory of the stenotic artery.47 Patients in the Extracranial-Intracranial Bypass Study with MCA stenosis who were randomized to medical therapy had an annual ipsilateral ischemic stroke rate of 7.8%.48,49 In the prospective, nonrandomized Groupe d’Etude des Sténoses Intra-Crâniennes Athéromateuses symptomatiques (GESICA), 102 patients with more than 50% symptomatic intracranial stenosis had “optimal” medical therapy, with a follow-up period of 23.4 months.50 Among these patients, ipsilateral transient ischemic attack (TIA) occurred in 12.6% and ipsilateral stroke in 7.0%.
Endovascular Tools and Technique
The Wingspan Stent System with the Gateway percutaneous transluminal angioplasty balloon catheter was designed for the treatment of intracranial atherosclerotic stenosis and received FDA approval for this indication under the humanitarian exemption device provision in August 2005. Prestent dilation of the stenotic lesion is done with the angioplasty balloon and the stent, a self-expanding nitinol device, is then deployed (Fig. 17.5 and Video 17.3).
Wingspan Studies
U.S. Wingspan Registry
In the U.S. Wingspan registry,51–53 treatment with the stent system was attempted in 158 patients with 168 intracranial atheromatous lesions. Of these, 161 lesions were successfully treated (96.0%) during the first treatment session. Of the 168 lesions in which treatment was attempted, there were 9 (5.4%) major periprocedural neurological complications, 4 of which ultimately led to the death of the patient within 30 days of the procedure. The total periprocedural event rate was 12.5% (21 of 168 cases). Most postprocedure events (18 of 21) were related to definable (and potentially controllable) issues: early antiplatelet interruption (n = 6) and in-stent restenosis (ISR) (n = 13). Imaging follow-up was available for 129 treated lesions (75 anterior circulation, 54 posterior circulation). Thirty-six of 129 (27.9%) patients with imaging follow-up of treated lesions experienced ISR. Of these 36, 29 (80.6%) underwent target lesion revascularization (TLR) with angioplasty alone (n = 26) or angioplasty with restenting (n = 3). Post-Wingspan ISR was more common in patients younger than 55 years old (odds ratio = 2.6). This increased risk can be accounted for by a high prevalence of anterior circulation lesions in this population, specifically those affecting the supraclinoid segment. When patients of all ages were considered, much higher rates of both ISR (66.6% vs. 24.4%) and symptomatic ISR (40% vs. 3.9%) were associated with supraclinoid segment lesions, by comparison with all other locations. Of the 29 patients undergoing primary TLR, 9 required one intervention for recurrent ISR, for a total of 42 TLR interventions. Only one major complication, a postprocedural reperfusion hemorrhage, was encountered during TLR (complication rates: 2.4% per procedure; 3.5% per patient). Angiographic follow-up was available for 22 of 29 patients after primary TLR. Eleven of 22 (50%) patients demonstrated recurrent ISR at follow-up angiography. Subsequently, 9 of these patients have undergone multiple re-treatments (6 patients had two re-treatments each, 2 had three re-treatments each, and 1 had four re-treatments) for recurrent ISR.
The 12-month follow-up results were recently published.54 The average follow-up duration was 14.2 months with 110 patients having at least 12 months of follow-up. The cumulative rate of the primary endpoint (stroke or death within 30 days or ipsilateral stroke after 30 days) was 15.7% for all patients and 13.9% for patients with high-grade stenosis (70-99%). Thirteen ipsilateral strokes occurred after 30 days, and 3 of these patients died. Ten of 13 (76.9%) strokes occurred within the first 6 months of the stenting procedure, and no events occurred after 12 months. An additional 9 patients had ipsilateral TIA after 30 days. Most postprocedure events (86%) were related to in-stent restenosis (n = 12), interruption of antiplatelet therapy (n = 6), or both (n = 1).
Wingspan NIH Registry
In the Wingspan National Institutes of Health (NIH) registry,37 129 patients with symptomatic 70% to 99% intracranial stenosis were enrolled from 16 medical centers. The rate of technical success (stent placement across the target lesion with <50% residual stenosis) was 97%. The rate of any stroke, ICH, or death within 30 days or ipsilateral stroke beyond 30 days was 14% at 6 months. The rate of 50% or greater restenosis on follow-up angiography was 25% among 52 patients with follow-up. The NIH registry investigators published a posthoc analysis report of 158 of 160 patients who had successful placement for intracranial atherosclerotic lesions with 50% to 99% stenosis.55 The primary endpoint (any stroke or death within 30 days or stroke in the territory of the stented artery beyond 30 days and up to 6 months) occurred in 13.9%. In multivariable analysis, the primary endpoint was associated with posterior circulation stenosis (vs. anterior circulation) (hazard ratio [HR] 3.4, p = 0.018), stenting at low enrollment sites (<10 patients each) (vs. high enrollment site) (HR 2.8, p = 0.038), 10 or fewer days from qualifying event to stenting (vs. ≥10 days) (HR 2.7, p = 0.058), and stroke as a qualifying event (vs. TIA or other cerebral ischemic event, such as vertebrobasilar insufficiency) (HR 3.2, p = 0.064).