Chapter 36 Endocrine Disorders
Critical illness leads to widespread changes in the endocrine system; the extent of the changes depends on the severity and duration of the physiologic insult. These changes are similar in nature irrespective of whether the insult involves surgery, sepsis, trauma, or a major cardiovascular event. In this chapter the endocrine disorders that are commonly encountered in postoperative cardiac surgery patients are described and an approach to their management is provided. The stress response to surgery and cardiopulmonary bypass is discussed in Chapter 2.
HYPERGLYCEMIA
Hospitalized patients with hyperglycemia can be classified as follows1:
Adverse Effects of Hyperglycemia and Rationale for Treatment
The adverse effects of hyperglycemia are shown in Table 36-1. Treatment with insulin reverses these effects and improves outcome in both diabetic and nondiabetic patients. In a study of diabetic patients with acute myocardial infarction, intensive control of hyperglycemia for at least 3 months, with a target blood glucose level of lower than 180 mg/dl (10 mmol/l), reduced mortality rates for at least 3 years.2 In studies of diabetic patients undergoing cardiac surgery, glucose control with a target of lower than 150 mg/dl (8.3 mmol/l) during the first 2 postoperative days reduced the rates of sternal wound infection and overall mortality.3,4 Intensive insulin therapy with a target glucose level of lower than 110 mg/dl (6.1 mmol/l) in surgical intensive care patients—of whom approximately 60% had undergone cardiac surgery—led to a marked reduction in mortality rates, principally among patients staying in an ICU for more than 5 days.5 In this study, progressively worse outcomes were seen at higher glucose levels. In a medical ICU setting, the same authors reported a reduction in morbidity with tight glucose control and a benefit in mortality rates in patients whose ICU stay was longer than 3 days.6 This later study also reported a much higher rate of hypoglycemia than was seen in the surgical ICU study. In both trials, nutritional targets were met early, with a high proportion of patients receiving parenteral nutrition. This complicates the applicability of these results to centers with less aggressive feeding practices.
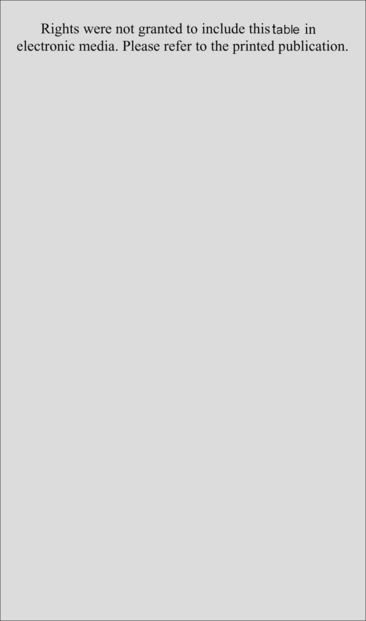
Table 36-1 Adverse Effects of Hyperglycemia
Rights were not granted to include this table in electronic media. Please refer to the printed book.
From Clement S, Braithwaite SS, Magee MF, et al: Management of diabetes and hyperglycemia in hospitals. Diabetes Care 27:553-591, 2004. TNF-α, tumor necrosis factor α; NFκB, nuclear factor kappa B.
Although there is no consensus on a threshold glucose level at which to start insulin in patients in an ICU, tighter glucose control is warranted with increasing severity of illness and anticipated length of ICU stay. Particular care should be taken with tight glucose control regimes to avoid hypoglycemia, especially in patients with variable or unreliable nutritional intakes. However, with appropriate education of staff and frequent bedside monitoring of blood glucose, tight control of blood glucose can be achieved. Except in the setting of myocardial ischemia and reperfusion (see subsequent material), it appears that the benefits arise from better control of hyperglycemia rather than from insulin per se.7,8
Treatment of Hyperglycemia
Oral Hypoglycemic Drugs
Oral hypoglycemic agents are not suitable for the control of hyperglycemia in the ICU. Sulfonylureas have a relatively long and unpredictable duration of action that risks hypoglycemia and hyperglycemia. Metformin reduces cardiovascular risk in outpatients9 but has been associated with potentially fatal lactic acidosis in patients at risk for renal dysfunction and tissue hypoxia,10,11 both of which are very common in the ICU. Thiazolidinediones have a slow onset of action and predispose to systemic edema.
Insulin
Numerous algorithms have been published to guide intravenous insulin dosing. The infusion rate should be determined by the blood glucose level, the rate of change of the blood glucose level, and some measure of insulin sensitivity. The last variable differs from patient to patient, and changes in the same patient at various times during an acute illness.3,5,12 An example of an insulin-infusion protocol that is based on the principles just outlined and that has been validated in the ICU environment is the Yale Protocol.12 A modified version of this protocol is provided in Table 36-1a.
Patients who are well enough to be transferred to the ward and who are eating regular meals can be switched from intravenous to subcutaneous bolus insulin. This may be given as three doses of short-acting insulin (before each meal) plus a dose of intermediate-acting insulin at night. The total daily dosage should be equivalent to the preceding day’s total intravenous dose, with 25% given as intermediate-acting insulin at night and 25% given in each of the three short-acting doses prior to meals. The insulin infusion should overlap with the first subcutaneous dose by 2 hours.1
Hypoglycemia
Symptoms of hypoglycemia may be masked in ICU patients, especially if they are sedated. Neurologic damage can occur when the blood glucose falls to less than 20 mg/dl (1.1 mmol/l). Levels lower than 50 mg/dl (2.8 mmol/l) should be treated with 50 ml of 50% dextrose (via a central vein), temporary cessation of the insulin infusion, and blood glucose testing every 15 minutes until return to stable normoglycemia. Factors likely to require a change in infusion rate should be anticipated. Insulin requirements are reduced by: (1) decreased nutrition delivery or absorption; (2) reduced infusion rates of β2-adrenoreceptor agonists; (3) the introduction of renal replacement therapy with dextrose-free fluid; (4) hepatic failure.6,13 Insulin requirements are increased with parenteral nutrition compared to an equivalent caloric amount of enteral nutrition.
Metabolic Support in Myocardial Ischemia using High-Dose Glucose-Insulin-Potassium Infusions
Myocardial Substrate Metabolism
As part of the stress response to surgery or myocardial ischemia, insulin activity is reduced and circulating levels of catecholamines, growth hormone, glucagon, and cortisol are increased (see Chapter 2). This results in increased myocardial utilization of free fatty acids and reduced utilization of glucose—despite hyperglycemia. In the absence of oxygen, the myocardium ceases to oxidize lactate and may become a net lactate producer (as the result of anaerobic glycolysis).14,15 These changes are potentially harmful to the ischemic myocardium. Increased reliance on free fatty acid metabolism leads to increased oxygen requirements, which may exacerbate ischemia. Ischemia-induced inhibition of β-oxidation leads to the accumulation of the toxic intermediates of fatty acid metabolism such as acyl CoA, which is associated with intracellular calcium overload and membrane instability. During postischemic reperfusion, myocardial fatty acid oxidation is poorly coupled with contractile function so that increased oxygen consumption is not associated with increased myocardial work. The net result is an increase in myocardial oxygen requirements, reduced contractility, and arrhythmias.
Insulin, given in doses sufficient to suppress circulating free fatty acid levels and optimize myocardial glucose uptake (≥ 0.1 units/kg/hr),16 protects ischemic myocardium and reduces reperfusion injury. Reduced mitochondrial fatty acid uptake and increased glucose uptake (with increased adenosine triphosphate [ATP] production resulting from glycolysis) improve membrane function and thereby reduce the risk for arrhythmias. Increased glucose oxidation and anaplerosis (replenishment of citric acid cycle substrates) reduce myocardial oxygen consumption and improve coupling between substrate oxidation and contractile function.
Glucose-Insulin-Potassium Infusions in Cardiac Surgery
High-dose infusions of glucose-insulin-potassium (GIK) have been reported to improve outcomes in patients with unstable angina undergoing urgent coronary artery bypass graft (CABG) surgery17 and in patients with refractory left ventricular failure following CABG surgery.18 The maximum benefit from perioperative high-dose GIK infusion is likely to be obtained if the infusion is commenced prior to the induction of anesthesia, continued for at least 12 hours postoperatively (to cover the period of reperfusion), and combined with tight glycemic control.19,20
Whether high-dose GIK improves outcome in patients with acute myocardial infarction, in addition to the benefit received from reperfusion therapy, is uncertain. Early small trials reported a benefit,21,22 but a more recent large, randomized trial in patients with ST-elevation myocardial infarction reported no benefit.23
One method of administering high-dose GIK is shown in Table 36-2. The principal risks in this technique are hypoglycemia and hyperkalemia, the latter after discontinuation of the infusions and in the presence of renal dysfunction. It is essential that the insulin and potassium supplements be stopped first, then the patient slowly weaned from the dextrose infusion (see Table 36-2).
Table 36-2 Protocol for High-Dose Glucose-Insulin-Potassium Infusion
Initiation of Infusion |
Infusion 1 |
50 units soluble insulin in 50 ml 0.9% saline at a fixed infusion rate of 0.1 units/kg/hr |
Infusion 2 |
500 ml 50% dextrose with 25 mmol KCl, 25 mmol KH2PO4, 20 mmol MgSO4 |
Infusion is commenced at 0.5 ml/kg/hr and adjusted to maintain the blood glucose at 100 to 150 mg/dl (5.6 to 8.3 mmol/l) |
Cessation of Infusions |
Infusion 1 |
Stop insulin infusion |
Infusion 2 |
Replace infusion of 50% dextrose + electrolytes with plain 50% dextrose |
Run at same rate as previous infusion |
Commence 5% dextrose at 1 ml/kg/hr |
Measure blood glucose every 1/2 hr and potassium hourly |
If blood glucose >250 mg/dl (13.9 mmol/l) reduce 50% dextrose by a half |
If blood glucose 200 to 250 mg/dl (11.1 to 13.9 mmol/l) reduce 50% dextrose by a quarter |
Stop 50% dextrose when infusion rate <5 ml/hr |
Continue to check blood glucose and serum potassium hourly for at least 6 hr |
Continue 5% dextrose (1 ml/kg/hr) for at least 6 hr |
DIABETES MELLITUS
About 30% of patients undergoing CABG surgery in the United States have diabetes.24 Diabetic patients undergoing cardiac surgery are more likely to have preexisting hypertension, renal insufficiency, previous myocardial infarction, congestive heart failure, or triple-vessel or left main coronary artery disease. Furthermore, they have higher hospital mortality rates and higher rates of postoperative complications than nondiabetic patients.25 Diabetic patients also have a severalfold higher risk for mortality following acute myocardial infarction as well as an increased incidence of congestive heart failure, reinfarction, and recurrent ischemia.2,11,26 Although the rate of death due to coronary heart disease has declined in the overall population over the past few decades, this decline has been less marked among diabetics.27 Furthermore, the incidences of insulin resistance and type 2 diabetes are increasing in North America.
Type 1 diabetes results from immunologic destruction of pancreatic β cells at a young age. Patients with type 1 diabetes are insulin deficient and require treatment with insulin to avoid ketoacidosis. Type 2 diabetes results from a combination of insulin resistance (due to obesity, physical inactivity, and genetic susceptibility) and an age-related decline in insulin secretion. Insulin resistance is often present for many years before type 2 diabetes becomes apparent. Increased insulin secretion compensates for insulin resistance at first, but as insulin secretion declines, hyperglycemia occurs, initially after meals or during periods of stress and later when fasting. Hyperglycemia can usually be controlled by diet initially but subsequently it is common for patients to require treatment with oral hypoglycemic drugs and eventually with insulin. Insulin resistance is associated with dyslipidemia, hypertension, and a prothrombotic tendency, each of which is an independent risk factor for coronary heart disease.11 Of patients with diabetes mellitus, 90% have type 2.
Diabetic Hyperglycemic Emergencies
The emergencies of diabetic ketoacidosis and a nonketotic hyperosmolar state can occur with both type 1 and type 2 diabetes.28,29 The underlying mechanism in both disorders is reduced circulating insulin with concurrent elevation of counterregulatory hormones, principally glucagon. Diabetic ketoacidosis occurs more commonly with type 1 diabetes and manifests as hyperglycemia and metabolic acidosis. Metabolic acidosis is caused by ketoacid formation resulting from fatty acid metabolism. Patients with type 2 diabetes are more likely to develop nonketotic hyperosmolar state, in which there is sufficient circulating insulin to prevent ketogenesis but insufficient to prevent hyperglycemia. Metabolic acidosis may develop in a nonketotic hyperosmolar state as the result of a combination of lactic acidosis (due to hypoperfusion), a low level of ketoacidosis (due to starvation), and inorganic acidosis (due to renal failure). Profound hyperosmolarity can lead to coma. Both conditions result in glycosuria, osmotic diuresis, and water and electrolyte depletion (Table 36-3). Dehydration tends to be more severe in the nonketotic hyperosmolar state. Common precipitants of both conditions include sepsis, acute coronary syndromes, glucocorticoids, sympathomimetics, and inadequate insulin therapy.
Table 36-3 Laboratory Features and Water and Electrolyte Deficits for Diabetic Ketoacidosis and Nonketotic Hyperosmolar State
DKA | NKHS | |
---|---|---|
Blood glucose (mg/dl) | >250 | >600 |
Serum osmolality (mOsm/l) | variable | >350 |
Arterial PH | <7.3 | >7.3 |
Serum bicarbonate (mmol/l) | <15 | >15 |
Ketones | moderate | small |
Anion gap (mmol/l) | >12 | variable |
Total Deficits | ||
Water (l) | 6 | 9 |
Sodium (mmol) | 500-700 | 350-900 |
Potassium (mmol) | 200-350 | 300-400 |
Phosphate (mmol) | 50-100 | 20-100 |
Magnesium (mEq) | 50-150 | 50-150 |
Calcium (mEq) | 50-150 | 50-150 |
DKA, diabetic ketoacidosis; L, liter; NKHS, nonketotic hyperosmolar state.
Data from Kitabachi AE, Umpierrez GE, Murphy MB, et al: Hyperglycemic crises in diabetes. Diabetes Care 27(suppl 1): S94-S102, 2004; Lebovitz HE: Diabetic ketoacidosis. Lancet 345:767-772, 1995.
Management of Diabetic Ketoacidosis and Nonketotic Hyperosmolar States
Fluids, insulin, and electrolytes are used to treat ketoacidosis and hyperosmolar states.28,29
Fluid Therapy
Severe hypovolemia should be treated by administering a rapid infusion of 1 to 2 liters of 0.9% saline. In the absence of marked hypovolemia 1 liter of 0.9% saline may be infused over the first hour followed by 500 ml/hr over the next 8 hours. Hartmann solution (see Table 32-3) may be used in place of 0.9% saline if there is profound acidosis. If, after the first hour, the corrected sodium (see Chapter 32) is greater than 150 mmol/l, 0.45% saline should be used in place of 0.9% saline.
ADRENOCORTICAL DISORDERS
Normal Adrenal Glucocorticoid Function
Although cortisol has some mineralocorticoid (aldosterone-like) effects, its predominant action is on intermediary metabolism. Cortisol raises blood glucose directly by promoting gluconeogenesis and glycogenolysis and indirectly by suppressing insulin. Protein catabolism and lipolysis are increased. The cardiovascular effects of cortisol include maintaining vascular tone, endothelial integrity, and cardiac contractility. Cortisol also potentiates the vasoconstrictor and inotropic effects of catecholamines. Cortisol modulates the balance of pro- and antiinflammatory mediators by means of a net antiinflammatory and immunosuppressive effect.30
Adrenocortical Stress Response
Any stressful stimulus, such as pain, cold, fever, hypovolemia, surgery, trauma, or inflammation, results in a sustained increase in corticotropin and cortisol secretion, with loss of diurnal variation. The magnitude and duration of this response is proportional to the severity of the stimulus. Following major surgery, peak plasma cortisol levels are approximately 30 μg/dl (828 nmol/l); return to basal levels (approximately 14 μg/dl [386 nmol/l]) occurs over the subsequent 72 hours.31 With severe illness or injury, the plasma cortisol may be closer to 50 μg/dl (1380 nmol/l), and can be as high as 200 μg/dl (5520 nmol/l), and remain elevated for many days.32 In addition, corticosteroid-binding globulin levels decrease, and cortisol is cleaved from corticosteroid-binding globulin by neutrophil elastase, which results in increased free cortisol levels.
Clinical Features of Relative Adrenal Insufficiency
Relative adrenal insufficiency most commonly manifests as vasopressor-dependent hypotension,30,33 difficulty in weaning from ventilatory support,34 or persistent inflammation. The hemodynamic state may mimic both septic shock, with high cardiac output and vasodilation, and hypovolemic shock, with depressed cardiac contractility, decreased preload, and vasoconstriction. Most of the clinical manifestations of chronic adrenal insufficiency are nonspecific. However, the presence of hypoglycemia or eosinophilia, which is uncommon in the ICU, should raise suspicion of adrenal insufficiency. Hyponatremia and hyperkalemia may be present, but they are more commonly due to other causes or masked by intravenous fluid therapy (see Chapter 32).35 Adrenal insufficiency may predispose to increased blood loss in patients undergoing CABG surgery.36
Causes of Relative Adrenal Insufficiency
Circulating cytokines cause relative adrenal insufficiency in 30% to 60% of patients with severe sepsis or septic shock or after major surgery.33,37 Destructive processes directly affecting the adrenal glands include adrenal or retroperitoneal hemorrhage, metastatic malignancy, or systemic infection (HIV, tuberculosis, cytomegalovirus, or fungal infection). The anesthetic agent etomidate and the antifungal ketoconazole inhibit enzymes involved in cortisol synthesis. Phenytoin and rifampicin enhance hepatic metabolism of cortisol.
Diagnostic Tests for Relative Adrenal Insufficiency
An ideal test of adrenal function would identify patients who would benefit from treatment with corticosteroids in terms of earlier recovery of organ function and improved survival rates.37 Unfortunately, no such test exists. Current tests (Table 36-4) are based on the measurement of total plasma cortisol, which may not be reflective of free cortisol and does not take into account tissue resistance. In critically ill patients, the adrenal axis should be maximally stimulated, thus negating the rationale for corticotropin-stimulation tests in patients in the ICU. However, the combination of baseline and stimulated plasma cortisol levels may have more prognostic significance.35
Table 36-4 Diagnostic Tests for Relative Adrenal Insufficiency
Test | Result | Comments |
---|---|---|
Random plasma total cortisol | Value less than 25 μg/dl (<690 nmol/l)37 |
CABG, coronary artery bypass graft
Treatment of Relative Adrenal Insufficiency
Older studies of short-term, high-dose glucocorticoid therapy showed no reduction in mortality rates in patients with severe sepsis or septic shock. More recent studies have shown that a longer course (>7 days) of lower, “stress” doses of glucocorticoids reduce vasopresssor requirements, shorten the duration of ventilation, and may improve survival rates in patients with laboratory evidence of adrenal insufficiency.31,33,34,38,39 Stress dose glucocorticoid therapy is not associated with an increased risk for infection.
Specific mineralocorticoid replacement by fludrocortisone is not necessary if more than 50 mg daily of hydrocortisone is given. Even with dexamethasone (which has minimal mineralocorticoid activity), administration of 0.9% saline eliminates the need for separate mineralocorticoid therapy. Various glucocorticoids are compared in Table 36-5.
Acute Adrenal Crisis
Acute, complete loss of adrenal function is rare and is usually caused by hemorrhage or infarction of the adrenal glands, most often in patients already severely ill with thromboembolic disease, coagulopathy, or shock. Patients present with acute circulatory failure, sometimes accompanied by abdominal or flank pain. Biochemical derangement is uncommon.35 The diagnosis is confirmed by a random plasma cortisol level of less than 3 μg/dl (80 nmol/l) and no response to a corticotropin stimulation test. Treatment involves intravenous hydrocortisone (100-mg bolus followed by a 10 mg/hr infusion or 100 mg every 6 hr) and fluid resuscitation with 0.9% saline. Intravenous glucose may be required to prevent hypoglycemia. If testing is delayed, intravenous dexamethasone (4 mg every 12 hr), which does not interfere with the cortisol assay, can be used initially in place of hydrocortisone.
THYROID DISORDERS
Cardiovascular Effects of Hyperthyroidism
An excess of thyroid hormone causes vasodilation, increased cardiac output, and tachycardia.40 Blood pressure may fall. Atrial fibrillation may occur, even with subclinical hyperthyroidism. Reduced blood pressure leads to sodium and water retention. Heart failure may develop as a result of tachycardia (or atrial fibrillation) or sodium and water retention or because of an impaired ability to respond to the increased metabolic rate that is associated with hyperthyroidism (high-output heart failure).
Diagnosis and Treatment of Hyperthyroidism
The changes in T3, T4, and thyrotropin that occur with primary (thyroid) and secondary (pituitary or hypothalamic) hyperthyroidism are shown in Table 36-6. Treatment should include a β blocker for control of tachycardia. Propranolol (40 to 120 mg/day in divided doses orally) is the β blocker of choice because of its rapid onset of action and ability to inhibit peripheral conversion of T4 to T3. Thyroid hormone production can be reduced by antithyroid drugs (e.g., carbimazole), radioactive iodine, or surgery. Treatment of hyperthyroidism usually results in spontaneous reversion of atrial fibrillation to sinus rhythm. Electrical or pharmacologic cardioversion may be successful only after the patient has been rendered euthyroid. With severe hyperthyroidism, intravenous hydrocortisone (50 to 100 mg every 6 hr) should also be given because it reduces the peripheral conversion of T4 to T3.
Cardiovascular Effects of Hypothyroidism
The cardiovascular manifestations of hypothyroidism40 are bradycardia, reduced myocardial contractility, increased systemic resistance, and mild hypertension. Despite reduced contractility, heart failure is uncommon because the metabolic rate is reduced. There may be prolongation of the cardiac action potential, manifested as a prolonged QT interval, which predisposes to ventricular arrhythmias (see Chapter 21). With severe hypothyroidism, there may be nonpitting edema (myxedema), pericardial effusion, and elevated plasma concentrations of cholesterol and creatine kinase.
Diagnosis and Treatment of Hypothyroidism
The changes in T3, T4, and thyrotropin that occur with hypothyroidism are shown in Table 36-6. Treatment with thyroxine reverses all of the cardiovascular effects of hypothyroidism. Young patients who do not have other cardiac disease can be given full replacement doses (100 to 150 μg orally once daily) of thyroxine at the outset. Older patients and those with ischemic heart disease should initially be given 25 μg daily, with a gradual increase in dosage in increments of 25 μg every month, up to the full replacement dosage. In suspected secondary (pituitary or hypothalamic) hypothyroidism, intravenous hydrocortisone (100 mg every 6 hr) should be given prior to starting thyroxine because acute adrenal insufficiency may be precipitated by thyroxine-induced increased metabolic rate.
Amiodarone and Thyroid Function
Amiodarone40 inhibits the peripheral conversion of T4 to T3, leading to a 25% fall in T3 concentration and a slight rise in T4 and thyrotropin concentrations. Because of its high iodine content, amiodarone can also inhibit the synthesis and secretion of T3 and T4. Amiodarone causes hypothyroidism in 5% to 25% of patients who are treated chronically with the drug. Thyroid function tests should be measured every few months, and thyroxine therapy should be instituted if indicated amiodarone does not have to be discontinued.
Sick Euthyroid Syndrome
Sick euthyroid syndrome40 is a condition characterized by low plasma levels of T3. Plasma levels of T4 and thyrotropin are usually normal but with severe forms of the syndrome are also reduced (see Table 36-6). Thus, the biochemical picture may resemble secondary hypothyroidism, that is, hypothyroidism due to pituitary failure, which is very rare. Sick euthyroid syndrome occurs in association with severe systemic illness, including acute myocardial infarction, heart failure, and cardiac surgery. It also develops within 24 hours of a fast. The main pathophysiologic mechanism of the syndrome is reduced peripheral conversion of T4 to T3. In addition, thyroid hormone binding globulin and albumin levels may be decreased, and the tissue uptake and intracellular effects of T3 and T4 may be reduced.
Following cardiac surgery, levels of free and total T3 typically decline by 50% to 75% for 1 to 4 days. Patients may have reduced myocardial contractility and increased peripheral vascular resistance similar to that seen with hypothyroidism, although clinically these findings may be difficult to distinguish from the underlying illness. Treatment of sick euthyroid syndrome with T4 or T3 does not improve outcome41 and is not recommended.
Triiodothyronine and Cardiac Surgery
The rationale for using thyroid hormones following cardiac surgery42 is primarily to improve cardiac performance rather than to correct hypothyroidism or sick euthyroid syndrome. Treatment with intravenous T3 during the intraoperative and postoperative periods increases cardiac output, reduces systemic vascular resistance, has an inotrope-sparing effect, and may reduce the incidence of atrial fibrillation.43,44 However, a reduction in postoperative morbidity and mortality rates has not been demonstrated.43
OTHER HORMONES
Growth Hormone
In critically ill patients, growth hormone secretion becomes nonpulsatile and has variable circulating levels. Treatment with high-dose growth hormone is detrimental for patients in the ICU,45 but physiologic doses may improve hemodynamics and functional status in patients with idiopathic dilated cardiomyopathy.46 Currently, there is no role for the routine use of growth hormone in patients after cardiac surgery.
Vasopressin (Antidiuretic Hormone)
In normal subjects, the primary role of vasopressin is to maintain water homeostasis (see Chapters 1 and Chapter 32). However, in response to hypotension resulting from hemorrhage or sepsis, vasopressin secretion acts as a potent vasoconstrictor by potentiating the effects of circulating catecholamines, by inactivating vascular smooth muscle potassium-ATP channels, and by blunting the vasodilatory effects of nitric oxide. During severe vasodilatory shock, vasopressin stores rapidly become depleted. Low-dose vasopressin infusion (0.04 μg/min) can restore blood pressure in such patients despite catecholamine resistance (see Chapters 20 and Chapter 35). Its role in brain-dead organ donors is discussed in Chapter 38.
1 Clement S, Braithwaite SS, Magee MF, et al. Management of diabetes and hyperglycemia in hospitals. Diabetes Care. 2004;27:553-591.
2 Malmberg K. Prospective randomised study of intensive insulin treatment on long term survival after acute myocardial infarction in patients with diabetes mellitus. DIGAMI (Diabetes Mellitus, Insulin Glucose Infusion in Acute Myocardial Infarction) Study Group. BMJ. 1997;314:1512-1515.
3 Furnary AP, Gao G, Grunkemeier GL, et al. Continuous insulin infusion reduces mortality in patients with diabetes undergoing coronary artery bypass grafting. J Thorac Cardiovasc Surg. 2003;125:1007-1021.
4 Furnary AP, Zerr KJ, Grunkemeier GL, et al. Continuous intravenous insulin infusion reduces the incidence of deep sternal wound infection in diabetic patients after cardiac surgical procedures. Ann Thorac Surg. 1999;67:352-360.
5 van den Berghe G, Wouters P, Weekers F, et al. Intensive insulin therapy in the critically ill patients. N Engl J Med. 2001;345:1359-1367.
6 van den Berghe G, Wilmer A, Hermans G, et al. Intensive insulin therapy in the medical ICU. N Engl J Med. 2006;354:449-461.
7 van den Berghe G, Wouters PJ, Bouillon R, et al. Outcome benefit of intensive insulin therapy in the critically ill: insulin dose versus glycemic control. Crit Care Med. 2003;31:359-366.
8 Finney SJ, Zekveld C, Elia A, et al. Glucose control and mortality in critically ill patients. JAMA. 2003;290:2041-2047.
9 UK Prospective Diabetes Study Group. Effect of intensive blood-glucose control with metformin on complications in overweight patients with type 2 diabetes (UKPDS 34). UK Prospective Diabetes Study (UKPDS) Group. Lancet. 1998;352:854-865.
10 Lalau JD, Lacroix C, de Cagny B, et al. Metformin-associated lactic acidosis in diabetic patients with acute renal failure: a critical analysis of its pathogenesis and prognosis. Nephrol Dial Transplant. 1994;9(suppl 4):126-129.
11 Clement S, Braithwaite SS, Magee MF, et al. Management of diabetes and hyperglycemia in hospitals. Diabetes Care. 2004;27:553-591.
12 Goldberg PA, Siegel MD, Sherwin RS, et al. Implementation of a safe and effective insulin infusion protocol in a medical intensive care unit. Diabetes Care. 2004;27:461-467.
13 Vriesendorp TM, van Santen S, DeVries JH, et al. Predisposing factors for hypoglycemia in the intensive care unit. Crit Care Med. 2006;34:96-101.
14 Stanley WC, Chandler MP. Energy metabolism in the normal and failing heart: potential for therapeutic interventions. Heart Fail Rev. 2002;7:115-130.
15 Carvajal K, Moreno-Sanchez R. Heart metabolic disturbances in cardiovascular diseases. Arch Med Res. 2003;34:89-99.
16 Stanley AWJr, Moraski RE, Russell RO, et al. Effects of glucose-insulin-potassium on myocardial substrate availability and utilization in stable coronary artery disease: studies on myocardial carbohydrate, lipid and oxygen arterial-coronary sinus differences in patients with coronary artery disease. Am J Cardiol. 1975;36:929-937.
17 Lazar HL, Philippides G, Fitzgerald C, et al. Glucose-insulin-potassium solutions enhance recovery after urgent coronary artery bypass grafting. J Thorac Cardiovasc Surg. 1997;113:354-360.
18 Gradinac S, Coleman GM, Taegtmeyer H, et al. Improved cardiac function with glucose-insulin-potassium after aortocoronary bypass grafting. Ann Thorac Surg. 1989;48:484-489.
19 Lazar HL, Chipkin SR, Fitzgerald CA, et al. Tight glycemic control in diabetic coronary artery bypass graft patients improves perioperative outcomes and decreases recurrent ischemic events. Circulation. 2004;109:1497-1502.
20 Carvalho G, Moore A, Qizilbash B, et al. Maintenance of normoglycemia during cardiac surgery. Anesth Analges. 2004;99:319-324.
21 Diaz R, Paolasso EA, Piegas LS, et al. Metabolic modulation of acute myocardial infarction. The ECLA (Estudios Cardiologicos Latinoamerica) Collaborative Group. Circulation. 1998;98:2227-2234.
22 Fath-Ordoubadi F, Beatt KJ. Glucose-insulin-potassium therapy for treatment of acute myocardial infarction: an overview of randomized placebo-controlled trials. Circulation. 1997;96:1152-1156.
23 Mehta SR, Yusuf S, Diaz R, et al. Effect of glucose-insulin-potassium infusion on mortality in patients with acute ST-segment elevation myocardial infarction: the CREATE-ECLA randomized controlled trial. JAMA. 2005;293:437-446.
24 Christenson JT, Cohen M, Ferguson JJr, et al. Trends in intraaortic balloon counterpulsation complications and outcomes in cardiac surgery. Ann Thorac Surg. 2002;74:1086-1090.
25 Shroyer AL, Coombs LP, Peterson ED, et al. The Society of Thoracic Surgeons: 30-day operative mortality and morbidity risk models. Ann Thorac Surg. 2003;75:1856-1864. discussion 1864-5
26 Capes SE, Hunt D, Malmberg K, et al. Stress hyperglycaemia and increased risk of death after myocardial infarction in patients with and without diabetes: a systematic overview. Lancet. 2000;355:773-778.
27 Gu K, Cowie CC, Harris MI. Diabetes and decline in heart disease mortality in US adults. JAMA. 1999;281:1291-1297.
28 Kitabchi AE, Umpierrez GE, Murphy MB, et al. Hyperglycemic crises in diabetes. Diabetes Care. 2004;27(suppl 1):S94-S102.
29 Lebovitz HE. Diabetic ketoacidosis. Lancet. 1995;345:767-772.
30 Keh D, Boehnke T, Weber-Cartens S, et al. Immunologic and hemodynamic effects of “low-dose” hydrocortisone in septic shock: a double-blind, randomized, placebo-controlled, crossover study. Am J Respir Crit Care Med. 2003;167:512-520.
31 Rivers EP, Gaspari M, Saad GA, et al. Adrenal insufficiency in high-risk surgical ICU patients. Chest. 2001;119:889-896.
32 Lamberts SW, Bruining HA, de Jong FH. Corticosteroid therapy in severe illness. N Engl J Med. 1997;337:1285-1292.
33 Annane D, Sebille V, Charpentier C, et al. Effect of treatment with low doses of hydrocortisone and fludrocortisone on mortality in patients with septic shock. JAMA. 2002;288:862-871.
34 Huang CJ, Lin HC. Association between adrenal insufficiency and ventilator weaning. Am J Respir Crit Care Med. 2006;173:276-280.
35 Cooper MS, Stewart PM. Corticosteroid insufficiency in acutely ill patients. N Engl J Med. 2003;348:727-734.
36 Henzen C, Kobza R, Schwaller-Protzmann B, et al. Adrenal function during coronary artery bypass grafting. Eur J Endocrinol. 2003;148:663-668.
37 Marik PE, Zaloga GP. Adrenal insufficiency during septic shock. Crit Care Med. 2003;31:141-145.
38 Briegel J, Forst H, Haller M, et al. Stress doses of hydrocortisone reverse hyperdynamic septic shock: a prospective, randomized, double-blind, single-center study. Crit Care Med. 1999;27:723-732.
39 Annane D, Bellissant E, Bollaert PE, et al. Corticosteroids for severe sepsis and septic shock: a systematic review and meta-analysis. BMJ. 2004;329:480.
40 Klein I, Ojamaa K. Thyroid hormone and the cardiovascular system. N Engl J Med. 2001;344:501-509.
41 Brent GA, Hershman JM. Thyroxine therapy in patients with severe nonthyroidal illnesses and low serum thyroxine concentration. J Clin Endocrinol Metab. 1986;63:1-8.
42 Klemperer JD. Thyroid hormone and cardiac surgery. Thyroid. 2002;12:517-521.
43 Klemperer JD, Klein I, Gomez M, et al. Thyroid hormone treatment after coronary-artery bypass surgery. N Engl J Med. 1995;333:1522-1527.
44 Mullis-Jansson SL, Argenziano M, Corwin S, et al. A randomized double-blind study of the effect of triiodothyronine on cardiac function and morbidity after coronary bypass surgery. J Thorac Cardiovasc Surg. 1999;117:1128-1134.
45 Takala J, Ruokonen E, Webster NR, et al. Increased mortality associated with growth hormone treatment in critically ill adults. N Engl J Med. 1999;341:785-792.
46 Fazio S, Sabatini D, Capaldo B, et al. A preliminary study of growth hormone in the treatment of dilated cardiomyopathy. N Engl J Med. 1996;334:809-814.
47 Siraux V, de Backer D, Yalavatti G, et al. Relative adrenal insufficiency in patients with septic shock: comparison of low-dose and conventional corticotropin tests. Crit Care Med. 2005;33:2479-2486.
48 Hamrahian AH, Oseni TS, Arafah BM. Measurements of serum free cortisol in critically ill patients. N Engl J Med. 2004;350:1629-1638.