CHAPTER 12 Emergency Nuclear Radiology
RADIONUCLIDES
Technetium-99m
Tc-99m is the most widely used tracer in nuclear medicine, accounting for over 85% of routine diagnostic procedures in a nuclear medicine department. The following radiation characteristics of technetium-99m make it an ideal agent (Fig. 12-1).
Tc-99m is obtained from “milking” a molybdenum-99 (Mo-99) generator (Fig. 12-2). Mo-99 is obtained as a by-product from the fission of uranium. Mo-99 is chemically separated from the other radionuclides in the reactor product. Purified Mo-99 as anionic molybdate solution is loaded on to the generator column, which contains alumina. The alumina, which is positively charged, is able to adsorb Mo-99 ions. The assembly is then autoclaved. Several normal saline washings of the column yield eluate containing Tc-99m. These washings are subjected to several quality control tests to determine eluate volume, radionuclide purity (“moly” breakthrough), radiochemical purity (proper chemical form of technetium as pertechnetate), pyrogenicity, sterility, and/or alumina breakthrough. The half-life of Mo-99 is 66 hours allowing for weekly delivery of the generator to elute Tc-99m.
The eluted technetium can now be tagged to the appropriate pharmaceutical for use. When radionuclides such as technetium are tagged to specific pharmaceuticals, they are called radiopharmaceuticals. The pharmaceutical portion of the diagnostic radiopharmaceutical is present in very small amounts and will not elicit any pharmacologic response in the patient. The radioactive component is present in even smaller amounts. The technetium-99m radiopharmaceuticals for use in the emergency situation are listed in Box 12-1.
Indium-111
In-111 is produced in a cyclotron from the radioactive parent Cd-112. It has a half-life of 67 hours (2.8 days). The principal photons are 178 keV and 247 keV. The relatively long half-life of In-111 allows sequential imaging (Fig. 12-3).
Gallium-67 Citrate
Gallium-67 is produced in a cyclotron from the parent zinc-68. Gallium-67 decays by electron capture to stable zinc-67 in 3.26 days (Fig. 12-4). The transition energy, which is 0.997 MeV, is dissipated by several electron capture transitions. Several gamma photons are emitted that are used for imaging. The principal ones are 93 keV with 37% abundance, 185 keV with 20% abundance, 300 keV with 17% abundance, and 394 keV with 5% abundance.
IMAGING EQUIPMENT
The components of the detection system/gamma camera (Fig. 12-5) include the following:
LUNG SCINTIGRAPHY
Radiopharmaceuticals and Techniques
Perfusion
The patient is imaged in a sitting position, although images could be obtained with the patient supine. Ideally, the patient is imaged on a large field-of-view (FOV) gamma camera using a parallel-hole collimator. A diverging collimator may be necessary in larger patients to encompass both lungs on the anterior and posterior views. The standard views are the anterior, posterior, right and left laterals, both right and left posterior oblique, and both right and left anterior oblique (Fig. 12-6). Generally, a minimum of 500,000 counts is accumulated per image.
Ventilation
Xenon-133
Xenon can be used to assess all phases of ventilation. The most commonly used technique involves having the patient breathe xenon through a spirometer. The patient exhales as deeply as possible and then inhales 10 to 20 mCi of Xe-133. The respiration is suspended at the end of the inhalation for 15 seconds, while the first image is obtained. The patient breathes xenon out into a spirometer, which constitutes a closed system. Approximately 2 L of oxygen are used to dilute the expired xenon (Fig. 12-7). The patient rebreathes this mixture for 2 to 3 minutes, at which time another static image is obtained. This constitutes the equilibrium image. After equilibrium has been reached, fresh air is breathed in until the xenon is completely washed out. Images are obtained every 15 seconds for 2 to 3 minutes. For patients with chronic obstructive pulmonary disease (COPD), the washout phase may be delayed up to 5 minutes to image areas of regional airway trapping. This entire process presupposes that the patient is able to cooperate with breathing into a spirometer or a closed system. The initial/single breath reflects the regional ventilatory rate. The equilibrium phase depicts the aerated volume of the lungs, while the washout phase delineates trapping. Xenon is fat soluble and partially soluble in blood, which will cause deposition in the liver, particularly in patients with fatty replacement in the liver.
Radiolabeled Aerosols
In the workup for PE, Tc-99m DTPA aerosol is ideal, since images can be obtained in projections to match the ventilation images. After inhalation, aerosol particles are deposited in the distal airways and not the alveoli. Following inhalation, the Tc DTPA particles dissolve in the fluids within the alveoli and ultimately diffuse across the epithelial barrier into the circulation. As long as the epithelial barrier is intact, the aerosol diffuses relatively slowly into the circulation. The half-time disappearance of the aerosol from the alveoli is about 80 minutes. This is much faster in patients whose epithelial barrier may be deficient, as in COPD or in smokers. The Tc DTPA that has entered into the circulation is cleared via the kidneys. Larger particles are deposited in the central airways, the mouth, and the alimentary tract (from swallowed particles) (Fig. 12-8).
Chest X-Ray
It is important to have a chest x-ray for evaluation before performing the ventilation-perfusion (VQ) scan (Fig. 12-9). It is good practice to have one that has been obtained within 24 hours of performing the VQ scan. It would be ideal to have a full-inspiration posteroanterior (PA) and lateral chest x-ray available for interpretation. However, a large percentage of the patients who are at risk for PE in the hospital or the intensive care unit setting may have several other cardiopulmonary pathologic processes that could interfere with the reading of the VQ scan. In these patients, one has to be satisfied with the portable radiograph, which is rarely of the quality of the standard PA and lateral radiographic study.
The first step is to obtain a chest x-ray for comparison with the VQ scans.
A high-probability scan has two or more large, mismatched segmental defects (or equivalents in moderate/large defects) with no abnormality on the ventilation study (Fig. 12-10). In the clinical setting where PE is highly likely, a high-probability VQ scan indicates a probability of PE greater than 90%. If the mismatched perfusion defects should resolve within days or weeks, the probability of recent embolism is higher.
A low-probability scan is one in which the perfusion scans are smaller than 25% of a segment regardless of the ventilation scan or chest x-ray appearance, matched on ventilation scan, and accompanied by larger radiographic abnormalities (Fig. 12-11).
An intermediate-probability scan is one that does not fit into the high or low categories.
BRAIN DEATH
Confirmation of absent intracranial perfusion offers confirmation of brain death. This can be obtained by demonstrating absence of intracranial perfusion and absence of sagittal sinus activity following intravenous administration of tracer. Occasionally the sagittal sinus may be perfused from the external carotid circulation (Fig. 12-12).
Interpretation
Tomographic SPECT images allow better visualization of activity distribution within the skull, and allow one to see brainstem and cerebellar activity that is hard to see with planar imaging shown above. Figure 12-13 shows the same patient as in Figure 12-12 with SPECT imaging clearly demonstrating activity in the brainstem and cerebellum. The cerebellar and brainstem activity usually clears in 24 to 36 hours and confirms diagnosis of brainstem death as well. The patient shown in Figures 12-12 and 12-13 showed no activity in the cerebellum or brainstem when study was repeated after 20 hours.
INFECTION
Leukocyte Labeling
Indium-Labeled Leukocytes
With indium-111–labeled WBC studies, images are obtained 24 hours after injection, by which time the pulmonary activity would have already cleared. It is important to note that the 24-hour time lag required for indium-labeled infection imaging would remove it from consideration as a “true emergency.” At 24 hours following injection, there is normal distribution through the spleen, liver, and bone marrow (Fig. 12-14).
Technetium-Labeled Leukocytes
A low-energy, all-purpose, high-resolution collimator is used with a 15% window centered on the 140-keV photopeak of Tc-99m. Granulocyte labeling is selective with the Tc-99m HMPAO complex. Urinary activity and renal parenchymal activity appear soon after injection. Biliary activity is seen in a variable number of patients as early as 3 hours following injection. This accounts for bowel activity appearing at 4 hours. Bone marrow uptake is also seen with Tc-99m–labeled leukocytes, although none is seen in normal bone. Images are routinely obtained at 2 and 4 hours post injection, although 45-minute and 24-hour images may also be obtained if needed (Fig. 12-15).
Technetium Methylene Diphosphonate
Tc-99m methylene diphosphonate (MDP) is one of the agents of choice for evaluation of osteomyelitis. This is the agent used for the triple-phase bone scan. It is injected intravenously, and flow/perfusion studies of the affected part are obtained at one image per second for a total of 30 seconds. For a perfusion study, ideally a high-sensitivity collimator is used. This is followed by the acquisition of “blood pool” images or immediate static images of the area in question. Two to four hours after intravenous administration of the Tc-99m MDP, delayed static images are acquired for 500 K counts per projection utilizing a high-resolution collimator (Fig. 12-16). In smaller nuclear medicine departments it is not uncommon to use a LEAP collimator for both the perfusion and the static images.
Abdominal Abscesses
The most common causes of intra-abdominal abscesses include the following:
There are six functional compartments within the peritoneal cavity:
Musculoskeletal Infections
The major problems of imaging for osteomyelitis with WBC-labeled studies are that (1) osteomyelitis is frequently more chronic and (2) WBCs normally accumulate in the bone marrow where they are destroyed by the reticuloendothelial system. In the imaging of a prosthesis, nuclear medicine imaging is not affected by the prosthesis, whereas the prosthesis is a deterrent to imaging with any of the other imaging modalities. Bone scintigraphy is useful, in that a negative scan essentially excludes a prosthetic complication. Adding Ga-67 citrate increases the accuracy of bone scintigraphy. The combination of leukocyte labeling combined with marrow imaging is believed to have the highest accuracy of all the imaging studies available (Fig. 12-17).
TRAUMA AND BONE INFARCTION
GASTROINTESTINAL BLEEDING
Radiopharmaceuticals
The two agents that are used for detection of the source of GI bleeding are Tc-99m–labeled RBCs (Fig. 12-18) and Tc-99m sulfur colloid. Neither of these agents is ideal, and each agent has advantages and disadvantages that the other may not possess.
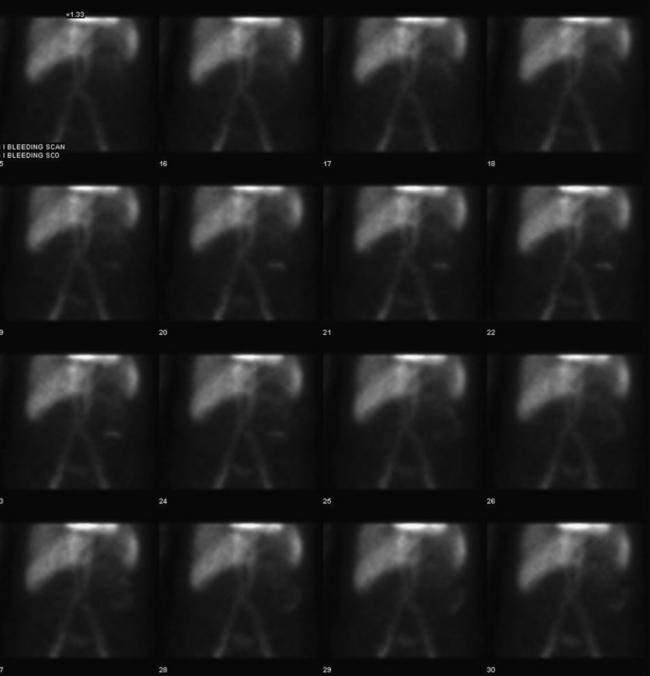
Figure 12-18 Tc-99m RBC study shows significant progressive accumulation of activity in the descending colon due to bleeding.
HEPATOBILIARY SCINTIGRAPHY
Acute Cholecystitis
The vast majority of patients with acute cholecystitis have cystic obstruction. It is based on this fact that hepatobiliary scintigraphy is used for the diagnosis of acute cholecystitis. Absence of visualization of the gallbladder for up to 4 hours after injection of the radiotracer is considered diagnostic for acute cholecystitis (Fig. 12-19). The appearance of the tracer in the gallbladder effectively excludes the presence of acute cholecystitis. However, in some cases of acalculous cholecystitis, there may be filling of the gallbladder. Additionally, the presence of an accessory cystic duct can cause a filling of the gallbladder through an “alternate” route. Structures that simulate a gallbladder can produce false negatives; these include duodenal diverticula and biliary duplication cysts.
Intravenous administration of morphine (0.04 mg/kg diluted in 10 mL of normal saline administered over 5 minutes) causes constriction of the sphincter of Oddi, raising intraductal pressure and forcing the filling of the gallbladder (Fig. 12-20). Occasionally there may not be enough tracer in the liver or the biliary tree to fill the gallbladder. This might necessitate a second injection of the tracer. Administration of morphine is a measure that should be used with caution where there is obstruction to the proximal portion of the cystic duct, as evidenced by the “cystic duct” sign. Enough pressure may be generated in the system to force the tracer into the gallbladder, producing a false negative study.
There are certain ancillary signs that are of use in the diagnosis of cholecystitis:
Tc-99m HIDA/DISIDA scans are of paramount importance in the detection of biliary leaks, postsurgical or post-traumatic (Fig. 12-21). These agents are highly sensitive in detecting biliary leaks, which are not seen with other modalities. Additional views of the paracolic gutter and the pelvis must be obtained. A thorough knowledge of the anatomy of the peritoneal spaces is useful in helping the surgeon with the postoperative care of the patient.
SCROTAL SCANNING
Interpretation
Testicular Torsion
In the early stage of torsion, the perfusion to the affected side may be normal. A “nubbin” sign is believed to be pathognomonic for torsion and represents the site of the actual torsion of the testicular vessels. This is believed to be due to visualization of the spermatic artery, which is being filled by the iliac artery (Fig. 12-22). In the later stages of the torsion, that is, 24 hours later, the relative avascular testis is thrown into high relief by the increased flow through the pudendal vessels to the scrotal sac. The now perfused scrotal sac with the absent vascularity in the testis provides a “Hello” sign.
Bar-shalom R., Yefremov N., Guralnik L., et al. SPECT/CT using Ga 67 and 111 in labeled leukocyte scintigraphy for diagnosis of infection. J Nucl Med. 2006;47:587-594.
Burke J: Advances in 99mTc radiopharmaceutical chemistry. Paper presented at the 148th annual meeting of the APA, San Francisco, March 2001.
Carvalho PA, Anthony PJ: Evaluation of GI bleeding with Tc 99m RBC. http://www.jpnm.org
Curtin K., Fitzgerald S.W., Nemcek A.A.Jr., et al. CT diagnosis of acute appendicitis: Imaging findings. AJR Am J Roentgenol. 1995;164:905.
Ell P.J., Gambhir S.S. Nuclear Medicine in Clinical Diagnosis and Treatment, 3rd ed. Philadelphia: Churchill Livingstone; 1980.
Fayad L.M., Johnson P., Fishman E.K. Multidetector CT of musculoskeletal disease in the pediatric patient: Principles, techniques, and clinical applications. Radiographics. 2005;25:603-618.
Feied C.F. Pulmonary embolism. In: Rosen P., Barkin R.M., editors. Emergency Medicine Principles and Practice, vol 3. 4th ed. St. Louis: Mosby Year-Book; 1998:3.
Heytens l., Verlooy J., Gheuens J., Bossaert L. Lazarus sign and extensor posturing in a brain-dead patient. J Neurosurg. 1989;71:449-451.
Hoffer P.B. Gallium 67 Imaging. New York: Wiley; 1978. p 3
Huang A.H. The hot nose sign. Radiology. 2005;235:216-217.
Hussain H.M., Barnes C.E. Pediatric skeletal trauma: Plain film to MRI. Appl Radiol 36. 8, 2007.
Hwang, Hwang I: Imaging osteomyelitis. www.wramc.amedd.army.mil/departments/nuclear
Kao S.C., Smith W.L. Skeletal injuries in the pediatric patient. Radiol Clin North Am. 1997;35:727-746.
Kowalsky R.J., Falen S.W. Radiopharmaceuticals in Nuclear Pharmacy and Nuclear Medicine. Washington, DC: APhA; 2004.
Lew D.P., Waldvogel F.A. Current concepts: Osteomyelitis. New Engl J Med. 1997;336:999-1007.
Love C., Palestro C.J. Radionuclide imaging of infection. J Nucl Med Technol. 2004;32:47-57.
Love C., Tomas M.B., Marwin S.E., et al. Role of nuclear medicine in diagnosis of the infected joint replacement. Radiographics. 2001;21:1229-1238.
Mandelstam S.A., Cook D., Fitzgerald M., et al. Complementary use of radiological skeletal survey and bone scintigraphy in detection of bony injury in suspected child abuse. Arch Dis Childhood. 2003;888:387-390.
McGlone B.S., Balan K.K. The use of nuclear medicine techniques in the emergency department. Emerg Med J. 2001;18:424-429.
Mettler F.A., Guiberteau M.J. Essentials of Nuclear Medicine Imaging, 5th ed. Philadelphia: WB Saunders; 2005.
Mishkin F.S., Dyken M.L. Increased early radionuclide activity in the nasopharyngeal area in patients with internal carotid artery obstruction: Hot nose. Radiology. 1970;96:77-80.
Oyen W.J., Boerman O.C., Corstens F.H. Imaging acute appendicitis: Invited commentary. J Nucl Med. 2000;41:456.
PIOPED Investigators. Technescan MAA kit (package insert). St. Louis, MO: Mallinckrodt; 2001.
PIOPED investigators. Value of the ventilation/perfusion scan in acute pulmonary embolism: Results of the prospective investigation of pulmonary embolism diagnosis. JAMA. 1990;263:2753-2759.
Runge M.S., Patterson C. Principles of Molecular Medicine, 2nd ed. Washington DC/Berlin: Humana Press; 2006.
Saber AA, LaRaja RD: Abdominal abscesses. Available online: http://emedicine.medscape.com/article/189468-diagnosis
Schweiger Schweiger B: Non-accidental injury in children—diagnostic imaging. Pediatric Trauma III. http://nordictraumarad.com/Syllabus04/pedtrauma3.pdf
Sharp et al., Sharp P.F., Gemmell H.G., Murray A.D. Practical Nuclear Medicine, 3rd ed. Washington DC/Berlin: Springer; 2005.
SNM Procedure Guideline for Hepatobiliary Scintigraphy, version 3.0, approved June 23, 2001.
Tofe A.J., Francis M.D. In vitro stabilization of low-tin bone imaging agent (99mTc-Sn-HEDP) by ascorbic acid. J Nucl Med. 1976;17:820-825.
Tucker W.D., Greene M.W., Weiss A.J., Murrenhoff A.P. Methods of preparation of some carrier-free radioisotopes involving sorption on alumina. USAEC Report BNL-3746, Brookhaven National Laboratory. May 29, 1958.
Zeissmann H.A. Clinical scintigraphy: cenitourinary system. In O’Malley J.P., Thrall J.H., editors: The Requisites: Nuclear Medicine, 3rd ed, Philadelphia: Mosby, 2005.