Chapter 91 Electrical Therapy for Tachyarrhythmias
Future Directions
In their 25 years of existence, implantable cardioverter-defibrillators (ICDs) have moved to the forefront as the best therapy for the prevention of sudden cardiac death (Figure 91-1). Since their inception, they have transitioned from a cumbersome, last-ditch therapy to the standard of care for patients at risk of ventricular tachyarrhythmias. Despite this record of tremendous innovation during this relatively short history, in the minds of some, ICDs have become “good enough” and are on their way to becoming a commodity, with only simplification and reduction of inappropriate shocks being the remaining challenges to differentiate existing products from future products. In contrast to that perspective, this chapter serves to describe the multiple opportunities and challenges that portray an exciting future for devices providing electrical therapy for tachyarrhythmias.
Therapies for Ventricular Tachyarrhythmia Termination
Defibrillation
The most essential therapy an ICD is intended to provide is termination of ventricular fibrillation (VF). The loss of coordinated cardiac contraction associated with fibrillation causes patients to lose blood pressure and consciousness immediately, which, if not quickly reversed, results in death. Electric countershock has long been known to interrupt fibrillation. The re-entrant wavefronts that are the hallmark of fibrillation can only be halted by nearly simultaneous depolarization of the ventricular myocardium. To achieve this, a sufficient amount of energy must be provided such that a voltage gradient of approximately 5 V/cm traverses most of the heart.1 Traditionally, such shocks have been delivered by discharging a capacitor between two electrodes on, in, or near the heart. Early ICDs used monophasic truncated exponential waveforms. Biphasic waveforms proved to have much better defibrillation efficacy and thereby significantly advanced the use of ICDs in the early 1990s.2–6
Capacitors
Today’s ICDs use capacitors in the range of 100 to 140 µF. Connected with transvenous electrode systems, their discharge time constant is in the order of 4 to 6 ms. Mathematical modeling has predicted that the optimal discharge time constant to minimize the energy required for defibrillation is around 2 to 4 ms.7 A shorter time constant could be achieved by simply reducing capacitance below 100 µF. In an ICD, the shock is actually discharged from a bank of multiple (typically three) capacitors. Each capacitor can hold a maximum of approximately 250 V (a property determined by their chemistry, which is most commonly aluminum electrolytic). Stacked in series, the total voltage adds up to approximately 750 V. Using the formula , the total stored energy for a 125 µF system is 35 J. If the discharge time constant were to be optimized by reducing the capacitance to 100 µF or less, the stored energy would be reduced to 28 J or less. While the waveform would be more optimal, the reduction in total stored energy would decrease more quickly with decreasing capacitance than would the energy required to defibrillate, thus reducing the energy safety margin. This dilemma can only be resolved by improvements in capacitor technology such that individual components can hold a higher peak voltage and thereby maintain the same maximum energy despite the reduction in capacitance. Research into new metal oxides or entirely different materials may allow the development of the required capacitor improvements. Recent development has focused on tantalum oxide rather than the currently used aluminum for capacitor construction. In addition to the potential for higher peak voltage, tantalum capacitors offer higher energy density and can be shaped more easily to conform to the desired form of the overall device. These factors are likely to yield devices that are smaller with shapes better suited for implantation.
Defibrillation Waveforms
The truncated exponential waveform used in ICDs has the advantage of being easy to generate from the small components required for an implantable device, but it is not ideally suited for depolarizing excitable membranes. Since cell membranes cannot react completely to a rapid step increase in voltage, slow-rise defibrillation waveforms are theoretically more efficient.8 Studies on humans have shown that ramp waveforms can defibrillate with approximately 20% lower delivered energy and 24% lower peak voltage (Figure 91-2).9 However, with currently available components, the technology required to produce ramp waveforms cannot be incorporated into small ICDs. In the future, new or more efficient technology may facilitate inclusion of new waveforms that may have better defibrillation efficacy, result in less tissue damage, or be associated with less discomfort.
Shock Delivery Systems
Shock delivery leads and electrodes are a vital component of the total ICD system. From experimental and modeling data, the ideal electrodes would deliver a uniform electric field of approximately 5 V/cm across the ventricles for an effective defibrillation shock. To accomplish this task, the earliest commercial ICDs used large surface area epicardial patches placed directly around the heart. Thoracotomy, with its attendant risks, was required for patch placement; therefore, transvenous electrode systems were developed and soon supplanted epicardial leads. Transvenous electrode systems with their less uniform electric fields would likely not have been as effective an alternative, were it not for the significantly improved defibrillation efficacy of biphasic shock waveforms; these systems became available around the same time. Today, almost all ICD implants include a combination of a coil electrode in the right ventricle (RV) and the metal housing of the ICD (the “Can” electrode). A majority of implants also include a coil electrode in the superior vena cava (SVC) incorporated into the same lead as the RV coil, a so-called dual-coil lead. When the SVC coil is electrically coupled to the Can electrode, a dual-coil lead has been shown to have lower defibrillation thresholds than a single-coil (RV only) system in most paired studies in humans, though the differences are relatively small.10–13 Accordingly, many physicians choose to use a single-coil system, which, at least in theory, might offer improved reliability because of the simpler design and improved extractability because of less fibrous growth in the region of the SVC coil. If patients do not meet the required implant criteria with standard transvenous systems, other alternatives include placement of another coil lead in the SVC, azygous vein, or left posterior subcutaneous region (Figure 91-3).14–16
In order to eliminate some of the disadvantages of transvenous lead systems, recent research has explored the efficacy of totally subcutaneous ICD systems. Defibrillation would be achieved by shocking transthoracically between the device and one or more subcutaneous coil electrodes with either an anteroposterior vector or a vector solely on the left anterior quadrant (Figure 91-4).17–19 The feasibility of defibrillation has been established for at least some patients, albeit with higher energy requirements than for traditional transvenous ICD devices; however, published literature on the sensitivity and specificity for the detection of ventricular arrhythmias using an entirely subcutaneous system is sparse. One study on recorded signals has reported sensitivity and specificity comparable with transvenous ICD systems.20 Before understanding the future potential of such a system, other trade-offs, such as increased device density, increased charge times, the difficulty to reliably pace for bradycardia following shock discharge, and inability to use painless anti-tachycardia pacing (ATP) instead of full defibrillation shock energy, must be resolved.
Importance of Shock Reduction
The importance of shock reduction has multiple benefits. Improved device longevity by not delivering unnecessary high power shocks is the more straightforward benefit. In the current ICDs, each shock reduces device longevity by approximately 1 month. Another obvious benefit is enhanced quality of life for patients who are spared discomfort. Research has shown that patients with ICDs suffer from psychological distress ranging from general anxiety to post-traumatic stress disorder, with shocks being a primary factor.21,22 Another potential benefit may be monetary savings, since shocks are a significant reason for hospitalization of patients with ICDs.23 Perhaps the most important factor is a recent analysis showing the significant impact of shocks on patient mortality. Analysis of data from the Multicenter Automatic Defibrillator Implantation Trial (MADIT-II) and the Sudden Cardiac Death in Heart Failure (SCD-HeFT) trial revealed a significant increase in mortality risk associated with both appropriate and inappropriate shocks (Table 91-1).24,25 From the available data, it is unclear whether this association is related to shock therapy itself or only to the episodes that precipitate their occurrence. Analysis of data pooled from multiple trials focused on the use of ATP for shock reduction showed that shocks, but not ATP therapy for appropriately detected VT/VF episodes, were associated with increased mortality; the analysis also concluded that shocks for inappropriately detected VT/VF did not have a significant impact on mortality (see Table 91-1).26 Given the established benefit of SCD prevention with ICDs in both the MADIT-II and SCD-HeFT trials, despite the trials’ reliance on shocks for VT/VF termination, it is possible that the ICD survival benefit was underestimated and, in fact, could have been greater if ATP had been used more aggressively in these trials. Further research into the possible mechanism may allow future ICDs to modify shock therapy to reduce any avoidable consequences associated with shocks.
Anti-tachycardia Pacing
While defibrillation is the most essential therapy for an ICD, ATP for ventricular arrhythmias is actually the most frequently delivered therapy. Even in so-called primary-prevention patients—those without a prior history of VT/VF—monomorphic VT represents more than 90% of the ventricular tachyarrhythmias detected by ICDs (Figure 91-5).27,28 It is interesting to note that the use of ATP and the first implantable anti-tachyarrhythmia devices were, in fact, pacemakers for the treatment of supraventricular tachycardia. It was known at the time that ATP could also be effective for the termination of VT, but the possibility of acceleration of a relatively well-controlled rate to a more hemodynamically significant arrhythmia prevented its widespread use for the treatment of VT until combined devices with high-voltage defibrillation capability were developed.
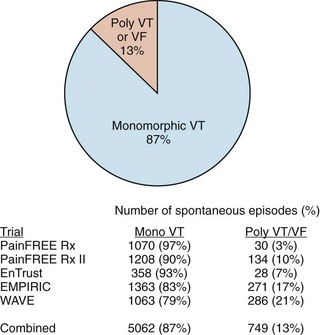
FIGURE 91-5 Distribution of monomorphic ventricular tachycardia (VT) versus polymorphic VT and ventricular fibrillation (VF) in five clinical trials sponsored by Medtronic Inc. (Minneapolis, MN) showing a predominance of monomorphic rhythms amenable to anti-tachyarrhythmia pacing.27–3048 Numbers shown are for spontaneous episodes adjudicated to be ventricular in origin. The detected rhythms are influenced by trial population, device type, and protocol for implantable cardioverter-defibrilator programming, but all studies demonstrated that polymorphic VT and VF represent only a minority of the episodes.
While ATP has long been used for the treatment of slow monomorphic tachycardia as a painless alternative to shock therapy, a series of trials in the past decade have demonstrated the success of empirically programmed ATP in treating fast ventricular tachycardia (FVT) as well. The Pacing Fast Ventricular Tachycardia Reduces Shock Therapies (PainFREE Rx), PainFREE Rx II, and EMPIRIC trials all delivered at least one sequence of burst ATP for fast ventricular tachycardia with a rate of 189 to 250 beats/min (320 to 240 ms).27–29 ATP was effective in terminating 49% to 78% of FVT episodes, providing a significant reduction in the need for shocks. One of the studies, PainFREE Rx II, had a control arm in which patients with similar FVT episodes received shocks. The results demonstrated a significant quality-of-life improvement for patients in the ATP arm compared with patients in the shock arm, which provided solid evidence for the benefit of shock reduction. The EMPIRIC trial had a control arm in which devices received physician-tailored programming as opposed to preset nominal programming. While both arms had similar rates of all-cause shocks, the trial demonstrated that the study’s nominal programming could be used empirically, thereby minimizing time spent during the implantation session. The relative paucity of VT acceleration or syncopal episodes was equally important in the trials. As further evidence becomes available, the use of ATP for FVT as a method of limiting shocks is expected to become more common. Some current ICDs, to eliminate any delay in therapy, incorporate a feature that allows ATP while also charging the capacitors.30 To preserve battery life, these devices can withhold charging once ATP termination has been established successfully.
Enhancements of Anti-tachycardia Pacing
One common belief with regard to the mechanism of ATP failure has been the lack of proximity of the pacing site to the re-entrant circuit responsible for VT. It has been postulated that when pacing from the RV apex, pacing wavefronts are unable to reach the left ventricular site of re-entry. However, recent work has suggested that the number of pulses required to reach the re-entrant circuit via decreasing or peeling back the refractory period may vary depending on the pacing site, but that, under most circumstances, the pacing train will reach the circuit regardless of where the pacing lead is placed. In a paired, randomized comparison of induced VT, ATP applied from both RV and left ventricular (LV) sites were equally effective at termination.31 In addition, in trials comparing RV ATP with biventricular (RV-LV) ATP for spontaneous VT, no significant difference based on pacing site was found.32,33
Given that physically reaching the re-entrant circuit is not dependent on the pacing site, a remaining explanation for ATP failure would be the inability of the pacing train to electrically interact with and terminate the re-entry. Clinical research is under way to exploit available information on the effect of heart rate and return cycle length following entrainment to automatically adjust the pace train, thus improving the results with better conduction into the excitable gap.34,35
Therapies for Prevention of Ventricular Arrhythmia
To date, implantable arrhythmia devices have focused on the successful detection and termination of life-threatening arrhythmias. An equally compelling opportunity would exist for the device to entirely prevent an arrhythmia. Several attempts have been made to demonstrate that specific pacing activity may effectively reduce episodes of VT. Some evidence exists that pacing at intervals shorter than the underlying sinus rhythm, the so-called ventricular overdrive pacing, may accomplish this task in secondary-prevention patients with VT. One clinical trial tested overdrive suppression in a randomized crossover design in patients presenting with frequent VTs, or ventricular tachycardia storm.36 Though the 17% reduction in the number of sustained VT episodes did not reach statistical significance (P = .06), results did establish a 78% reduction in the number of nonsustained VTs (P = .004). Although continuous overdrive pacing may have negative effects in some patients with exacerbated heart failure (HF) or ischemia, further research may determine a population that may benefit or may determine the conditions that predict impending episodes and the opportunity for appropriate intermittent application of prevention algorithms.
Another proposed method for VT/VF prevention is to suppress pauses associated with premature beats. It has been shown that approximately 10% to 20% of VTs may be associated with short-interval or long-interval onset following premature ventricular contractions. In one randomized crossover study of a pause suppression ICD feature, no significant decrease was found in VT/VF events.37 However, a post hoc analysis found a significant reduction in the subset of patients who exhibited at least one short-interval or long-interval onset of VT during the follow-up.38 Again, even if not universally applicable, the concept that an ICD may be able to detect conditions in some patients when a prevention algorithm would be beneficial should be explored for future ICDs.
Hybrid Therapies
The important influence of the nervous system on cardiac function and arrhythmias has long been recognized. However, the idea of using this heart-brain connection for the chronic management of arrhythmias has only recently been explored. Prospective research in animal models revealed that spinal cord stimulation, which was first applied for the control of pain associated with angina, could reduce the number of animals experiencing reperfusion VT/VF.39–41 More recently, chronic spinal cord stimulation has been shown to reduce cardiac dimensions and other HF metrics in animal models of chronic HF.42 The possibility that a spinal cord stimulator, which is still in its infancy, could be used in concert with an ICD to concurrently manage arrhythmias represents an exciting area of research.
Detection and Discrimination of Ventricular Arrhythmias
With current technology, ICDs achieve greater than 99% sensitivity in detecting ventricular tachyarrhythmias. Maintaining a high specificity for treating only fast rhythms of ventricular origin is far more difficult. Both supraventricular tachycardias (such as sinus tachycardia and atrial flutter/fibrillation) and oversensing (including T waves, myopotentials, and nonphysiological noise) overlap in rate with VT/VF. To be most successful, ICDs incorporate various discrimination algorithms to differentiate true VT from inappropriate sensing. The two primary methods involve either simultaneous analysis of atrial sensed events or a morphology analysis of the ventricular electrogram. The advent of dual-chamber ICDs in the 1990s added not only the ability to provide atrial-based pacing but, more importantly, also an atrial electrogram for incorporation into discrimination algorithms. The use of an atrial signal allows for a comparison of the atrial rate as well as the sequence and relative number of P waves and R waves to differentiate fast rhythms that are ventricular from those of supraventricular origin. Dual-chamber discrimination algorithms have yielded supraventricular tachycardia (SVT) detection specificity of about 55% to 90%, with rapid one-to-one conducted rhythms being the most challenging to discriminate.43–45 Though the earliest ICDs had crude morphology algorithms, more sophisticated morphology algorithms have been developed to differentiate narrow R waves that are presumably supraventricular in origin from wide R waves that imply VT.46 These ventricular electrogram morphology algorithms have demonstrated a reduction of inappropriate SVT detection of 55% to 90%.47–49 Until now, ICD detection and discrimination algorithms have relied solely on processing passively sensed physiological signals. During acute testing in the electrophysiology laboratory, both atrial and ventricular stimuli are often used to discriminate ventricular arrhythmias from supraventricular arrhythmias. Future ICDs may incorporate algorithms to use atrial pacing, ventricular pacing, or both to discriminate fast one-to-one rhythms not easily identified with existing single-chamber or dual-chamber algorithms.50 In one early investigation on spontaneous rhythms, the “discriminating” stimuli terminated approximately 80% of one-to-one VTs and subsequently correctly classified 649 (99.7%) of 651 ongoing one-to-one SVTs.51
Sustained Versus Nonsustained Episodes
The time that an ICD should wait to declare that an ongoing VT is sustained and therefore treatable has received increased attention as it has become clear that at nominal detection settings ICDs may over-treat ventricular arrhythmias. This suggestion has been raised by data from randomized controlled ICD survival trials, which indicate that more treated VT events occur in the ICD therapy groups than do deaths in the control groups. Therefore, a treated event—even if it is fast ventricular tachycardia—does not necessarily equate to a truly lifesaving therapy but, rather, might be an event that would have terminated spontaneously. The dilemma for physicians and device manufacturers is how long the ICD should stand by in spite of knowing that added duration of tachycardia may increase the likelihood of syncope or even defibrillation failure.52,53 A recent clinical trial (Primary Prevention Parameters Evaluation [PREPARE]) programmed ICD detection to a threshold of at least 30 fast intervals before satisfying criteria for sustained treatable VT/VF.29 The trial results established a marked increase in time to first shock and a reduction in the number of treated events, compared with historical controls using a shorter duration of episodes. This reduction in treated events was partly related to self-terminating episodes of VT and partly to the improved function of the discrimination algorithm related to the longer duration allowed. Importantly, neither syncope nor more serious consequences increased significantly.
Reviewed in the context of previously described ATP trials, PREPARE also sheds light on the true efficacy of ATP versus nonsustained episodes appearing as ATP success. The earlier trials all prescribed FVT detection requiring 12 of 16 intervals (PainFREE Rx) or 18 of 24 (PainFREE Rx II and EMPIRIC) to be 320 ms or less with the last eight intervals greater than 240 ms. In the shock arm of PainFREE Rx II, half the patients were randomized to receive shocks for FVT, and 34% of those episodes self-terminated before shocks, providing evidence that at least some of the ATP successes in the ATP arm were likely spontaneously terminating VT.28 In the PREPARE study, which required 30 of 40 intervals to be between 240 and 320 ms for detection, the ATP efficacy was 49%.54 With the earlier trials showing 72% to 78% ATP success, PREPARE confirmed the PainFREE Rx II shock arm results that approximately one third of detected FVT episodes will spontaneously terminate if left untreated for a brief additional period.
Monitoring and Diagnostics
Information management has become an important consideration in implantable medical devices. With significant component miniaturization and improvements in storage capacity of device memory in the past 2 decades, ICDs have transitioned from storing only primitive event counter information to more complex diagnostics. Such features include high-quality stored electrograms for each detected episode, device function diagnostics, and daily trend information for extended periods on multiple cardiac metrics (Figure 91-6). Stored electrograms may be used to interpret events that trigger device detection, to determine the efficacy of programmed therapies, or to diagnose lead malfunction. Automatic monitoring of device function has become a standard feature in ICD devices that feature programmable patient alerts with different levels of urgency. Elective replacement indicators have long been a relatively nonurgent indicator of impending battery depletion. Other more urgent warnings might include failed therapies, potential lead fractures, or inadvertent inappropriate programming of detection suspension.
Monitoring Comorbidities
Patients requiring ICD devices frequently have comorbidities such as hypertension, HF, diabetes, coronary artery disease, renal insufficiency, and sleep apnea. As devices become more sophisticated, other physiological information in addition to cardiac arrhythmias may be monitored. For example, the Chronicle Offers Management to Patients with Advanced Signs and Symptoms of Heart Failure (COMPASS-HF) trial studied the ability of an RV pressure monitoring device (Chronicle) to improve outcomes in patients with chronic HF. The primary endpoint to reduce all-cause HF-related events was not met, but the Chronicle group did have a nonsignificant 21% reduction compared with the control group.55 An analysis of the time to first HF hospitalization did show a 36% reduction in the relative risk of an HF-related hospitalization in the Chronicle group. It is possible that such a monitoring device may have had a statistically significant impact if compared with a control group not being monitored as closely as those under the rigor of this trial. As mentioned earlier, a unique investigational ICD records and stores information on RV pressure to assist in the management of HF as well as providing traditional pacing and high-voltage therapy. Another parameter to aid in HF management provided by some ICDs is a metric related to fluid volume in the lungs. For example, transthoracic impedance information that relates to lung wetness may be trended to detect changes in HF status even before significant alterations in symptoms or other classic signs appear. In an early 2-year trial of 33 patients, in which 10 were hospitalized for fluid overload, intrathoracic impedance reduction began approximately 2 weeks before the onset of worsening symptoms.56 Automated detection of impedance decreases was 77% sensitive in detecting hospitalization for fluid overload, with a low false-positive rate. This ability allows earlier detection of HF decompensation leading to earlier treatment and the prevention of adverse events such as hospitalization. Management of such information may be provided by health care professionals; or in the future, patients will increasingly be empowered to modify their lifestyle or alter therapy themselves.
In addition to monitoring HF status, future devices will aid in the management of comorbidities. Under current implantation guidelines, the majority of ICD recipients have ischemic cardiac disease. Cardiac repolarization changes associated with acute ischemia have been observed on intracardiac electrograms available to ICDs (Figure 91-7).57–59 Detection algorithms to alert physicians and patients to serious ischemic events are being designed and are under study.60–62 Such information might allow earlier acute coronary intervention, or in the future, devices may be incorporated with drug delivery systems to provide closed-loop pharmacologic therapy.
Hypertension is a common disease in general and no less common in the population of patients who receive implantable electrical devices. As we learn more about the disease, it is clear that intermittent blood pressure measurements in the clinical setting may not always be adequate for diagnosis or long-term management, leading to at least some interest in external or implantable ambulatory monitoring.63,64 Small sensors that can easily be implanted are in development. Using device memory and telemetry, accurate recordings can be acquired and stored over time. In addition, there are 5% to 30% of patients with significant hypertension who are refractory to current pharmacologic therapy.65,66 It is certainly not hard to imagine that implantable devices might help treat hypertension using electrical stimulation for autonomic modulation or even closed-loop systems for drug delivery.
Connectivity and Communications
Another new aspect of device communication will likely be intrabody communication (Figure 91-8). With the advance of technology, it is possible, if not likely, that patients may benefit from several devices to treat other chronic diseases. Especially as the information collected by monitoring devices becomes more diverse and devices become smaller, a significant challenge will be the exchange of information among devices, that is, establishing a central point for communication with external systems as well as coordination between systems.67 In addition to using sensors to monitor chronic disease, it is likely that such technology will also be applied to a system for personal health care.68
Key References
Bardy GH, Smith W, Hood M, et al. An entirely subcutaneous implantable cardioverter-defibrillator. N Engl J Med. 2010;363:36-44.
Bourge RC, Abraham WT, Adamson PB, et alCOMPASS-HF Study Group. Randomized controlled trial of an implantable continuous hemodynamic monitor in patients with advanced heart failure: The COMPASS-HF study. J Am Coll Cardiol. 2008;51:1073-1079.
Frantz R, Benza R, Kjellström B, et al. Continuous hemodynamic monitoring in patients with pulmonary arterial hypertension. J Heart Lung Transplant. 2008;27:780-788.
Issa ZF, Zhou X, Ujhelyi MR, et al. Thoracic spinal cord stimulation reduces the risk of ischemic ventricular arrhythmias in a postinfarction heart failure canine model. Circulation. 2005;111:3217-3220.
Lopshire JC, Zhou X, Dusa C, et al. Spinal cord stimulation improves ventricular function and reduces ventricular arrhythmias in a canine post-infarction heart failure model. Circulation. 2009;120:286-294.
Ridley DP, Gula LJ, Krahn AD, et al. Atrial response to ventricular antitachycardia pacing discriminates mechanism of 1:1 atrioventricular tachycardia. J Cardiovasc Electrophysiol. 2005;16:601-605.
Schoels W, Steinhaus D, Johnson WB, et al. EnTrust Clinical Study Investigators: Optimizing implantable cardioverter-defibrillator treatment of rapid ventricular tachycardia: Antitachycardia pacing therapy during charging. Heart Rhythm. 2007;4:879-885.
Shorofsky SR, Rashba E, Havel W, et al. Improved defibrillation efficacy with an ascending ramp waveform. Heart Rhythm. 2005;2(4):388-394.
Theres H, Stadler RW, Stylos L, et al. Comparison of electrocardiogram and intrathoracic electrogram signals for detection of ischemic ST segment changes during normal sinus and ventricular paced rhythms. J Cardiovasc Electrophysiol. 2002;13:990-995.
Wathen MS, Sweeney MO, DeGroot P, et al. Shock reduction using antitachycardia pacing for spontaneous rapid ventricular tachycardia in patients with coronary artery disease. Circulation. 2001;104:796-801.
Wathen MS, DeGroot P, Sweeney MO, et al. PainFREE Rx II Investigators: Prospective randomized multicenter trial of empirical antitachycardia pacing versus shocks for spontaneous rapid ventricular tachycardia in patients with implantable cardioverter defibrillators: PainFREE Rx II trial results. Circulation. 2004;110:2591-2596.
Wesselink W, Hampton D, Splett V, Musley S. Subcutaneous detection of acute myocardial infarction—preliminary results. Proceedings of the 5th International Summer School and Symposium on Medical Devices and Biosensors. 2008, 198-200.
Wilkoff BL, Williamson BD, Stern RS, et alPREPARE Study Investigators. Strategic programming of detection and therapy parameters in implantable cardioverter-defibrillators reduces shocks in primary prevention patients: Results from the PREPARE (Primary Prevention Parameters Evaluation) study. J Am Coll Cardiol. 2008;52:541-550.
Yee R, Birgersdotter-Green U, Belk P, et al. The relationship between pacing site and termination of sustained monomorphic ventricular tachycardia by antitachycardia pacing. Pacing Clin Electrophysiol. 2010;33(1):27-32.
1 Witkowski FX, Penkoske PA, Plonsey R. Mechanism of cardiac defibrillation in open-chest dogs with unipolar DC-coupled simultaneous activation and shock potential recordings. Circulation. 1990;82:244-260.
2 Winkle RA, Mead RH, Ruder MA, et al. Improved low energy defibrillation efficacy in man with the use of a biphasic truncated exponential waveform. Am Hrt J. 1989;17:122-127.
3 Saksena S, An H, Mehra R, et al. Prospective comparison of biphasic and monophasic shocks for implantable cardioverter-defibrillators using endocardial leads. Am J Cardiol. 1992;70:304-310.
4 Block M, Hammel D, Bocker D, et al. A prospective randomized cross-over comparison of mono- and biphasic defibrillation using nonthoracotomy lead configurations in humans. J Cardiovasc Electrophysiol. 1994;5:581-590.
5 Natale A, Dhala A, Axtell K, et al. Permanent implant of a biphasic device using a transvenous system: Comparison with a monophasic device using the same lead system. Pacing Clin Electrophysiol. 1994;17:757.
6 Wyse D, Kavanagh K, Gillis A, et al. Comparison of biphasic and monophasic shocks for defibrillation using a nonthoracotomy system. Am J Cardiol. 1993;71:197-202.
7 Swerdlow CD, Brewer JE, Kass RM, Kroll MW. Application of models of defibrillation to human defibrillation data: Implications for optimizing implantable defibrillator capacitance. Circulation. 1997;96:2813-2822.
8 Fishler MG. Theoretical predictions of the optimal monophasic and biphasic defibrillation waveshapes. IEEE Trans Biomed Eng. 2000;47:59-67.
9 Shorofsky SR, Rashba E, Havel W, et al. Improved defibrillation efficacy with an ascending ramp waveform in humans. Heart Rhythm. 2005;2:388-394.
10 Gold MR, Foster AH, Shorofsky SR. Lead system optimization for transvenous defibrillation. Am J Cardiol. 1997;80:1163-1167.
11 Gold MR, Olsovsky MR, Pelini MA, et al. Comparison of single- and dual-coil active pectoral defibrillation lead systems. J Am Coll Cardiol. 1998;31:1391-1394.
12 Gold MR, Olsovsky MR, DeGroot P, Cuello C, Shorofsky SR. Optimization of transvenous coil position for active can defibrillation thresholds. J Cardiovasc Electrophysiol. 2000;11:25-29.
13 Gold M, Val-Mejias J, Leman R, et al. Optimization of superior vena cava coil position and usage for transvenous defibrillation. Heart Rhythm. 2008;5:394-399.
14 Cesario D, Bhargava M, Valderrábano M, et al. Azygos vein lead implantation: A novel adjunctive technique for implantable cardioverter defibrillator placement. J Cardiovasc Electrophysiol. 2004;15:780-783.
15 Gradaus R, Block M, Seidl K, et al. Defibrillation efficacy comparing a subcutaneous array electrode versus an “active Can” implantable cardioverter defibrillator and a subcutaneous array electrode in addition to an “active Can” implantable cardioverter defibrillator: Results from active Can versus array trials I and II. J Cardiovasc Electrophysiol. 2001;12:921-927.
16 Sweeney MO. Subcutaneous defibrillation leads: Long term stability of DFT, effect of late position changes, and implications for totally subcutaneous defibrillators. Heart Rhythm. 2007;4:S219.
17 Lieberman RA, Havel WJ, Rashba E, et al. Acute defibrillation performance of a novel, non-transvenous shock pathway in adult ICD indicated patients. Heart Rhythm. 2008;5:28-34.
18 Burke MC, Coman JA, Cates AW, et al. Defibrillation energy requirements using a left anterior chest cutaneous to subcutaneous shocking vector: Implications for a total subcutaneous implantable defibrillator. Heart Rhythm. 2005;2:1332-1338.
19 Bardy GH, Smith WM, Hood MA, et al. An entirely subcutaneous implantable cardioverter-defibrillator. N Engl J Med. 2010;363:36-44.
20 Gold MR, Theuns DA, Knight BP, et al. Arrhythmia detection by a totally subcutaneous S-ICD system compared to transvenous single-chamber ICD systems with morphology discrimination. Heart Rhythm. 2009;6:S34.
21 Sears SF, Lewis TA, Kuhl EA, Conti JB. Predictors of quality of life in patients with implantable cardioverter defibrillators. Psychosomatics. 2005;46:451-457.
22 Matchett M, Sears SF, Hazelton G, et al. The implantable cardioverter defibrillator: Its history, current psychological impact and future. Expert Rev Med Dev. 2009;6:43-50.
23 Korte T, Jung W, Ostermann G, et al. Hospital readmission after transvenous cardioverter/defibrillator implantation: A single centre study. Eur Heart J. 2000;21:1186-1191.
24 Daubert JP, Zareba W, Cannom DS, et al. MADIT II Investigators: Inappropriate implantable cardioverter-defibrillator shocks in MADIT II: Frequency, mechanisms, predictors, and survival impact. J Am Coll Cardiol. 2008;51:1357-1365.
25 Poole JE, Johnson GW, Hellkamp AS, et al. Prognostic importance of defibrillator shocks in patients with heart failure. N Engl J Med. 2008;359:1009-1017.
26 Sweeney MO, Sherfesee L, DeGroot PJ, et al. Differences in effects of electrical therapy type for ventricular arrhythmias on mortality in implantable cardioverter-defibrillator patients. Heart Rhythm. 2010;7:353-360.
27 Wathen MS, Sweeney MO, DeGroot P, et al. Shock reduction using antitachycardia pacing for spontaneous rapid ventricular tachycardia in patients with coronary artery disease. Circulation. 2001;104:796-801.
28 Wathen MS, DeGroot P, Sweeney MO, et al. PainFREE Rx II Investigators: Prospective randomized multicenter trial of empirical antitachycardia pacing versus shocks for spontaneous rapid ventricular tachycardia in patients with implantable cardioverter defibrillators: PainFREE Rx II trial results. Circulation. 2004;110:2591-2596.
29 Wilkoff BL, Williamson BD, Stern RS, et al. PREPARE Study Investigators: Strategic programming of detection and therapy parameters in implantable cardioverter-defibrillators reduces shocks in primary prevention patients: Results from the PREPARE (Primary Prevention Parameters Evaluation) study. J Am Coll Cardiol. 2008;52:541-550.
30 Schoels W, Steinhaus D, Johnson WB, et al. EnTrust Clinical Study Investigators: Optimizing implantable cardioverter-defibrillator treatment of rapid ventricular tachycardia: Antitachycardia pacing therapy during charging. Heart Rhythm. 2007;4:879-885.
31 Yee R, Birgersdotter-Green U, Belk P, et al. The relationship between pacing site and termination of sustained monomorphic ventricular tachycardia by antitachycardia pacing. Pacing Clin Electrophysiol. 2010;33(1):27-32.
32 Bocchiardo M, Achtelik M, Gaita F, et al. Efficacy of biventricular sensing and treatment of ventricular arrhythmias. Pacing Clin Electrophysiol. 2000;23:1989-1991.
33 Gasparini M, Anselme F, Clementy J, et al. Biventricular versus right ventricular antitachycardia pacing to terminate ventricular tachyarrhythmias in patients receiving cardiac resynchronization therapy: The ADVANCE CRT-D trial. Am Heart J. 2010;159:1116-1123.
34 Kouakam C, Lauwerier B, Klug D, et al. Effect of elevated heart rate preceding the onset of ventricular tachycardia on antitachycardia pacing effectiveness in patients with implantable cardioverter defibrillators. Am J Cardiol. 2003;92:26-32.
35 Yee R, Wathen M, Birgersdotter-Green U, et al. Determination of antitachycardia pacing failure mechanism by return cycle length analysis [abstract]. Eur Heart J. 2006;27:462.
36 Yee R, Muratore C, Rabinovich R, et al. Pacing algorithm for ventricular arrhythmic prevention in patients with implantable cardioverter-defibrillator [abstract]. Pacing Clin Electrophysiol. 2003;26:1038.
37 Grönefeld GC, Israel CW, Padmanabhan V, et al. Ventricular rate stabilization for the prevention of pause dependent ventricular tachyarrhythmias: Results from a prospective study in 309 ICD recipients. Pacing Clin Electrophysiol. 2002;25:1708-1714.
38 Canby RC, Zhou X, Pulling CC, et al. Reduction of tachyarrhythmias by ventricular rate stabilization in patients with ICD [abstract]. Pacing Clin Electrophysiol. 2001;24:585.
39 de Jongste MJ, Hautvast RW, Hillege HL, Lie KI. Efficacy of spinal cord stimulation as adjuvant therapy for intractable angina pectoris: A prospective, randomized clinical study. J Am Coll Cardiol. 1994;23:1592-1597.
40 Mannheimer C, Eliasson T, Augustinsson LE, et al. Electrical stimulation versus coronary artery bypass surgery in severe angina pectoris: The EBSY study. Circulation. 1998;97:1157-1163.
41 Issa ZF, Zhou X, Ujhelyi MR, et al. Thoracic spinal cord stimulation reduces the risk of ischemic ventricular arrhythmias in a postinfarction heart failure canine model. Circulation. 2005;111:3217-3220.
42 Lopshire JC, Zhou X, Dusa C, et al. Spinal cord stimulation improves ventricular function and reduces ventricular arrhythmias in a canine post-infarction heart failure model. Circulation. 2009;120:286-294.
43 Wilkoff BL, Kühlkamp V, Volosin K, et al. Critical analysis of dual-chamber implantable cardioverter-defibrillator arrhythmia detection: Results and technical considerations. Circulation. 2001;103:381-386.
44 Theuns DAMJ, Klootwijk APJ, Goedhart DM, Jordaens LJ. Prevention of inappropriate therapy in implantable cardioverter-defibrillators. Results of a prospective, randomized study of tachyarrhythmia detection algorithms. J Am Coll Cardiol. 2004;44:2362-2367.
45 Kouakam C, Kacet S, Hazard JR, et al. Performance of a dual-chamber implantable defibrillator algorithm for discrimination of ventricular from supraventricular tachycardia. Europace. 2004;6:32-42.
46 Toivonen T, Viitasalo M, Jarvinen A. The performance of the probability density function in differentiating supraventricular from ventricular rhythms. Pacing Cardiovasc Electrophysiol. 1992;15:726-730.
47 Friedman PA, McClelland RL, Bamlet WR, et al. Dual-chamber versus single-chamber detection enhancements for implantable defibrillator rhythm diagnosis: The DETECT Supraventricular Tachycardia Study. Circulation. 2006;113:2871-2879.
48 Klein GJ, Gillberg JM, Tang A, et al. Improving SVT discrimination in single-chamber ICDs: A new electrogram morphology-based algorithm. J Cardiovasc Electrophysiol. 2006;17:1310-1319.
49 Theuns DAMJ, Rivero-Ayerza M, Goedhart DM, et al. Morphology discrimination in implantable cardioverter-defibrillators: Consistency of template match percentage during atrial tachyarrhythmias at different heart rates. Europace. 2008;10:1060-1066.
50 Ridley DP, Gula LJ, Krahn AD, et al. Atrial response to ventricular antitachycardia pacing discriminates mechanism of 1:1 atrioventricular tachycardia. J Cardiovasc Electrophysiol. 2005;16:601-605.
51 Saba S, Volosin K, Yee R, Brown M. Combined atrial and ventricular antitachycardia pacing as a novel method of rhythm discrimination. Heart Rhythm. 2009;6:S72.
52 Windecker S, Ideker RE, Plumb VJ, et al. The influence of ventricular fibrillation duration on defibrillation efficacy using biphasic waveforms in humans. J Am Coll Cardiol. 1999;33:33-38.
53 Gradaus R, Bode-Schnurbus L, Weber M, et al. Effect of ventricular fibrillation duration on the defibrillation threshold in humans. Pacing Clin Electrophysiol. 2002;25:14-19.
54 Lee S, Stern R, Wathen M, et al. Anti-tachycardia pacing therapy effectively terminates fast ventricular tachycardia after longer detection duration in primary prevention patients: Results from the PREPARE trial [abstract]. Heart Rhythm. 2008;5:S344.
55 Bourge RC, Abraham WT, Adamson PB, et al. COMPASS-HF Study Group: Randomized controlled trial of an implantable continuous hemodynamic monitor in patients with advanced heart failure: The COMPASS-HF study. J Am Coll Cardiol. 2008;51:1073-1079.
56 Yu CM, Wang L, Chau E, et al. Intrathoracic impedance monitoring in patients with heart failure: Correlation with fluid status and feasibility of early warning preceding hospitalization. Circulation. 2005;112:841-848.
57 Varriale P, Niznik J. Unipolar ventricular electrogram in the diagnosis of right ventricular ischemic injury. Pacing Clin Electrophysiol. 1978;1:335-341.
58 Theres H, Stadler RW, Stylos L, et al. Comparison of electrocardiogram and intrathoracic electrogram signals for detection of ischemic ST segment changes during normal sinus and ventricular paced rhythms. J Cardiovasc Electrophysiol. 2002;13:990-995.
59 Musley S, Splett V, Warman E. Comparison of ST-segment deviation between surface lead V2 and endocardial signals during proximal and distal coronary artery occlusions in swine. Heart Rhythm. 2007;4:S402.
60 Baron TW, Faber TS, Grom A, et al. Real-time assessment of acute myocardial ischaemia by an intra-thoracic 6-lead ECG: Evaluation of a new diagnostic option in the implantable defibrillator. Europace. 2006;8:994-1001.
61 Williams JL, Mendenhall GS, Saba S. Effect of ischemia on implantable defibrillator intracardiac shock electrograms. J Cardiovasc Electrophysiol. 2008;19:275-281.
62 Wesselink W, Hampton D, Splett V, Musley S. Subcutaneous detection of acute myocardial infarction—preliminary results. Proceedings of the 5th International Summer School and Symposium on Medical Devices and Biosensors. 2008, 198-200.
63 Pickering TG, Shimbo D, Haas D. Ambulatory blood-pressure monitoring. N Engl J Med. 2006;344:2368-2374.
64 Frantz R, Benza R, Kjellström B, et al. Continuous hemodynamic monitoring in patients with pulmonary arterial hypertension. J Heart Lung Transplant. 2008;27:780-788.
65 Calhoun DC, Jones D, Textor S, et al. Resistant hypertension: Diagnosis, evaluation, and treatment. Hypertension. 2008;51:1403-1419.
66 van Halteren A, Bults R, Wac K, et al. Wireless body area networks for healthcare: The MobiHealth Project. Stud Health Technol Inform. 2004;108:181-193.
67 Alshehab A, Kobayashi N, Ruiz J, et al. A study on intrabody communication for personal healthcare monitoring system. Telemed J E Health. 2008;14:851-857.
68 Kaplan NM. Resistant hypertension. J Hypertension. 2005;23:1441-1444.