2 Early developmental events
Development of the nervous system
The location of the cells destined to develop into the neuraxis (nervous system) is probably already defined in the late gastrula stage of the embryo, at about 18 postovulatory days. At this point the embryo is about 1.5 mm in length (Huttenlocher 2002).
The development proper of the nervous system can first be identified at approximately 3 weeks (21 days) after conception, with the appearance of the neural plate. Originally the neural plate is roughly the shape of a ping-pong paddle, but soon develops a distinct neural groove, flanked by neural folds (Fig. 2.1). This fold is eventually drawn together by contractile tissue deep to the neural groove which pulls the folds together. Initially, while the tube is closing, its walls consist of a single layer of neuroepithelial cells. Each of these cells contacts both the internal (luminal) and external (basal) limiting membranes. This fold fuses by the end of the third week to the early fourth week, generally in what will eventually become the cervical region of the spinal cord and then extends zipper-like, rostrally forming the brain proper and caudally forming the thoracic, lumbar, sacral, and coccygeal regions of the spinal cord (Fig. 2.2).
The neural tube is originally open to the amniotic cavity by virtue of the neuropores located both rostrally and caudally, but by the end of the fourth week the neural tube closes at both ends with the closure of the neuropores. At the rostral end of the neural tube the primitive forebrain divides into two cerebral hemispheres and forms the two lateral ventricles and the third ventricle. The embryo is about 7 mm long at this point (day 32) in development (Behrman & Vaughan 1987). Once the neural tube has closed, the ectoderm forming the lips of the neural fold separates and forms the neural crest tissue (Fig. 2.3).
The neural crest cells give rise to several components of the peripheral nervous system, as well as a number of non-neural tissues. Tissues eventually formed by the neural crest cells include pigment cells of the skin; medullary cells of the adrenal gland; calcitonin-secreting cells of the thyroid gland; neurons of the paravertebral ganglia; many of the neurons in the ganglia of the cranial nerves V, VII, VIII, IX, and X; and neurons of the dorsal root ganglia (Leikola 1976) (Fig. 2.4). The cells of the neural crest disperse by migrating along well-defined pathways to their destinations. The phenotype of each cell seems to be largely determined by the position that they eventually occupy (Le Douarin 1982). For example, cells destined to migrate to the adenyl medulla (norepinephrine-secreting cells) have been experimentally transplanted to sites that give rise to cholinergic (acetylcholine-secreting) cells. The transplanted cells converted to cholinergic-secreting neurons (Cowan 1992).
The continued growth and expansion of the neural tube leads to the stimulation of overlying ectodermal tissues at various sites called ectodermal placodes. These ectodermal placodes lead to the development and formation of the sensory epithelia and cranial nerve ganglia. The various placodal development sites include the olfactory placode, the trigeminal placode, the auditory/vestibular placode, the facial placode, the vagal placode, and the glossopharyngeal placode.
These early stages of development are largely dependent on the processes of primary embryonic induction, which is largely under genetic control (Williams & Warwick 1980).
Gross morphological development
Shortly after the closure of the neural tube, a series of three vesicle-like swellings appear at the rostral apex of the tube. These swellings will eventually form the three primary brain vesicles: the prosencephalic (forebrain), the mesencephalic (midbrain), and the rhombencephalic (hindbrain) areas. Through various folding patterns the three primary vesicles ultimately give rise to the mature brain structures. The development of the three primary vesicles into their representative mature structures results in the following progression: the prosencephalic region develops into the telencephalon and the diencephalon; the mesencephalon remains undifferentiated, giving rise to the mature mesencephalon; and the rhombencephalon develops into the myelencephalon and metencephalon. Each of these secondary brain vesicles further develops into the recognised mature divisions of the human brain (Figs 2.5 and 2.6).
Primary developmental processes
The development of the mature nervous system is brought about by a series of steps that include processes both progressive and regressive (Cowan 1978). These steps include cell proliferation, neuronal migration, selective cell aggregation, cytodifferentiation, axonal outgrowth, and synapse formation. The first regressive events occur about the time that neurons in each population begin to form connections within their prospective projection fields. This phase is marked by the selective death of a substantial proportion (50%) of the initial population of prospective neuron cells. Many connections that were initially formed are eliminated and certain axon terminals are withdrawn. A large number of axon collaterals are also removed at this stage (Cowan & Hunt 1985). Each phase is considered in detail below.
Cell proliferative phase
The wall of the neural tube is formed initially by pseudostratified columnar epithelium that rests on the external basement membrane. Each cell sends a peripheral process to both the external (basal) lamina and the neural tube internal (luminal) surface. At the luminal surface a complex of junctional connections allows communication with other cells. The nuclei are found at different levels within the neuroepithelium, except those in the later stages of mitosis which are constantly found at the luminal aspect (Cowan 1981). The young neurons constantly migrate from the internal or luminal lamina to the external or basal lamina in a process called interkinetic nuclear migration. Throughout this process the neurons must continually retract and reform their peripheral processes. At this stage of development the neural tube consists of two histological areas of tissues, the ependymal or germinal cell layer found on the luminal aspect of the tube and the ventricular zone or matrix layer which spans the remainder of the tube and is in contact with the basal membrane. Continued cell division leads to growth and expansion of the neural tube in three ways: general expansion of the neural tube surface epithelium, rapid growth of the brain and spinal cord, and differentiation of the different lineages of cell types including glial cells and neurons. This differentiation phase is marked by a migration of some of the cells from the ventricular zone to the newly emerging intermediate or mantle zone, away from the luminal surface. The neurons in the intermediate zone send processes outward towards the basal membrane which eventually develops into the marginal zone. To summarise, at this point (4 weeks or 28 days) the development of the neural tube consists of four histologically identifiable layers: the ependymal or germinal layer, from which all the cells of the central nervous system will develop; the ventricular or matrix layer, which consists of newly formed cells undergoing interkinetic nuclear migration and mitosis; the intermediate or mantle zone, which consists of cells migrating from the intermediate zone; and the marginal zone, which consists of the elongated cytoplasmic processes of the cells in the intermediate zone. Interposed throughout these layers are developing glial cells including the radial glial cells which seem to act as guide cells for the migrating neurons (Fig. 2.7).
Each population of neurons is generated during a distinct period of embryonic or fetal life. This period is usually quite short, ranging from a few days to a week. Cells born of the same cohort can be classified as embryological homologues. The relationships that each cohort of neurons forms with other neurons of the same cohort become very important as the system matures. These relationships can be used clinically to gain insight into the function of other related homologous systems by stimulating one system of neurons and measuring the response of a homologous system through a variety of clinical testing procedures (see Embryological Homologous Relationships later in this chapter). The sequence at which the cells withdraw from the proliferative pool is well defined. For example, in the retina the ganglion cells farthest from the original luminal zone are generated first, followed by the inner nuclear layer, which are in turned followed by the photoreceptors. The actual sequence may vary somewhat from region to region; for example, the situation in the motor cortex is completely reversed with the deepest cells forming first (Fig. 2.8).
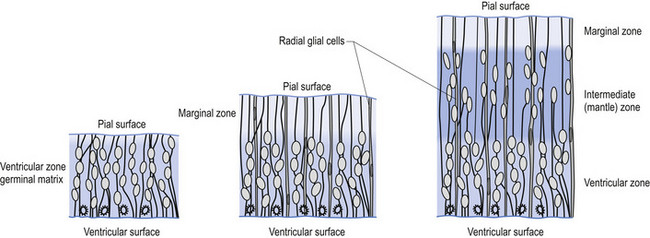
Figure 2.8 A magnified diagrammatic view of Fig. 2.7. The various cell proliferation zones, the intermediate zone, and the migration of the neurons via the radial glial cells are illustrated.
The process of neurogenesis seems to be highly programmed in both space and time. The process seems to progress largely in a ventral to dorsal, and cervical to sacral gradient (above, down, inside, out). In keeping with this strategy, the motor systems arising from the basal plate located ventrally are generally generated before the sensory systems arising from the alar plate, which is located dorsally (Figs 2.9A and 2.9B).