14 The cerebellum and vestibular system
Introduction
It is now clear that the cerebellum and vestibular systems also play a role in the integration of sensory information that is essential for generating appropriate responses to environmental stimuli and for a variety of other functions including constructing a perception of ourselves in the universe; controlling muscle movement; maintaining balance; maintaining internal organ and blood flow functionality; maintaining cortical arousal; and developing active plasticity in neural networks which allows environmental conditioning to occur. The importance of the cerebellum in the overall function of the neuraxis is demonstrated by the fact that there are approximately 20 million corticopontocerebellar fibres projecting between the cerebellum and the cortex, compared to only about 1 million corticospinal fibres supplying the cortical output to the voluntary muscles of the body. The integration function of the cerebellum is evident, as the input-to-output or afferent-to-efferent ratio in the cerebellum is approximately 40:1.
Anatomy of the cerebellum
The cerebellum lies behind the pons and medulla in the posterior cranial fossa (Fig. 14.1). It is separated from the cerebrum by an extension of dura mater, the tentorium cerebelli, and from the pons and medulla by the fourth ventricle (Fig. 14.2). It is somewhat smaller than the cerebrum but this difference varies with age, being 1/8 the size of the adult cortex but only 1/20 the size of the infant cortex.
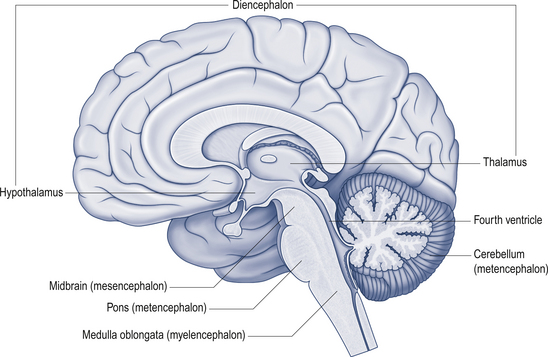
Figure 14.2 The relationship of the cerebellum to the cortex.
(from Drake et al. 2010. Gray’s Anatomy for Students 2nd edn. Churchill Livingstone, with permission)
The cerebellum is derived from the rhombencephalon, along with its homologues the pons and medulla, and is connected to the brainstem via three peduncles. These peduncles, together with the anterior and posterior medullary velum, are the main routes of entry or exit from the cerebellum. The inferior cerebellar peduncle, also known as the restiform body, conveys a number of axon projections into the cerebellum including (Figs 14.3 and 14.4):
1. The posterior spinocerebellar tract, which contains mossy fibres from the spinal cord that project to the cortex of the spinocerebellum;
2. The accessory cuneocerebellar tract, arising from the dorsal external arcuate fibres from the accessory cuneate nucleus;
3. The olivocerebellar tract, which contains climbing fibres of the contralateral inferior olivary nucleus;
4. The reticular cerebellar tract, which is formed from the ventral external arcuate fibres carrying projections from the arcuate and lateral reticular nucleus of the medulla; and
5. The vestibulocerebellar tract, which is formed from the projection fibres of the vestibular nuclei.
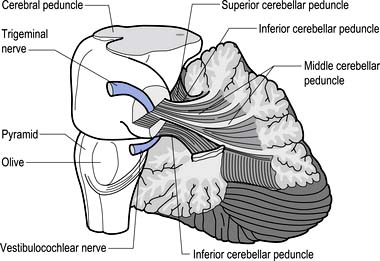
Figure 14.3 The anatomical relationships of the inferior, middle, and superior cerebellar peduncles.
The middle cerebellar peduncle or brachium pontis is the largest of the cerebellar peduncles, and contains the massive afferent corticopontocerebellar pathways. The middle peduncle is composed of axon projection fibres from the pontine nuclei to the contralateral cerebellum (Figs 14.3 and 14.4). The primary projections from the cerebral cortex to the cerebellum include premotor and supplementary motor areas (area 6), primary motor areas (area 4), primary sensory areas (areas 3, 1, and 2), and association and limbic cortices.
1. The dentatorubral tract, which projects from the dentate nucleus of the cerebellum to the opposite red nucleus in the mesencephalon;
2. The dentatothalamic tract, which projects to the contralateral thalamus;
3. The uncinate fasciculus, which contains fibres from the fastigial nucleus en route to the lateral vestibular nuclei in the medulla (Figs 14.3 and 14.4); and
4. Aminergic afferent projections, including noradrenergic, dopaminergic, and serotonergic afferents projecting to all areas of the cerebellum.
Noradrenergic neurons in the locus ceruleus project to Purkinje dendrites in the molecular layer and with granule cells in the granular layer (Bloom et al. 1971; Kimoto et al. 1981). Dopaminergic fibres arising from the neurons in the ventral mesencephalic tegmentum project to the Purkinje and granule neurons of interposed and lateral cerebellar nuclei (Simon et al. 1979). Serotonergic afferent fibres arise from the raphe nuclei of the brainstem and terminate in both the molecular and granule layers (Takeuchi et al. 1982).
The cerebellar surface is a striking example of natural economics in that it contains parallel convolutions or folia running in a transverse direction on the surface of the cerebellum that increase the surface area of the cerebellar cortex and give the cerebellum a tree-like appearance (Fig. 14.6). There are three primary lobes, anterior, posterior, and flocculonodular, on the cerebellar surface which are further dived into ten lobules. The cerebellum can be divided into nine regions along the vermis, which is a small unpaired structure in the median portion of the cerebellum separating the two large lateral masses. These nine regions are listed in Table 14.1 from anterior to posterior (see also Fig. 14.7). There are two major fissures that divide the cerebellum into three main lobes, and a number of other fissures that divide each lobe into its respective lobules.
Embryological development of the cerebellum
At the same time, the primary fissure begins to cut into the surface of the cerebellum, separating the anterior from the posterior lobe and other smaller fissures develop on the inferior surface.
The archicerebellum is the first region to appear in phylogeny and comprises the flocculi, their peduncles, the nodulus, and the lingula. The archicerebellum is the oldest and most medial portion of the cerebellum. In humans, archicerebellum contributes to the vestibulocerebellum, which comprises the flocculonodular lobe and the lingula (see Fig. 14.7) (Brodal 1981). As its new name would indicate, the vestibulocerebellum is the region of the cerebellum that communicates most intimately with the vestibular system. In fact, the vestibular nuclei of the brainstem share similar relationships with the cortex of the archicerebellum as the deep cerebellar nuclei share with the cortex of the palaeo- and neocerebellum. They therefore serve functionally as a cerebellar nuclear complex. The vestibulocerebellum also contains the only cerebellar cortical cells that leave the body of the cerebellum before synapsing. In the other regions of the cerebellum, the output cells of the cerebellar cortex synapse on neurons of the deep cerebellar nuclei. The stimuli from these neurons evoke inhibitory postsynaptic potentials (IPSPs) in the deep cerebellar nuclei.
Phylogenically, the palaeocerebellum is next to develop. Apart from the lingula, it comprises the anterior lobe, the pyramid, and uvula of the posterior vermis. This separated the archicerebellum into two parts, the lingula anteriorly and the flocculonodulus posteriorly (Brodal 1981). The palaeocerebellum contributes to the spinocerebellum, which is involved in a variety of parameters associated with movement.
The layers of the cerebellar cortex
The next layer, the Purkinje layer, consists of the only output fibres of the cerebellar cortex, the Purkinje cells. The dendrites of Purkinje cells project outwards to the molecular layer. Purkinje cells have recurrent collaterals that inhibit adjacent Purkinje cells and Golgi type II neurons.
Cerebellar cortex is composed of 3 layers and 5 cell types
The cellular interactions of the cortical cells result in one inhibitory output tract, from Purkinje cells which synapses on the deep cerebellar and vestibular nuclei. There are two main input tracts to the cerebellum, each of which is excitatory. The two input tracts consist of climbing fibres, which are actually the axons of neurons which reside in the contralateral inferior olive, and the mossy fibres, which are axons of neurons of a variety of pontomedullary reticular nuclei and axons of neurons in laminae VI and VII of the spinal cord (Ito 1984). As discussed earlier, aminergic neurons in the brainstem also project to the cerebellum.
The climbing fibres first give off collateral projections to the deep cerebellar nuclei before synapsing on granule, Golgi, basket, and Purkinje cells in the cerebellar cortex (Gilman et al. 1981; Van der Want et al. 1989). Only one synapse per Purkinje cell occurs; however, many Purkinje cells are innervated by a single climbing fibre so that a single climbing fibre spike produces a burst of Purkinje cell activity. The mossy fibres influence the Purkinje activity indirectly via synapses on granule cells. The parallel fibres of the granule cells in turn synapse on the Purkinje cells directly or via basket or stellate interneurons. Each parallel fibre excites a long array of about 500 Purkinje neurons, whereas each Purkinje neuron receives input from approximately 200 000 parallel fibres (Gilman 1992) (Fig. 14.12).
All of the cerebellar neurons of the cortex are inhibitory except the granule cells. The Purkinje inhibitory output is exerted on the spontaneously active nuclear cells. Thus, the nuclear cells must have a strong ’pacemaker’ potential or a powerful excitatory input to match the inhibition resulting from the Purkinje cells. The latter excitatory input may be manifested in excitatory impulses from axon collaterals of mossy and climbing fibres that each give off before synapsing in the cerebellar cortex.
Functional regions of the cerebellum
Fastigial efferent fibres can be crossed and uncrossed. The crossed fibres project via the uncinate fasciculus of the superior cerebellar peduncle to the super colliculus bilaterally, and to the interstitial nucleus of Cajal (Ito 1984). Some fibres also project to the ventral lateral and ventral posterior lateral nuclei of the thalamus via the superior cerebellar peduncle. The uncrossed fibres form the fastigiobulbar projections whose bulk forms the smaller juxtarestiform body. These fibres project to all four of the ipsilateral vestibular nuclei and the ipsilateral reticular formation (Batton et al. 1977). The fastigial nuclei fire after the commencement of movement. This nucleus receives large inputs from the periphery and sends few projections to the motor cortex. The fastigial nucleus is involved in the feedback mechanisms of the cerebellum.
The second component originates in the intermediate areas of the cerebellar hemispheres and projects to the interposed nuclei. Some neurons from the interpositus nuclei project through the superior peduncles to synapse on magnocellular neurons of the contralateral red nucleus (Asanuma et al. 1983). The majority, however, synapse on neurons of the contralateral thalamic nuclei of the ventral lateral pars caudalis and ventral posterolateral pars oralis. These thalamic neurons fire almost simultaneously to the primary motor cortex and are involved in efferent copy mechanisms. Efferent copy mechanism compares the intended programme from the cortex to the cerebellum’s knowledge of the state of the organism. Corrections are sent to the brain prior to the movement being carried out. This minimises the time delay in regulating evolving movements in a changing environment.
The inability to carry out a motor programme because of apparent weakness in muscles may in fact be due to a disturbance in midline cerebellar function. An example can illustrate the concept. As an arm or leg is raised or moved away from the body a greater demand is placed on postural mechanisms, which then may fail because of poor reinforcement by medial descending motor pathways from the brainstem and cerebellum.