Chapter 168 Dynamic Stabilization of the Lumbar Spine
Indications and Techniques
Over the past 20 years, developments have been under way to produce spinal systems that control a painful “dysfunctional” spinal unit without causing fusion. Examples of this include facet replacements, interspinous process devices, and pedicle screw–based motion-controlling systems. These latter systems include motion-limiting systems (e.g., the Graf device), mechanical micromotion systems (e.g., Scient’x Isobar TTL), and the Dynesys system. This latter system is the most commonly utilized system in the world today and is reviewed in detail in this chapter. While the theory of these systems is to restore stability yet provide motion, their efficacy has not been completely proved.1 the U.S. Food and Drug Administration (FDA) has not approved any pedicle screw–based system as a stand-alone device for nonfusion applications. The systems available for use, including the Dynesys system and others, are cleared through the FDA 510(k) process for fusion applications as being substantially equivalent to existing pedicle screw–based fusion augmentation devices.
Biomechanical Rationale
Both the spine surgeon and the implant design engineer benefit substantially from a clear understanding of the native function of the spine and the contribution of each of the spinal elements. The motion of the spine can be studied in the most basic form at a single level that begins with the functional spinal unit (FSU). The FSU comprises two vertebral bodies with their articulations, including the intervertebral disc, as well as the two posterior facet joints. The intervertebral disc forms an integral part of the FSU and has a high potential of becoming problematic, especially with age. Anatomically, the disc consists of fibrous layers arranged in alternating lamellar structures, in conjunction with a gelatinous inner core referred to as the nucleus pulposus. The other important articulations within the FSU are the facet joints, which are also susceptible to disease. Facets, in the normal condition, play a role in controlling the motion of the FSU. This three-joint complex within each FSU controls the kinematic response to load. The primary modes of loading taken into consideration are those associated with axial compression, flexion–extension bending, lateral bending, and axial torsion, as shown in Fig. 168-1.
Over time, as degeneration occurs, the disc becomes fibrotic and less able to dissipate and distribute loads. Consequently, nonphysiologic loads are then distributed to the vertebral endplates and the annulus of the disc, which may lead to morphologic endplate changes and annular fissuring. With the onset of the degenerative cascade, both the intervertebral disc and then facets becomes compromised. Currently, it is unclear whether the onset of anterior column degeneration leads to posterior degeneration, and vice versa. Regardless, the degeneration within the FSU may lead to the inability to withstand even physiologic loads, and eventually, depending on the severity, instability may develop. Both clinically and biomechanically, instability can be defined by the inability of the FSU to control physiologic displacement. With instability, the neurologic structures are prone to impingement and injury. Instability of the intervertebral discs shifts greater-than-normal motion and load to the facet joints and ligamentum flavum. With time, these structures all undergo hypertrophy with narrowing of the central neural canal, as well as of the lateral recesses and neural foramina.
Another design parameter that a potential manufacturer needs to address is that of the amount of stiffness required in an individual patient. Many in vitro biomechanical studies have demonstrated that the degree of inherent stiffness in the spine varies among individual specimens. Generally, older cadaveric spine specimens are more rigid than younger spines. Clinically, this has traditionally been assessed by the Kocher Clamp Test, described by Albert Key in 1944 (personal communication). Essentially, the surgeon grasps the posterior spinous processes and pulls. This provides a tactile indication of the spinal stiffness, which Key used to compare to prior experiences. A more objective measure of assessment was described by Brown et al., who utilized an FDA-approved intraoperative spinal stiffness gauge that measured force displacement (Spinal Stiffness Gauge, Mekanika, Boca Raton, FL)2 (Fig. 168-2). Their findings of 655 motion segments demonstrated wide variability in degrees of stiffness. Generally, the L5–S1 segment was stiffer than L4-5, which was stiffer than L3–4. Male patients had stiffer spinal segments than did female patients. Older patients had stiffer spinal segments than did younger patients. Finally, stiffness was found to decrease by 20% following decompression. These basic findings raise the issue of patient-specific requirements and the potential need to customize implants based on intraoperative findings.
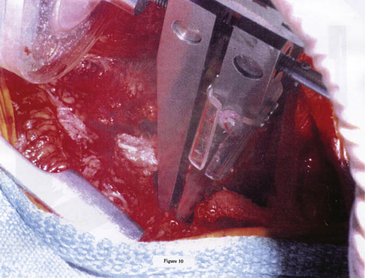
FIGURE 168-2 In vitro biomechanical testing probe placed between posterior spinous processes to determine spinal stiffness.
Another design concern is the amount of stability that an individual patient may require to achieve the best clinical outcome. That is, all other variables being equal, it remains unknown as to the required stiffness in an individual situation that will lead to pain relief and enough stabilization so as to prevent recurrence of symptoms and clinical (and/or potential radiographic) instability.
System Designs with Biomechanical and Clinical Outcomes
Complex biomechanical and clinical needs have been addressed by designers with varying degrees of success. As previously stated, all pedicel screw–based nonfusion stabilization devices are similar in that they anchor an implant that allows for some degree of mobility to pedicle screws. Perhaps the most basic design type was the Graf ligament. This system limited movement in flexion, and had good early clinical results, but ultimately it has not received larger clinical use.3
N-Hance Spine System
The N-Hance spine system is a unique system in that it comprises a solid titanium rod with a sliding component. The sliding component, which attaches to a pedicle screw, has polyurethane bumpers immediately above and below it. This system damps flexion and extension (biomechanically represented by an increase and decrease of the interpedicular distance) against the polyurethane stops (Fig. 168-3). The length of the fixed component of the rod can accommodate span multiple segments so that the system can be utilized for both rigid and nonrigid fixation. This combination of rigid stabilization (acting as a traditional pedicle screw fusion augmentation construct) and nonrigid stabilization (potentially utilized as a nonfusion spinal augmentation construct) is commonly described as a “hybrid” construct.4