Chapter 19 Double Outlet Ventricle
The earliest report that alluded to double outlet right ventricle was published in French in 1703.1 Ninety years elapsed before an English-language publication appeared.2 In 1793, John Abernathy,2 an assistant surgeon at St Bartholomew’s Hospital in London, described “partial transposition” of the great arteries, and in 1898, Karl von Vierordt3 called double outlet right ventricle partial transposition to signify that the aorta was transposed but the pulmonary trunk was normally aligned. It was not until 1957 that Witham4 introduced double outlet right ventricle as a diagnostic term for a partial transposition complex. Witham’s term is now preferred to the synonymous right ventricular origin of both great arteries. Double outlet left ventricle is the rarest of the ventriculoarterial malalignments (see subsequent section).5,6
The question of how best to define double outlet right ventricle has been much debated, and the debate continues without a consensus.7,8 It has been argued that the malformation is virtually unclassifiable because of its excessively complex and diverse anatomy. Should double outlet right ventricle be defined as a connection between the great arterial trunks and the right ventricular mass, or as a malformation in which the leaflets of both great arterial valves are supported by right ventricular infundibular musculature?7 How much overriding of one or the other arterial valves is acceptable? In this chapter, double outlet right ventricle is defined pragmatically as a malformation in which the greater part of the circumference of both arterial valves is supported within a morphologic right ventricle in hearts with two distinct ventricular chambers and concordant atrioventricular connections (Table 19-1).7 The aorta and main pulmonary artery are separated by an outlet septum housed exclusively in the right ventricle. A conus resides beneath each of the two great arterial valves (double conuses), although either conus may be attenuated. The position of the outlet septum establishes two types of infundibular relationships: namely, anterior/posterior and side-by-side. In the more common anterior/posterior relationship, the aorta arises from the posterior infundibulum, and the ventricular septal defect is subaortic. In the less common side-by-side relationship, the pulmonary trunk arises from the medial infundibulum, and the ventricular septal defect is subpulmonary.
Table 19-1 Double Outlet Right Ventricle: Clinical Classification
The More Common Types |
The Less Common Types |
Connections of the great arteries to the ventricles
The aorta is either to the right of and anterior to the pulmonary trunk or side-by-side with the aorta to the right.9 Each great artery arises above a conus that prevents fibrous continuity with atrioventricular valve tissue, but conal attenuation occasionally permits fibrous continuity.9–11 When each great artery is equipped with a separate conus, and both great arteries arise exclusively from the right ventricle, the term double outlet right ventricle is not disputed. However, the appropriate terminology for hearts with a biventricular great arterial valve is unresolved.
Double outlet right ventricle with a subaortic ventricular septal defect, pulmonary stenosis, and an aortic override greater than 50% resembles Fallot’s tetralogy (Box 19-1; see Chapter 18). Double outlet right ventricle with a subpulmonary ventricular septal defect and a posterior non–border-forming biventricular pulmonary trunk resembles complete transposition of the great arteries (see Box 19-1; see Chapter 27). Double outlet right ventricle, Fallot’s tetralogy, and complete transposition have been proposed to represent a spectrum of anomalies resulting from embryonic arrest of the normal rotation of the junction of the outflow tract and the great arteries. In 1949, Taussig and Bing12 published a case of complete transposition of the aorta and levoposition of the pulmonary artery, which arose chiefly from the right ventricle (see subsequent section).
Box 19-1 Double Outlet Right Ventricle: Major Clinical Patterns
Relationship of the ventricular septal defect to the great arteries
A ventricular septal defect is usually subaortic or subpulmonary and provides the left ventricle with its only outlet (Figure 19-1). Less often, the defect is committed to both great arteries, or the ventricular septum is intact (see Table 19-1).9,13,14 Rarely, the defect is in muscular or inlet septum and is therefore not committed to either great artery.15,16
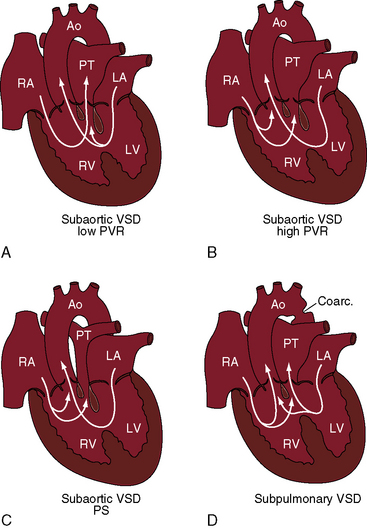
Figure 19-1 Illustrations of four major clinical patterns of double outlet right ventricle (see Table 19-1 and Box 19-1). A, Subaortic ventricular septal defect (VSD), low pulmonary vascular resistance, no pulmonary stenosis. B, Subaortic ventricular septal defect, high pulmonary vascular resistance. C, Subaortic ventricular septal defect with pulmonary stenosis. D, Subpulmonary ventricular septal defect. (Ao = aorta; RA = right atrium; PT = pulmonary trunk; LA = left atrium; LV = left ventricle; RV = right ventricle.)
The location of the ventricular septal defect is the major determinant of intracardiac streaming. When the defect is committed to the aorta, blood flow is preferentially channeled into the aorta (see Figure 19-1A). Streaming is secondarily influenced by pulmonary vas cular resistance and pulmonary stenosis. In the Taussig-Bing anomaly (see previous), which constitutes less than 10% of cases of double outlet right ventricle, the aorta arises completely from the right ventricle, a nonrestrictive ventricular septal defect is subpulmonary, and the pulmonary trunk is biventricular, although principally in the right ventricle.12 Left ventricular blood preferentially enters the pulmonary artery through the subpulmonary ventricular septal defect (Figures 19-1D and 19-2).10–12,17
Obstruction to ventricular outflow
Pulmonary stenosis occurs in 40% to 70% of cases of double outlet right ventricle with subaortic ventricular septal defect (see Figure 19-1C)10,18 and is represented by an underdeveloped subpulmonary conus or by a stenotic bicuspid pulmonary valve.9 A subpulmonary ventricular septal is rarely accompanied by pulmonary stenosis.19 When there is pulmonary artesia (Figure 19-3), the right ventricle has a single functional outlet—the aorta—so double outlet then refers to ventriculoarterial alignment.
A nonrestrictive ventricular septal defect is physiologically advantageous because it provides the left ventricle with an unobstructed exit. An inherently restrictive subaortic ventricular septal defect or a spontaneous decrease in size is a form of subaortic stenosis.20–22 The decrease in size may culminate in complete closure,13,22 or rarely, the ventricular septum is congenitally intact and the left ventricle is hypoplastic.13,23 Aortic stenosis can also be caused by an underdeveloped subaortic infundibulum, which occurs in about 50% of cases with a subaortic ventricular septal defect.
Nearly a third of patients with straddling atrioventricular valves (biventricular insertion of chordae tendineae) have a double outlet right ventricle (see Figure 19-10 and Video 9-1).24 The straddle involves the right-sided or left-sided portion of the atrioventricular valve.24 Overriding refers to biventricular commitment of the atrioventricular annulus, which is not a feature of double outlet right ventricle.
The atrioventricular node is normally located, and the conduction system penetrates the right side of the central fibrous body (see section Electrocardiogram).25 The location of the atrioventricular bundle is related to the ventricular septal defect as in isolated ventricular septal defect (see Chapter 17).
The classification of double outlet right ventricle in Table 19-1 is based on anatomic faults that are principally responsible for the physiologic derangements and clinical expressions of a subaortic or subpulmonary ventricular septal defect and from the absence or presence of pulmonary stenosis or pulmonary vascular disease.
The physiologic consequences of double outlet right ventricle with a subaortic ventricular septal defect and no pulmonary stenosis resemble an isolated nonrestrictive perimembranous ventricular septal defect (see Box 19-1; see Chapter 17). Because the defect is committed to the aorta, left ventricular blood preferentially enters the aorta, and low pulmonary vascular resistance permits a substantial portion of left ventricular blood to stream into the pulmonary circulation and permits right ventricular blood to stream almost exclusively into the pulmonary trunk (see Figure 19-1A). Pulmonary blood flow is increased, and aortic oxygen saturation is virtually normal. As pulmonary vascular resistance rises, right ventricular blood is diverted into the aorta and left ventricular blood is diverted away from the pulmonary trunk (see Figure 19-1B). Pulmonary blood flow declines, and aortic oxygen saturation declines in parallel.
When pulmonary stenosis occurs with double outlet right ventricle, the ventricular septal defect is almost always subaortic. Pulmonary stenosis may initially be absent or mild and then develop and progress. Pulmonary stenosis diverts right ventricular and left ventricular blood away from the pulmonary artery and into the aorta (see Figure 19-1C), so pulmonary blood flow and aortic oxygen saturation fall. The more severe the pulmonary stenosis, the more blood from right and left ventricles enters the aorta; and in the presence of pulmonary atresia, all blood from both ventricles enters the aorta (see Figure 19-3).26 Double outlet right ventricle with a nonrestrictive subaortic ventricular septal defect and severe pulmonary stenosis or atresia physiologically resembles Fallot’s tetralogy (see Box 19-1; see Chapter 18).10,26
When the ventricular septal defect is subpulmonary, left ventricular blood preferentially enters the pulmonary trunk, and right ventricular blood preferentially enters the aorta (see Figures 19-1D and 19-2), so pulmonary arterial oxygen saturation exceeds aortic oxygen saturation. When pulmonary vascular resistance is low, pulmonary blood flow is increased, systemic arterial oxygen saturation is high, cyanosis is relatively mild, and the left ventricle is volume overloaded as in complete transposition of the great arteries (see Chapter 27). A rise in pulmonary vascular resistance diverts right ventricular blood from the pulmonary artery into the aorta. Pulmonary blood flow declines, systemic arterial oxygen saturation falls, and left ventricular volume overload is curtailed (see Figure 19-1D).
Double outlet right ventricle with subaortic ventricular septal defect
The clinical manifestations closely resemble isolated nonrestrictive perimembranous ventricular septal defect (see Figure 19-1A and B and Box 19-1). Male:female ratio is estimated at 1.7:1.27 A large kindred included a second cousin with double outlet right ventricle, a first cousin with complete transposition of the great arteries, and two siblings with truncus arteriosus.28
History
The murmur of ventricular septal defect dates from birth because flow from the left ventricle through the defect is obligatory and does not await the neonatal fall in pulmonary vascular resistance. However, the intensity of the murmur increases as neonatal pulmonary resistance falls and as increased left ventricular stroke volume is ejected through the ventricular septal defect into both great arteries. Increased pulmonary blood flow results in volume overload of the left ventricle, congestive heart failure, and poor growth and development. Cyanosis is mild or absent because left ventricular blood preferentially enters the aorta and right ventricular blood preferentially enters the pulmonary artery (see Figure 19-1A). A rise in pulmonary vascular resistance curtails pulmonary blood flow and relieves the left ventricular of volume overload. Patients occasionally reach young adulthood (Figure 19-4A); one patient underwent intracardiac repair at age 53 years (Figure 19-4B), and one survived to age 65 years.29
Physical Appearance
Transient neonatal cyanosis coincides with transient elevation in neonatal pulmonary vascular resistance. The subsequent fall in pulmonary vascular resistance is accompanied by an increase in pulmonary blood flow, volume overload of the left ventricle, and the catabolic appearance of congestive heart failure. A subsequent rise in pulmonary resistance diverts left ventricular blood from the pulmonary trunk and diverts right ventricular blood into the aorta (see Figure 19-1B)—Eisenmenger’s syndrome—with the appearance of cyanosis and clubbing (see Box 19-1). Double outlet right ventricle is occasionally associated with trisomy 18 and the distinctive overlapping fingers (clinodactyly), rocker-bottom feet, and lax skin (Figure 19-5).30–32
Jugular Venous Pulse
When increased pulmonary blood flow results in biventricular failure, the jugular venous A wave, V wave, and mean pressure are elevated. The height and waveform of the jugular pulse normalize when pulmonary vascular resistance increases. The right ventricle then ejects at systemic resistance with little extra help from the right atrium, as in Eisenmenger’s syndrome (see Chapter 17).
Precordial Movement and Palpation
A hyperactive precordium with Harrison’s grooves result from chronic dyspnea. A right ventricular impulse is accompanied by the impulse of a dilated hypertensive pulmonary trunk and a palpable pulmonary valve closure sound. A thrill generated by the ventricular septal defect is maximal in the third and fourth intercostal spaces at the left sternal border (see section Auscultation).
Auscultation
The first heart sound is soft because the PR interval tends to be prolonged (see section Electrocardiogram).33Low pulmonary vascular resistance results in a holosystolic murmur of ventricular septal defect that is maximal in the third and fourth intercostal spaces at the left sternal border. The pulmonary component of the second heart sound is loud because the hypertensive dilated pulmonary trunk is anterior. The aortic component of the second sound is prominent because the aortic valve lies side by side and to the right of the pulmonary trunk rather than posterior. Inspiratory splitting is preserved as long as pulmonary vascular resistance is less than systemic. Increased flow across the mitral valve generates an apical mid-diastolic murmur.
Elevated pulmonary vascular resistance reduces pulmonary blood flow and left ventricular volume overload (see Figure 19-1B). The murmur of ventricular septal defect becomes decrescendo and is softer (Figure 19-6) but does not disappear because flow from left ventricle into aorta is obligatory. Except for the soft decrescendo murmur, the auscultatory signs are analogous to Eisenmenger’s syndrome with an isolated nonrestrictive ventricular septal defect: namely, a pulmonary ejection sound, a loud single second heart sound, and a Graham Steell murmur of pulmonary hypertensive pulmonary regurgitation (see Chapter 17).
Electrocardiogram
PR interval prolongation is common (Figure 19-7; see previous) because of the unusually long course of the common atrioventricular bundle.33 When pulmonary blood flow is increased, peaked right atrial P waves are associated with bifid broad left atrial P waves (Figure 19-8). Left axis deviation with counterclockwise depolarization is an important feature of double outlet right ventricle with a subaortic ventricular septal defect, no pulmonary stenosis, and increased pulmonary blood flow (see Figure 19-7).25,33 The mechanism of left axis deviation is unknown but cannot be related to an abnormality of the conduction system, which is structurally the same when pulmonary vascular resistance is elevated and there is right axis deviation (see Figure 19-8) and when double outlet right ventricle exists with pulmonary stenosis and right axis deviation.25 The QRS duration is normal, but right ventricular conduction defects sometimes occur, including right bundle branch block.33 Right ventricular hypertrophy is obligatory and is manifested by tall R waves in leads V1 and aVR with deep S waves in left precordial leads (see Figures 19-7 and 19-8). Left ventricular volume overload is indicated by large RS complexes in midprecordial leads and by tall R waves in left precordial leads (see Figure 19-7). Elevated pulmonary vascular resistance is associated with right axis deviation and pure right ventricular hypertrophy (see Figure 19-8).
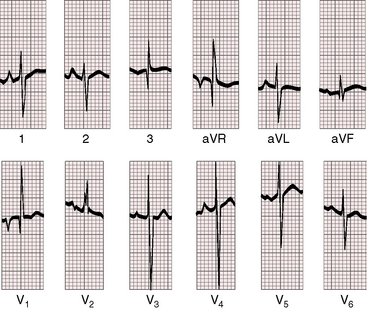
Figure 19-8 Electrocardiogram from a 6-week-old male with trisomy 18 (see Figure 19-5) and double outlet right ventricle, subaortic ventricular septal defect, and moderately elevated pulmonary vascular resistance. Peaked right atrial P waves are present in leads 1 and 2, and a broad bifid left atrial P wave is present in lead V2. Right axis deviation is moderate, and depolarization is clockwise. The q wave in lead V1 indicates right atrial enlargement. Right ventricular hypertrophy is manifested by tall R waves in leads V1