Disorders of vision and visual-perceptual dysfunction
Laurie Ruth Chaikin, MS, OTR/L, OD, FCOVD
After reading this chapter the student or therapist will be able to:
1. Identify and analyze visual anatomy and physiology as they pertain to visual function.
2. Analyze the functional visual skills and how visual dysfunction may affect functional performance.
3. Identify the symptoms of visual dysfunction.
4. Develop the skill necessary to take a visual case history by use of behaviors and clinical observations.
5. Identify the difference between phoria and strabismus.
6. Identify and evaluate the difference between visual field loss and unilateral neglect.
7. Identify and differentiate various pediatric and age-related disease conditions that may affect vision.
8. Clearly differentiate nonoptical and optical assessment and intervention adaptations for patients with low vision.
9. Differentiate basic tools for vision screening.
10. Identify when and why to refer and the tools necessary to document that decision.
Vision is an integral part of development of perception. Some aspects of vision, such as pupillary function, are innate, but many other aspects are stimulated to develop by experience and interaction with the environment. Visual acuity itself has been demonstrated to rely on the presence of a clear image focused on the retina. If this does not occur, a “lazy eye,” or amblyopia, will result. Depth perception develops as a result of precise eye alignment. This ability will be delayed, less precise, or absent if correction of eye misalignment is not done within the first 7 years of life. Research has demonstrated that, in fact, most visual skills such as acuity, binocular coordination, accommodation, ocular motilities, and depth perception are largely intact by age 6 months to 1 year.1 Visual skill development parallels postural reflex integration and provides a foundation for perception.
Anatomy of the eye
Eye chamber and lens
Structures and function are discussed from anterior to posterior (Figure 28-1). The first structure that light hits after it is reflected from an image is the cornea. (Technically, light first hits the tear layer, which has its own structure and rests on the corneal surface.) Corneal tissue is completely transparent. Light is refracted, or bent, to the greatest degree by the cornea because the light rays must pass through different media, which change in density, as in going from air to water.2 The refraction of light can be observed by noting how a stick placed into water appears bent where it enters the water (Figure 28-2).
Damage to the cornea from abrasions, burns, or congenital or disease-related processes can alter the spherical shape of the cornea and disturb the quality of the image that falls on the retina. Radial keratotomy, a surgical procedure done in the 1980s to reduce nearsightedness by placing spokelike cuts in the cornea, sometimes had the side effect of scarring the cornea and causing distorted vision. This surgery is no longer done. The newer surgeries such as laser-assisted in situ keratomileusis (LASIK) are far superior and more predictable in their reduction of refractive error (nearsightedness, farsightedness, or astigmatism) and induce virtually no scarring or distortion. In keratoconus the cornea slowly becomes steeper and more cone shaped, distorting the image and causing reduced vision.3
Iris
Behind the cornea is the iris, or colored portion, which consists of fibers that control the opening of the pupil, the dark circular opening in the center of the eye. The constriction and dilation of the pupil control the amount of light entering the eye in a similar fashion to the way the f-stop on a camera changes the size of the aperture to control the amount of light and the depth of field.4 Under bright light conditions the opening constricts, and under dim light conditions it dilates, allowing light in to stimulate the photoreceptor cells of the retina. This constriction and dilation are under autonomic nervous system (ANS) control, with both sympathetic and parasympathetic components.5 Under conditions of sympathetic stimulation (fight or flight) the pupils dilate, perhaps giving rise to the expression “eyes wide with fear.” Under parasympathetic stimulation the pupils constrict. The effect of drugs that stimulate the ANS can be observed.6 For example, someone who has taken heroin will have pinpoint pupils.
Lens
Behind the iris is the lens. The lens is involved in focusing, or accommodation. It is a biconvex, circular, semirigid, crystalline structure that fine-tunes the image on the retina. In a camera the lens is represented by the external optical lens system. The ability to change the focus on the camera is achieved by turning the lens to change the distance of the lens from the film, which effectively increases or decreases the power of the lens, allowing near or distance objects to be seen more clearly. The same effect, a change in the power of the lens, is achieved in the eye by the action of tiny ciliary muscles, which act on suspensory ligaments, thereby changing the thickness and curvature of the lens. A thicker lens with a greater curvature produces higher power and the ability to see clearly at near distances. A thinner lens and flatter curvature produces less optical power, which is what is needed to allow distant objects to be clear (Figure 28-3). The process of lens thickening and thinning is accommodation.4,5
Ideally the lens will bring an image into perfect focus so that it lands right on the fovea, the area of central vision. If the focused image falls in front of the retina, however, then a blurred circle will fall on the fovea (Figure 28-4). In this case the lens is too thick, having too high an optical power. One simple remedy is to place a negative (concave) lens externally in front of the eye in glasses (or contact lenses) to reduce the power of the internal lens and allow the image to fall directly on the fovea. In presbyopia (old eyes), the flexibility of the lens fibers decreases and the lens becomes more rigid.7 Accommodation gets weaker until the image can no longer be focused on the retina. Normal-sighted individuals first begin to notice these changes in their early forties. When this occurs, a plus (positive) lens (or bifocals, progressive lenses, bifocal or monovision contact lenses) may be worn to aid in reading.4
Vitreous chamber
The space behind the lens, which is filled with a gel-like substance, is called the vitreous chamber. As we age, the gel tends to liquefy, and some of the remnants of embryological development that were trapped are released to float freely. This can cause the very common perception of “floaters,” the shadows cast by these particles onto the macular region. They can be disturbing but generally float out of view over time.5
Retina
The retina at the back of the eye is the photosensitive layer, like the film in a camera, receiving the pattern of light reflected from objects. The topography of the retina (Figure 28-5) includes the optic disc, which is where the optic nerve exits and arteries and veins emerge and exit. This is also the blind spot because there are no photoreceptor cells on the disc. The macula is temporal to the optic disc and contains the fovea, providing central vision. The surrounding retina provides peripheral vision and defines a 180-degree half-sphere.5
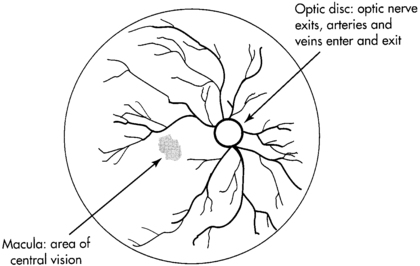

Visual pathway
The visual pathway begins with the photoreceptor cells, which begin a three-neuron chain exiting through the optic nerve. This chain consists of the rods and cones, which synapse with bipolar cells that synapse with ganglion cells (Figure 28-6).5,8
The phenomenon responsible for the high degree of neural representation of the foveal region and that accounts for the tremendous conscious awareness of the central view is called convergence.5 At the periphery of the retina the degree of convergence is great; many photoreceptor cells synapse on one ganglion cell, which accounts for poor acuity but high light sensitivity. The closer to the macula, the less the degree of convergence, until, finally, at the fovea there is no convergence. This means that one photoreceptor cell synapses with one bipolar cell and one ganglion cell.
The awareness of what is seen is directly related to the amount of convergence, which reflects the extent of neural representation. The 1:1 correspondence between photoreceptor and ganglion cell at the fovea means that there is a high degree of neural representation of the foveal image in the brain. It is even greater than the neural representation of the lips, tongue, or hands.9 This accounts for the primary awareness of what is in the foveal field and secondary awareness of the peripheral field. Conscious awareness of the environment is whatever is in the foveal field at the moment. But continuous information about the environment is flowing over the peripheral retina, usually subconsciously. Attention quickly shifts from foveal to nonfoveal stimulation when changes in light intensity or rapid movement are registered. This type of stimulus arouses attention immediately because it could have specific survival value. For example, a person is driving down the street and senses rapid motion off to the right. The foveas swing around immediately to identify a small red ball bouncing into the street. This information goes to the association areas, in which “small ball” is associated with “small child soon to follow.” Frontal cortical centers are aroused and a decision is made to initiate motor areas to take the foot off the accelerator and put it onto the brake, while simultaneously moving the wheel away from the ball and scanning for the object of concern, that is, the child.
Exercise 28-3: peripheral central awareness
The moment light hits the retina, the photographic film model must be abandoned for the image processing or computerized image enhancement model. The primary visual pathway at the retinal level is a three-neuron chain. From back to front the first neuron is the photoreceptor cell, rods or cones. They synapse with a bipolar cell, which in turn synapses with a ganglion cell. The axon of the ganglion cell exits by means of the optic nerve. Image enhancement occurs at the two junctions of the three–nerve-cell pathway. Lateral cells at the neural junctions have an inhibitory action on the primary three-neuron pathway, and through the inhibition of an impulse the image is modulated. For example, at the first junction between photoreceptor cell and bipolar cell, there are horizontal cells. These cells enhance the contrast between light and dark by inhibiting the firing of bipolar cells at the edge of an image. This makes the edge of the image appear darker than the central area, which increases the contrast and thereby increases attention-getting value. After all, it is by perceiving edges that we are able to maneuver around objects. In a similar manner, amacrine cells act at the second neural junction between bipolar and ganglion cells to enhance movement detection.10
The visual pathway continues through the brain (Figure 28-7). The ganglion cell axons exit the eyeball by means of the optic nerve, carrying the complete retinal picture in coded electrochemical patterns. From there the patterns project to different sites within the central nervous system (Figure 28-8). Projections to the pretectum are important in pupillary reflexes; projections to the pretectal nuclei, the accessory optic nuclei, and the superior colliculus are all involved in eye movement functions.5 The largest bundle, called the optic tract, projects to the lateral geniculate body in the hypothalamus, where additional image enhancement and processing occurs. The next group of axons continues to the primary visual cortex and from there to visual association areas.
At what point does the retinal image become a perception, and with what part of the brain does one see? Current theory regarding visual perception is the result of Nobel prize–winning research by Hubel and Wiesel in the 1960s called the receptive field theory.11 This theory states that different neurons are feature detectors, defining objects in terms of movement, direction, orientation, color, depth, and acuity. Research in 1990 by Hubel and Livingstone12 was able to locate a segregation of function at the level of the lateral geniculate body. They identified two types of cells, one type being larger and faster magno cells, which are apparently phylogenetically older and color blind but which have a high contrast sensitivity and are able to detect differences in contrast of 1% to 2%. They also have low spatial resolution (low acuity). They seem to operate globally and are responsible for perception of movement, depth perception from motion, perspective, parallax, stereopsis, shading, contour, and interocular rivalry. Through linking properties (objects having common movement or depth) emerges figure-ground perception. Much of this perception occurs in the middle temporal lobe.
Eye movement system
The eye movement system consists of six pairs of eye muscles: the medial recti, lateral recti, superior and inferior recti, and superior and inferior obliques (see Figure 28-8). Together they are controlled by cranial nerves III (oculomotor), IV (trochlear), and VI (abducens). The eye movement system has both reflex and voluntary components. Reflexive movements are coordinated through vestibular interconnections at a midbrain level. The vestibuloocular reflex (VOR) functions primarily to keep the image stabilized on the retina. Through connections between pairs of eye muscles and the semicircular canals, movement is analyzed as being either external movement of an object or movement of the head or body. From this information the VOR is able to direct the appropriate head or eye movement.5
Functional visual skills
Refractive error
Before discussing binocular coordination and the individual visual skills, it is important to describe refractive errors and how they can affect binocular coordination. Three common types of refractive errors are myopia or nearsightedness, hyperopia or farsightedness, and astigmatism.5,10
The following are examples of different refractive errors:
−5.50 DS (diopter sphere): myopia
+1.50 c¯ − 1.50 × 180: astigmatism (Note: × stands for the axis of the cylinder correction.)
Binocular coordination is the end result of the efficient functioning of the visual skills (Box 28-1). The individual visual skills include accommodation, eye alignment or vergence, eye movements with normal vestibular coordination, stereopsis (depth perception), and peripheral and central coordination. During normal activities, all the skills are inseparable.
Accommodation
Accommodation is the ability to bring near objects into clear focus automatically and without strain. Relaxation of accommodation allows distant objects to come into focus. The primary action is that of the ciliary muscles acting on the lens, and the primary system of control is the ANS, with sympathetic and parasympathetic components.5
Both accommodation and pupil size changes are reflexes that work in concert: as accommodation relaxes the pupil dilates and as accommodation increases the pupil constricts.4 As a person focuses on a near object, the lenses thicken, allowing the near object to come into focus. At the same time the pupils constrict to increase depth of focus (just as in a camera). As a person looks into the distance, the lens gets flatter, relaxing accommodation, and the pupil dilates, decreasing the depth of field.
Pursuits and saccades
Problems in pursuits or saccades can be the result of a dysfunction of any individual muscles, the VOR, or areas of the brain controlling pursuits or saccades.13–15 Because the VOR helps to stabilize the image on the retina and to differentiate image movement from eye movement, simple tracking can be more difficult. In addition, visual field loss, either central or peripheral, can dramatically affect localization ability. People with blind half- or quarter-fields can be observed to do searching eye movements rather than directly jumping to the object.
Memory also may be affected by an eye movement dysfunction. Research by Adler-Grinberg and Stark16 and Noton and Stark17 examined patterns of eye movements as subjects looked at a picture. Distinct eye movement patterns, called scan paths, became apparent. When the subject was asked to recall the picture, the same eye movement pattern was elicited as when the subject originally saw the picture. It would appear that a type of oculomotor praxis is involved in recall. Applying this idea to the clinical setting, if a patient has inaccurate eye movement with poor pursuits or excessive saccades, then perhaps the stored memory is less efficiently stored and consequently more difficult to reconstruct from memory. Additionally, if a patient has a type of brain damage with generalized dyspraxia, the eye movement system could quite likely be affected and might be involved in the patient’s perceptual dysfunction.
Another more recent example of the relationship between eye movements and memory is the use of eye movement desensitization and reprocessing (EMDR) therapy to help individuals with posttraumatic stress disorder reintegrate traumatic experiences.18 Although the exact mechanism is at this time unknown, the prevailing hypothesis is that the lateral eye movements elicit an orienting response, scanning the environment for further danger, and that this is an investigatory reflex associated with a relaxed physical state.19
Symptoms of visual dysfunction
History
Are you having difficulty with seeing, or with your eyes?
Do you wear glasses? Contact lenses? For distance, near, bifocals, or monovision (one eye near, other distance)?
Does your correction (glasses, contact lenses) work as well now as before the (stroke, accident, and so on)?
Have you noticed any blurriness? Near or far?
Do you ever see double? See two? See overlapping or shadow images?
Do you ever find that when you reach for an object that you knock it over or your hand misses?
Do letters jump around on the page after reading for a while?
Are you experiencing any eye strain or headaches? Where and when?
Do you ever lose your place when reading?
Are portions of a page or any objects missing?
Do people or things suddenly appear from one side that you did not see approaching?
Clinical observations include the following:
Head turn or tilt during near tasks, or postural adjustments to task
One eye appears to go in, out, up, or down
Eyes appear red, puffy, or irritated or have a discharge (Notify nurses or physician of these observations.)
Has difficulty maintaining eye contact
Spaces out, drifts off, daydreams
During activity, neglects one side of body or space
During movement, bumps into walls or objects (either walking or in a wheelchair)
Phoria and strabismus
Esophoria: The eyes are postured in front of the point of focus.
Exophoria: The eyes are postured in back of the point of focus.
Phoria is measured in units of prism diopters, which indicate the size of the prism needed to measure the eye position in or out from the straight-ahead position.4
The essential concept in understanding the difference between phoria and strabismus is that in strabismus fusion and depth perception are not present. Definitions of different types of strabismus are presented in Box 28-2. It is not a conclusive list; many other types and permutations are beyond the scope of this discussion. The intent here is to expose the therapist to different terms that may be used by the physician in diagnosing the type of strabismus.
Many convergence problems are amenable to vision therapy,20–22 but some are not.23 Whether a particular problem can be helped by vision therapy can be determined by an eye doctor, who can prescribe specific exercises.
Oculomotor dysfunction
Oculomotor dysfunction is a very common sequela of neurological deficits, with an incidence as high as 90% according to Ciufredda and colleagues.24,25 Commonly the smooth pursuit system will be affected, such that the smooth movement is interrupted by a series of fixation stops and the movements appear jerky. Damage anywhere along the visual motor pathway may cause a variety of eye movement disorders. This includes injury to the pontine and mesencephalic reticular formation, oculomotor nucleus in the brain stem, caudate nucleus and substantia nigra, cerebellum, and vestibular nuclei.24
As mentioned previously, detection of peripheral targets serves to direct an eye movement with a specific velocity and direction to bring the foveas in line for purposes of identification. Therapy for rehabilitation of eye movement disorders should be directed at using peripheral awareness with slow controlled eye movement toward the target. Once these movements are tolerated, head movement can be added, then slowly body movement.26,27
Visual field defects—hemianopsia and quadrantanopsia
Visual field loss may indicate damage that is prechiasmic, at the optic chiasm, postchiasmic, in the visual radiations of the thalamus, or in the visual cortex. The resultant visual field loss is characteristic (even diagnostic) in each case. The visual field loss pattern will generally reflect the location of the lesion. It could be bitemporal (outer half of each field), half-field loss (hemianopsia) with or without macular involvement, or quarter-field loss (see Figure 28-7). Some symptoms of field loss are an inability to read or starting to read in the middle of the page, ignoring food on one half of the plate, and difficulty orienting to stimuli in a specific area of space.
Hemianopsia is a loss of half of the visual field in each eye, and quadrantanopsia is loss of a quarter of the visual field in each eye. Homonymous hemianopsia refers to the inner or nasal half and the outer or temporal half of each eye being affected. The retina itself is intact, but a neurological lesion has interrupted the ability of the visual cortex to receive recognition of the image. Vision processing may be occurring at lower centers, such as the lateral geniculate body, but if signals are not being received by the cortex, then they are not recognized as “seen.” In 1979, Zihl and von Cramon28 published their findings that damaged visual fields could be trained by use of a light stimulus presented repeatedly at the border of the visual field defect. Balliet and co-workers29 (when attempting to repeat the experiment, adding controls for oculomotor fixations) proposed that subjects were actually learning to make small compensatory eye movements rather than experiencing true improvements in the visual fields. In the 1980s and 1990s a group of German researchers developed a computer-based field training system for researching the question of visual field training. They found in their research that visual fields did expand on average by 5 degrees, with functional improvements noted by more than 80% of their patients (Figure 28-9).30–34 A company called NovaVision introduced the computer-based visual field restitution training program in the United States with good results (see Appendix 28-A). This author has also noted documentable and functional improvements in visual fields even when trained with less sophisticated methods.
Summary of disorders of vision
Table 28-1 summarizes primary visual deficits. Once a therapist or other specialist has eliminated the possibility of primary visual deficits, the clinician must assess whether the identified problem is resulting from central associative processing that is causing visual-perceptual dysfunction.
TABLE 28-1
VISUAL DEFICIT | FUNCTIONAL DEFICIT | MANAGEMENT | TREATMENT |
Decreased visual acuity (distance or near) | Decreased acuity for distance or near tasks (reading) | Provide best lens correction for distance and near vision |
Copyright by Mary Jane Bouska, OTR/L, 1988. Modified by Laurie R. Chaikin, OD, OTR/L, FCOVD.
Eye diseases
Pediatric conditions
Retinopathy of prematurity
The incidence of retinopathy of prematurity is increasing because of the improved survival of premature infants as a result of improved ventilation.35 Immature retinal vessels are sensitive to high oxygen tension. The effect on the vessels is vasoconstriction, eventually leading to obliteration of the vessels. This creates a state of ischemia, which stimulates the growth of new blood vessels. These small, fragile vessels bleed easily, leading to fibrosis and traction on the retina. As a result of the traction, the macula gets stretched, interfering with the function of central vision.
The temporal vessels are most affected because they develop last. The degree of damage may be mild or severe, depending on the amount of prematurity.7
Cerebral palsy
Therapists who work with children with cerebral palsy may have noticed a high incidence of vision problems. Many studies confirm these observations. A study by Scheinman36 examining the incidence of visual problems in children with cerebral palsy and normal intelligence found the following incidences: strabismus in 69%, high phorias in 4%, accommodative dysfunction in 30%, and refractive errors in 63%.
Age–related conditions
Cataracts.
In the senile cataract the lens slowly loses its ability to prevent oxidation from occurring, and liquefaction of the outer layers begins. The normally soluble proteins adhere together, causing light scatter.3 Vision slowly declines as opacification and light scatter increase, until the lens must be removed.
Age-related macular degeneration.
Age-related macular degeneration (AMD) is the leading cause of blindness in the Western world and is the most important retinal disease of the aged (affecting 28% of the 75- to 85-year-old age group).7
Loss of central vision results from fluid that leaks up from the deeper layers of the retina, pushing the retina up and detaching it from the nourishing layer. New vessel growth and hemorrhage and atrophy further destroy central vision. There is much research going on regarding treatments for AMD. The most promising at this time is the use of bevacizumab (Avastin) or ranibizumab (Lucentis), which is injected into the eye; then the eye is treated with a laser. The drug targets the neovascular network of blood vessels, and the laser treatment obliterates the vessel network, sparing the photoreceptors.37
Glaucoma.
Glaucoma occurs in 7.2% of the 75- to 85-year-old age group.7 It is generally caused by an increase in the intraocular pressure. This pressure interferes with the inflow and outflow of blood and nutrients at the optic disc. As it progresses, glaucoma can cause tunnel vision and, in some, complete blindness. Because of the type of vision loss affecting the periphery, mobility and safety are significantly impaired. Try walking around holding a paper towel tube to your eye while closing the other eye, and see what happens to your ability to maneuver around obstacles or find your destination.
Visual field loss.
Visual field loss may be either central (macular degeneration, glaucoma, or retinal disease) or peripheral field loss from glaucoma, retinal damage, or stroke at any point in the visual pathway. This is potentially the most functionally disabling form of visual impairment (see Figure 28-7).
Implications for functional performance
Lighting
Low-vision AIDS
Telescopes.
The implantable telescope is an exciting new option available for patients with end-stage AMD. After careful evaluation the patient may be considered to be a good candidate for implantation. The tiny telescope is surgically implanted near the lens inside the eye. It has the benefits of having magnification immediately available for use for distance targets and reading; however, the peripheral vision in the implanted eye is significantly reduced. Similar to someone adjusting to monovision contact lenses, the patient with the implanted telescope learns to look through either the telescopic eye or the other eye (Figure 28-10).38–40
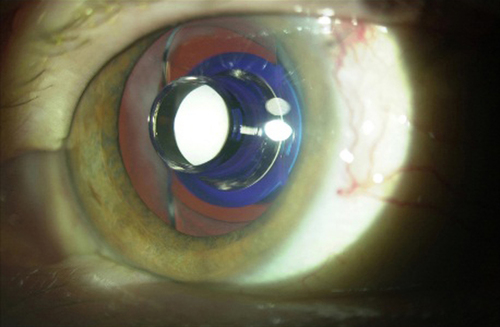

Electronic digital magnifiers.
The best systems for severely impaired clients are the electronic digital magnifiers such as closed-circuit television (CCTV). The CCTV system consists of a camera housed in a device that can be directed at the object to be viewed. The scope of magnification is significantly larger, ranging from low power to 50×. Additional benefits include no distortion like that caused by optical magnifiers and a field of view limited only by the size of the screen. The housing for the camera may be in a stand, with a screen above, or portable, held in the hand or strapped to the head. Examples are the Merlin, Jordy, and MaxPort by Enhanced Vision Systems and the portable digital magnifiers such as Compact mini by Optelec.41
Current research.
Areas of research have included mounting a video camera onto spectacles and then transducing the visual information to electrodes implanted in visual cortical centers. In one study this system allowed a low-vision patient to see the large E (20/400) and detect large contours.42–44 Recently a company called Second Sight Medical Products developed the Argus II, which includes implantation of a 60-electrode grid on the retina, which is used in conjunction with a video camera mounted on eyeglasses. A wireless microprocessor with battery pack is worn on the waist. Altogether the system enables rudimentary perception of shapes and forms, allowing improved mobility in patients whose vision has been impaired by retinal diseases such as retinitis pigmentosa.45
Visual screening
Primary visual dysfunction must be differentiated from a visual-perceptual disorder so that appropriate treatment can be addressed for each problem. Gianutsos and colleagues46 found that more than half the individuals in their study admitted for general head injury rehabilitation who were eligible for cognitive services had visual sensory impairments sufficient to warrant further evaluation. Visual screening can identify the need for referral for a complete eye examination. The results of the examination become part of the differential diagnosis regarding a perceptual dysfunction. Box 28-3 presents key elements in vision screening.
Acuities: Acuities should always be tested first because decreased acuities will bias other tests except for ocular motilities and the peripheral field test.
Positioning: The body and head should be in good alignment or straightened with positioning devices, with the head in midline.
Glasses: If the client normally wears glasses, for either distance or near vision, the patient should be wearing glasses for tests for which spectacle correction is required. When in doubt, try it both ways, record the best response, and note whether glasses were worn.
Distance acuities
Equipment
Record
If the patient is unable to see the top line at 20 feet, the patient is asked to move forward until able to identify the top letters. Then the distance and letter size (top line) are recorded. For example, if the patient had to move up to 4 feet to see the top line, then 4/100 is recorded. To calculate 20-foot equivalence, an equation is used where x equals the size of the letter (e.g., 4/100 = 20/x); thus, 4x = 2000 and 2000/4 = 500. The client’s vision is 20/500 (Box 28-4).
For clients whose attention is poor, the testing distance may need to be as close as 2 feet. Other testing stimuli can be used for children, such as the Broken Wheel Test* or the Lighthouse cards.* Acuity in low-functioning clients or infants can be evaluated by use of preferential looking methods. Targets are usually high-contrast grating patterns of decreasing size. One such type is the Teller cards.†
Pursuits
Equipment
Any target that holds the patient’s attention can be used, such as a pencil or small toy.
Procedure
One pencil is held 16 to 20 inches in front of the client, and the client is asked to look directly at one part, such as the eraser, and to keep the head still, holding it if necessary. The pencil is moved around in the pattern shown in Figure 28-11, which is designed to incorporate all directions of gaze. The examiner should observe for smooth following, noticing and recording jerks and jumps, where they occur, or if the eyes stop at a certain point. If one or both eyes stop tracking, the client is encouraged to look at the pencil. If the patient is unable to do this, then the movement pattern is repeated with each eye separately and where the movement stops is recorded. Clients who have had a cerebrovascular accident (CVA) or head injury should be tested first monocularly (each eye separately).
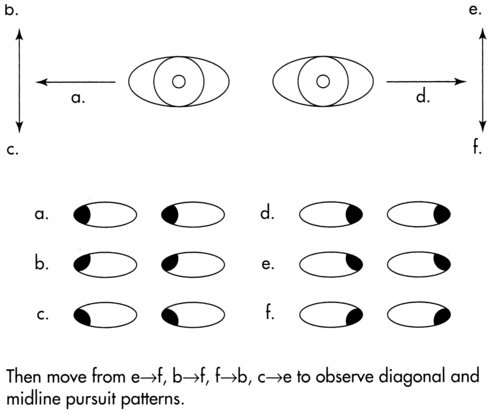

Saccades
Equipment
Tracking pencils can be used, although a few saccadic tests are available. One is the King Devick Saccadic Test; the other is the Developmental Eye Movement Test.* These both require form perception (number reading) and may be difficult, depending on the client’s cognitive level.
Procedure
This call-shift is repeated about 10 times, moving into different fields of gaze. The screener continues until the client is seen to respond. The screener observes for overshooting or undershooting the target, for the ability to isolate the eyes from the head (hold head still), for controlled eye movement, and for ability to wait until the verbal command to look. It is important to observe for the client’s ability to shift to all fields of gaze. A lower level of testing would be to ask the client to move the eyes from one target to the other as quickly as possible (Figure 28-12).
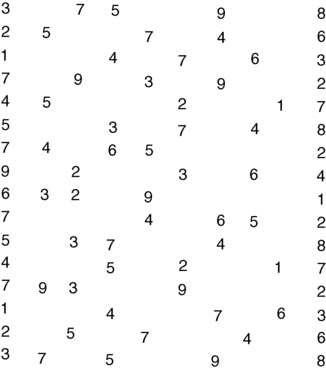

Record
Poor = Inability to control eyes with verbal command, consistent undershooting or overshooting, inability to isolate eyes from head
Fair = Ability to maintain eyes on target with verbal command 50% of the time, with slight undershooting or overshooting, and ability to isolate eyes from head with verbal reminders
Good = Ability to follow verbal commands 90% of the time, with no undershooting or overshooting, and complete eye from head isolation
Near point of convergence
Procedure
Implications
An eye movement problem may be the result of direct damage to the eye muscles themselves (Figure 28-13) or to the nerves controlling them, as in the case of a head injury. Damage to the vestibular center also may involve visual components. Neurons from cranial nerves III, IV, and VI synapse in the vestibular nuclei. Reflex control of eye movements occurs through the VOR and the optokinetic system.
Cover tests
Purpose
Far cover tests
The preceding procedure is repeated with the patient looking at a distant target.
Interpretation and referral.
A visible eye movement may be part of a post-CVA client’s premorbid pattern. This should be determined by asking the client or the family members before making a referral. Or the condition may be the result of neurological damage to cranial nerve III, IV, or VI from CVA, head injury, or cerebral palsy. Eye muscles are striated muscles, under voluntary control. Like other striated muscles that can be affected by neurological damage, they may recover spontaneously, they may not recover at all, or they may benefit from visual retraining. Many learning-disabled children with vestibular dysfunction have poor binocular skills. An ophthalmologist or optometrist specially trained in visual remediation can determine a patient’s potential for vision therapy. Some published research has demonstrated the success of vision therapy for post-CVA patients.47
Referral considerations
It is not always clear when to refer a client or to whom. Many doctors do not test all areas of visual function. Generally, behavioral or developmental optometrists have a functionally oriented philosophy quite similar to occupational therapy models of functional performance.*
Recommended referral guidelines are shown in Box 28-5.
Rehabilitation optometric evaluation
Oculomotilities will be evaluated grossly, and more specific tests may be done. One test is the Developmental Eye Movement Test (see Figure 28-12). Another test is the Visagraph (Figure 28-14). This instrument records eye movements while the client is reading text. It will measure total reading rate, number of fixations and regressions per 100 words, span of recognition, and reading comprehension. The tool is excellent for in-depth evaluation and monitoring the progress of treatment over time.
Ocular health testing will include glaucoma testing, examination for cataracts, and retinal health evaluation. For visual field testing, either a screening field or a threshold visual field will be done on some type of automated perimeter such as the Humphrey Visual Field Analyzer or the Octopus. Threshold testing is done to determine the extent and depth of a defect, and it can help to determine whether there is potential for visual field retraining. In Figure 28-9 the black portions of the visual field are areas of absolute damage. The areas of white with small dots are intact visual fields. At the border of the damage area is a gray zone, which theoretically is amenable to training.30–33
Visual intervention
Early intervention is recommended when possible to identify ways in which a vision problem may be interfering with other therapies.47–50 Some treatments may be applied early on, as well. For example, if the client has an eye muscle paresis, range of motion exercises to the involved muscle can prevent the development of a contracture of the unopposed muscle.
Therapeutic considerations
Accommodative dysfunction
If the client has an accommodative dysfunction, the treatment may be the prescription of glasses for reading or tabletop tasks or possibly near-far focusing exercises,4 depending on the age of the client. “Flipper bars” are special lenses that exercise the focusing system.51
Visual dysfunction and balance disorders
Therapy may be directed at both areas: improving balance by enhancing balance and proprioceptive mechanisms and/or by enhancing visual input through visual rehabilitation techniques. There has been recent research involving the strategic placement of small weights on the torso, which has had immediate and sometimes dramatic effects on balance.52,53 Interestingly, these types of inputs to the torso can also affect visual processing (observations by BalanceWear vest developer Cindy Gibson-Horn, RPT, and me). It is hypothesized that the mechanism for this change may be mediated by cerebellar-visual pathways.54
Visual-perceptual dysfunction
Problems of unilateral spatial inattention
Identification of clinical problems
General category.
In its purest form, unilateral spatial inattention is defined as a condition in which an individual with normal sensory and motor systems fails to orient toward, respond to, or report stimuli on the side contralateral to the cerebral lesion. Although this condition is not often seen in its pure form, inattention has been documented in persons who demonstrate no accompanying visual field defect (homonymous hemianopsia) or limb sensory or motor loss.55 In most cases, however, unilateral spatial inattention is not seen alone but is associated with (although not caused by) accompanying sensory and motor defects such as homonymous hemianopsia and decreased tactile, proprioceptive, and stereognostic perception along with paresis or paralysis of the upper limb.56
Unilateral spatial inattention occurs most frequently in individuals with a diagnosis of stroke (CVA), traumatic brain injury, or tumor. Most authors agree that unilateral spatial inattention occurs more often with right hemisphere than with left hemisphere lesions.57–61 This frequency supports theories that the right hemisphere is dominant for visual-spatial organization. It is clear, however, that inattention may be present in individuals with left hemisphere lesions, but that the inattention tends to resolve more quickly.62 The clinician should remember that, although the chances are statistically lower, the client with right hemiplegia may exhibit inattention to right stimuli.
Unilateral spatial inattention has been associated with lesions in both cortical and subcortical structures. It is most commonly seen in inferior parietal lobe lesions60 but has also been observed in lesions in the inferior frontal cortex, the dorsolateral frontal lobe, the superior temporal gyrus, and the cingulate gyrus63 and with basal ganglia, thalamic,64 and putaminal hemorrhage.65–67 Finally, lesions in the brain stem reticular formation have induced inattention in cats66 and monkeys.64
Although a number of theories have been postulated regarding the mechanism underlying unilateral spatial inattention, no mechanism has been validly documented in human subjects. The one fact that is clear from all theoretical postulates is that inattention is a hemispheric deficit. LeDoux and Smylie68 demonstrated this point effectively in an interesting case study of a right-sided lesion. During full visual exposure (bilateral hemispheric) of visual-perceptual slides, the affected individual made visual-spatial errors in left space. However, when the same slides were directed only to the right visual field (left hemisphere), performance improved substantially. It is as if the deficient hemisphere fails to receive or orient toward incoming information while the intact receiving hemisphere remains oblivious and goes about its own business. Treatment for inattention is problematic mainly because the mechanisms underlying unilateral spatial inattention are not clearly understood.
Theories on mechanisms underlying unilateral spatial inattention have attempted to explain it as an integrative associative defect as opposed to simply a problem of decreased sensory input. Theories include a unilateral attentional hypothesis, suggesting that inattention results from a disruption in the orienting response—that is, the corticolimbic hemisphere is underaroused during bilateral input, and therefore stimuli presented to that hemisphere are neglected.60,63 Another theory is the oculomotor imbalance hypothesis, which suggests that individuals with inattention have a visual-spatial disorder worsened by oculomotor imbalance. The hypothesis suggests that the lesion disconnects the frontal eye fields in the damaged hemisphere from their sensory afferent nerves, resulting in an oculomotor imbalance deviating the gaze toward the lesion. This imbalance can be compensated for only momentarily by a voluntary effort to gaze toward the opposite hemispace (i.e., neglected space).69
Behavioral manifestations of unilateral spatial inattention.
Inattention, like all other perceptual disorders, may occur on a scale from mild to severe. Mild cases of inattention may go unrecognized unless behavior is carefully observed. Scanning is symmetrical except during tasks requiring increasingly complex perceptual and cognitive demands. Leicester and colleagues70 believe that inattention occurs mainly when the individual has a general perceptual problem with the material, that is, some other problem with processing the task. This performance difficulty or stress brings on the additional inattention behavior; for example, neglect in matching auditory letter samples is more common in those with aphasia than in those with right hemisphere involvement without aphasia.
Assessment
Cancellation tasks. The client may be given a sheet of paper with horizontal lines of numbers or letters and asked to cross out all the 8s or As.
Crossing-out tasks. In this standardized test the client is asked to cross out diagonal lines drawn at random on an unlined sheet of paper.
Line-bisection tasks. The client is asked to bisect a 4- to 8-inch line on a piece of paper placed at the midline.
Drawing and copying tasks. The client may be asked to draw or copy a house, clock, or flower or to fill in the numbers of a clock drawn by the examiner. For copying tasks, it is important that the copy be placed in the client’s attended space.
Clients with inattention demonstrate one or more of the following behaviors: failing to cancel figures or cross out lines in the unattended space; bisecting the line unequally, placing their mark toward the side of the midline ipsilateral to their lesion; placing their drawing toward the edge of the paper ipsilateral to their lesion rather than in the middle of the page; drawing only the right or left half of the house, flower, or clock; crowding all the numbers of the clock into the right or left half of the clock; or completing numbers on only one half of the clock (Figure 28-15), and demonstrating differences in reaction time.71 When interpreting performance, the examiner is looking specifically for asymmetries in performance. Clients with inattention often have other visual-perceptual deficits that result in faulty performance on these tasks; however, these deficits are always symmetrical, that is, evident in any space to which the individual attends.
Asymmetries in performance should be carefully observed during functional activities such as eating, filling out a form, reading, dressing, and maneuvering through the environment. The therapist may note unawareness of doorways and hallways in the unattended space; turns may be made only toward one direction. As a result, these clients lose their way in the hospital or even in the therapy clinic. This behavior should be distinguished from a topographical perceptual deficit in which the individual cannot integrate or remember spatial concepts well enough to find his or her way without getting lost. The Behavioral Inattention Test has recently been published as a standardized measure of functional inattention.72
Finally, various studies have shown that inattention may occur during testing that requires visual processing and therefore may invalidate test results.57,73,74 Unresponsiveness to figures on one side of the page during visual, perceptual, cognitive, or language assessments may be subtle but must be documented to rule out the influence of inattention on raw score; that is, if the patient did not see the entire test display for an item, that test item is invalid. Responses to figures on the right half and left half of the test page should be counted. If the frequency of answers is noticeably less on one half of the page than would normally be expected, inattention may have occurred during testing. This may be used as additional evidence of inattention; but more important, this factor should be accounted for when computing the test score. Only those test items in which the correct answer was located in the attended space should be scored; that is, only those items in which the correct answer was right of midline in a client with left inattention should be scored.
Interventions
As previously stated, the mechanisms underlying unilateral spatial inattention are not well understood; however, recent research has uncovered a strong correlation between nonspatial aspects of attention called tonic and phasic attention and spatial aspects of neglect. Tonic attention is intrinsic arousal that fluctuates on the order of hours to minutes and contributes to sustained attention and preparation for more complex cognitive tasks. Phasic attention is a rapid change in attention in response to a sudden and brief event and is related to orienting responses and selective attention.75 This research has postulated that nonspatial attention mechanisms affect spatial and nonspatial behavior. Some highly successful training protocols using these attentional mechanisms have been developed, and the researchers have been able to demonstrate improved responses with carryover in the environment (see Appendix 28-A). They also demonstrated that this remediation approach was more effective than just scanning strategies. A number of studies have investigated the remediation of unilateral spatial inattention. They have attempted to (1) define effective remediation techniques and (2) measure changes in trained tasks and generalization to untrained tasks, that is, determine whether inattention training in one task carries over to other unrelated tasks such as activities of daily living. Treatment techniques used in all these studies resulted in less inattention during trained tasks.58,76,77 An overview of these studies suggests that training may decrease inattention, although extent of change and generalization to other tasks may vary widely. Discrepancies in these results may be related to neurological variables in the various client samples, severity of inattention, sample size, or tasks measured. A discussion of general principles of remediation follows.
Diller78 has designed a number of specific training techniques to decrease inattention during reading and paper and pencil tasks. With a little creativity, these techniques may be applied to other activities. For example, when the client is reading, a visual marker is placed on the extreme edge of the page in unattended space. The individual is instructed not to begin reading until he or she sees the visual marker. The marker is used to “anchor” the client’s vision. As inattention decreases, the anchor is faded. Each line may also be numbered and the numbers used to anchor scanning horizontally and vertically. To control impulsiveness, which often accompanies inattention, clients are taught to slow down or pace their performance by incorporating techniques such as reciting the words aloud. Underlining and looping letters or words can also be used as a method to slow down impulsive scanning (Figure 28-16). Finally, the density of stimuli is reduced; decreased density appears to decrease inattention in these tasks.
To stimulate tactile awareness in clients with tactile extinction, Anderson and Choy79 suggest stimulating the affected arm as the individual watches. A rough cloth, vibrator, or the therapist’s or client’s hand may be used. Eventually, this activity may be done before activities that require spontaneous symmetrical scanning, such as dressing or walking through an obstacle course.
Problems of cortical blindness, color imperception, and visual agnosia
Identification of clinical problems
Cortical blindness.
Cortical blindness is considered a primary sensory disorder as opposed to a secondary associative disorder. It is discussed here, however, because of the many variations of this lesion that may result in problems with interpretation of visual stimuli. Cortical blindness, also known as central blindness, is a total or almost total loss of vision resulting from bilateral cerebral destruction of the visual projection cortex (area 17). Similar destruction limited to one hemisphere results in hemianopsia.55 The lesion may be ischemic, neoplastic, degenerative, or traumatic. The client may perceive the defect as a “blurring” of vision or as a marked decrease in visual acuity or may be unaware of the complete nature of the disability and even deny it, blaming the problem on eyeglasses that are too weak or a room that is too dark.
Color imperception.
Color perception may be impaired in the client with brain damage. This symptom is usually associated with right hemisphere or bilateral lesions.80 This deficit is different from color agnosia, in which there is a problem with naming colors correctly. Clients with defective color perception may see colors as “muddy” or “impure” in hue, or the color of a small target may fade into the background, decreasing the ability to differentiate it from the background.61,81 Total loss of color monochromatism is rare, but it can occur.
Visual agnosia.
A lesion circumscribed to the visual associative areas (areas 18 and 19) results in a number of unique visual disorders that are categorized as some form of visual agnosia. Lesions are usually bilateral with combined parietooccipital, occipitotemporal, and callosal lesions. Visual agnosia is defined as a failure to recognize visual stimuli (e.g., objects, faces, letters) although visual-sensory processing, language, and general intellectual functions are preserved at sufficiently high levels.82 It also has been described as perception without meaning; perception apparently occurs, but the percept seems “disconnected” from previously associated meaning. In this pure form, visual agnosia is a relatively rare syndrome, and there is controversy as to whether it is simply an extension of primary visual sensory deficits (variations of cortical blindness) or whether it should be considered as a separate neuropsychological entity.
Visual object agnosia.
During evaluation for the presence of visual object agnosia, the individual is presented with a number of common objects (e.g., key, comb, brush) and asked to name them. The evaluator may assume that the object is recognized if the client (1) names, describes, or demonstrates the use of the object or (2) selects it from among a group of objects as it is named by the examiner. If the person recognizes (describes or demonstrates) but is unable to name the object, failure is most likely a result of an anomia rather than an agnosic defect. Individuals with real visual agnosia have no concept of what the object is.82
Simultanagnosia.
Along the same vein are visual disorders that constrict or “narrow” the visual field during active perceptual analysis (i.e., when perceptions are tested separately, the visual field is within normal limits). Simultanagnosia is a disorder in which the person actually perceives only one element of an object or picture at a time and is unable to absorb the whole. As the individual concentrates on the visual environment, there is an extreme reduction of visual span. The problem is functionally similar to tubular vision. The narrowing of the functional perceptual field decreases the ability to simultaneously deal with two or more stimuli. It appears as if the person has bilateral visual inattention with macular sparing, although perimetric testing reveals full visual fields. A typical example is the individual whose visual attention is focused on the tip of a cigarette held between the lips and fails to perceive a match flame offered several inches away.83
Facial agnosia.
Another special type of agnosia that has been documented is failure to recognize familiar faces. The disorder is also known as prosopagnosia. The individual is able to recognize a face as a face but is unable to connect the face and differences in faces with people he or she knows. This person is unable to recognize family members, friends, and hospital staff by face. One must be careful not to confuse this with generalized dementia. There may be categorical recognition problems with items involving special visual experience, for example, recognition of cars, types of trees, or emblems. Facial agnosia is usually seen in combination with a number of other deficits, including spatial disorientation, defective color perception, loss of topographical memory, constructional apraxia, and a left upper quadrant visual field loss. These other symptoms are most likely not causative but rather a result of the similar neurological location of these functions.84
Color agnosia.
Finally, the individual may have difficulty recognizing names of colors, that is, an inability to name colors that are shown or to point to the color named by the examiner.85 This defect is considered agnosic (as opposed to a defect in color perception) because the client is able to recognize all colors in the Ishihara Color Plates86 and is also able to sort colors by hue. The determining factor here appears to be a problem with visual-verbal association. Color agnosia is most common in clients with left hemisphere lesions and is often accompanied by the syndrome of alexia without agraphia.82
Assessment
Cortical blindness and variations of it should be thoroughly assessed by the vision specialist. Assessment for agnosia must be preceded by a thorough assessment for visual acuity problems, visual field deficits, and unilateral visual inattention because these primary visual sensory and scanning deficits are often mistaken for agnosic performance. Next, basic color perception should be measured by use of the Ishihara Color Plates86 and color-sorting or color-matching tasks. Individuals with defective color perception will have difficulty with some visual-perceptual tasks because contextual cues related to color and shading are unavailable to them. Agnosia is a valid diagnosis only if (1) the aforementioned primary visual skills are intact and (2) language skills are intact (i.e., there should be no word-finding difficulty in spontaneous speech).
Color agnosia is evaluated by having the client name a color and point to colors named by the examiner. Facial agnosia is evaluated by presenting the individual with photographs of famous world figures, actors, politicians, and family members.61
Interventions
There are no reliable studies regarding treatment of cortical blindness, color imperception, or visual agnosia. Treatment principles presented here are based on the experience of Bouska and Biddle73 and Bouska and Kwatny.57 If cortical blindness or simultanagnosia is suspected, the therapist must first attempt to increase the client’s knowledge of foveal versus peripheral vision, that is, where the client is fixating. A small headlamp attached to the client’s forehead may be used under conditions of subdued lighting. The headlamp should not be used in a completely darkened room because the client needs to use normal spatial cues from the environment. The movement of the projected light in the environment and kinesthetic input from the neck receptors augment knowledge of where the eye is fixed. To carry out this task, the client must learn to position the eyes in midline of the head. The individual is asked to move the light (i.e., head and eyes) to locate and discriminate fairly large, bright stimuli placed on a plain background (e.g., yellow block on a brown table). As acuity and localization skills improve, stimuli and background should be made smaller and more complex (e.g., paper clip on a printed background or letters printed at different locations on a large page). The client should be encouraged to accurately point to or manipulate targets once located with the light or to keep the light on a target as he or she slowly moves the target with one hand. Thus the kinesthetic input from the limb can augment visual localization abilities.76 In patients with color imperception, treatment should initially involve materials and tasks with sharp color contrasts with minimal detail and should progress to less contrast (more hues) with more detail.
If the assessment has revealed a narrowing of the perceptual field, treatment should be aimed at progressively increasing the perception of large, bright, peripheral targets. For example, the client may be asked to fixate on a centrally placed target while another bright target is brought in slowly from or uncovered in the periphery.87,88 The individual is encouraged to maintain fixation on the central target while remaining alert for the presence of another target somewhere in the periphery. As the client improves, targets should be smaller, multiple, and exposed for briefer periods. Peripheral targets should always have bright surfaces that reflect light because the peripheral receptors in the retina are mainly rods (light as opposed to color receptors). Another powerful variation of this technique is to involve hand use in peripheral location of objects. For example, the patient senses the presence of a peripheral object then reaches with the hand to pinpoint location, then shifts the eyes to identify it. This can further help to differentiate between central and peripheral vision.
Color and facial agnosia may be approached by simply drilling the individual with regard to two or three names of colors or names of faces of people important to her or him. The client may be helped to pick out or memorize cues for associating names with faces.61
Problems of visual-spatial disorders
Identification of clinical problems
Individuals with brain lesions, particularly in the right posterior parietal and occipital areas, may have difficulties with tasks that require a normal concept of space.55 Disorders of this nature have been termed visual-spatial disorders, spatial disorientation, visual-spatial agnosia, spatial relations syndrome, and numerous other names. Visual-spatial abilities are complexly interwoven within the performance of many perceptual and cognitive activities such as dressing, building a design, reading, calculating, walking through an aisle, and playing tennis. An attempt is made here, however, to discuss spatial disorders in their purest form—that is, basic disorders—before dealing with visual-constructive disorders and disorders of analysis and synthesis. Constructional tasks require spatial planning, a type of planning that involves the building up and breaking down of objects in two and three dimensions. Constructional apraxia is viewed as a particular type of spatial-perceptual disorder and therefore is discussed separately under visual-constructive disorders and disorders of analysis and synthesis. Similarly, although perceptual skills such as figure-ground, form constancy, complex visual discrimination, and figure closure involve spatial concepts, tasks involving these skills often require the intellectual operations of synthesis and deduction. They, too, are discussed in the section dealing with analysis and synthesis.
All visual-spatial disabilities involve some problem with the apprehension of the spatial relationships between or within objects. Benton89 has categorized them as the following disabilities:
1. Inability to localize objects in space, to estimate their size, and to judge their distance from the observer. The client may be unable to accurately touch an object in space or indicate the position of the object (e.g., above, below, in front of, or behind). Relative localization may be impaired so that the individual may be unable to tell which object is closest. There may be difficulty determining which of two objects is larger or which line is longer. Holmes90 reported cases of gross disorder in spatial orientation revealed through walking; affected individuals, even after seeing objects correctly, ran into them. In another example a man intending to go toward his bed would invariably set out in the wrong direction. Difficulty in estimating distances may also extend to judgments of distances of perceived sounds and lead to overly slow and cautious gait or fear of venturing into public areas.
2. Impaired memory for the location of objects or places. An example is not being able to recall the position of a target previously viewed or the arrangement of furniture in a room. Individuals with this difficulty often lose things because they have no spatial memory to rely on for recall.
3. Inability to trace a path or follow a route from one place to another. Persons without this ability, known as topographical orientation, have difficulty understanding and remembering relationships of places to one another, so they may have difficulty finding their way in a space, as in locating the therapy clinic in a hospital or locating the housewares department in a store previously familiar to them. Normally functioning individuals often have mild signs of topographical disorientation. Everyone is familiar with the disoriented feeling of not knowing how to get out of a large department store or losing a sense of direction in a familiar city. Many of the topographical errors made by clients result from unilateral spatial inattention. For example, someone with left inattention may make only right turns. Topographical disorientation, however, may be seen in a person with no signs of unilateral inattention. This individual will demonstrate route-finding difficulties at certain points and will apparently randomly choose a direction.
4. Problems with reading and counting. These high-level tasks require directional control of eye movements and organized scanning abilities. Eye movements (saccades) during reading bring a new region of the text on the fovea, the part of the retina where visual acuity is the greatest and clear detail can be obtained from the stimulus. During reading, the line of print that falls on the retina may be divided into three regions: the foveal region, the parafoveal region, and the peripheral region. The foveal region subtends about 1 to 2 degrees of visual angle around the reader’s fixation point, the parafoveal region subtends about 10 degrees of visual angle around the reader’s fixation point, and the peripheral region includes everything on the page beyond the parafoveal region. Parafoveal and peripheral vision contribute spatial information that is used to guide the reader’s eye.91 Visual-spatial disorders appear to interfere to varying degrees with the spatial schema of a page of type or numbers and the dynamic organizational scanning that must take place to gather information appropriately. Clients with unilateral spatial inattention will miss words or numbers located on one half of the page. Other spatial problems unrelated to unilateral inattention include skipping individual words within a line or part of a line, skipping lines, repeating lines, “blocking” or having the inability to change direction of fixation, particularly at the end of a line, and generally losing the place on the total page. Performance usually deteriorates progressively as the individual continues to read. Eventually such persons cannot make sense of what they read, or if counting they complain of being lost or confused. This type of reading or counting disorder has nothing to do with recognition or interpretation of letters or numbers or their spatial configuration; rather, it represents a problem with dynamic sequential visual-spatial exploration during cognitive processing.
Other visual-spatial problems may include loss of depth perception, problems with body schema, and defective judgment of line orientation. There may also be difficulties with discrimination of right and left. Although unilateral spatial inattention is considered a visual-spatial disorder by many, it has been discussed separately in this chapter to increase clarity. Problems with judging line orientation (slant) or unilateral spatial inattention often interfere with a client’s spatial ability to tell time with a standard watch or clock. Perception of the vertical may also be considered a visual-spatial skill. Verticality perception is the interpretation of internal and external cues to maintain body balance. This maintenance is a complex neuromuscular process involving visual, proprioceptive, and vestibular systems. Clients with right lesions, particularly in the parieto-occipital region, have more difficulty perceiving verticality than those with left lesions. This may affect posture and ambulation.92
Assessment
The client should be asked to accurately touch a number of targets in all parts of the visual field while fixating on a central point. Mislocalization should be noted as well as the part of the visual field in which it occurred. Mislocalization within the central field is infrequent; however, defective localization of stimuli on one or both extramacular fields is more frequently seen.55 The client should be asked to determine which of a number of small cube blocks (placed perpendicularly in front of the client) is closest, which is farthest, and which is in the middle. Differences in binocular (stereoscopic) and monocular viewing should be measured in this and other tasks. Impairment in both of these types of depth perception and subsequent inaccuracy in judging distances have been described in individuals with brain injury.89
The influence of spatial dysfunction on reading and counting written material may be measured simply by asking the client to read a page of regular newsprint. The examiner should observe performance carefully and document type and frequency of errors. If errors occur, eye movements should be observed to gather additional information. Pages of scanning material (letters or numbers) often give additional information on spatial planning during reading. These are pages of print in which the size and density of the print are controlled. Scanning behavior may be demonstrated by asking the client to circle specific letters. Switching direction in the middle of a line, skipping letters or lines, perseveration, or any other abnormal performance behavior should be noted. Benton’s Judgment of Line Orientation Test93 may be used to document problems with directional orientation of lines. If there is no indication of apraxia, the client may simply be given a ruler and asked to match it to the directional orientation of the examiner’s ruler.
Interventions
Clients who do not know where they are in space need to internalize a spatial understanding before they can make judgments regarding the space around them. In gross motor spatial training, clients can be asked to roll and reach toward various targets. Supine, prone, sitting, and standing, with vision occluded, clients should try to localize tactile stimuli (various body locations touched by the therapist) and auditory stimuli (e.g., snapping fingers or ringing a bell) presented above, below, behind, in front of, and to the right and left of their bodies. The individual should state where the stimulus is and then point, roll, crawl, or walk toward it; this verbal, kinesthetic, and vestibular input augments spatial learning. In the occupational therapy kitchen, the client, once oriented to the room, may be asked to retrieve one type of object (e.g., cup) from “the top cupboard above your head,” from “the bottom cupboard below your waist,” from “the table behind you,” or from “the drawer on your right [or left].” These clients may also place objects in various positions within a room. They should then stand in the middle of the room, close their eyes, and from memory visualize, verbalize, and point to where the objects are in relation to themselves. Having localized them, the clients should then walk through the space and retrieve the objects in sequence. Functional carryover should always be emphasized, such as having individuals remember through visualization where they put their glasses in the living room before they begin searching. Visualization is defined as the internal “seeing” of something that is not present at that moment: a vision without a visual input or internal visual imagery.94 Visualization (spatial and other) is part of all perceptual tasks and may be used effectively as a treatment strategy. As previously discussed, a small feedback light placed in the middle of the client’s forehead can help teach spatial localization through eye-hand movements.
Organized visual-spatial exploration (eye movements) during reading or other scanning and cancellation tasks may be taught. Number and letter scanning sheets may be used for such training. Initially the size of numbers and the spaces between numbers should be large; this places less stress on visual acuity while training scanning. Before beginning, clients should orient themselves to the page spatially by numbering the right and left edges of each line. These numbers are used as additional spatial localization cues if needed during the scanning task.45 Clients should then be asked to circle a specific number (or numbers) whenever it occurs. To control erratic or impulsive eye movements, they should be instructed to use a pencil to underline each line and then loop the selected letter as it comes into view (see Figure 28-16). They may also be asked to read each letter. Underlining allows the kinesthetic and tactile receptors of the arm to control eye movements; verbalization allows the language and auditory systems to influence eye movements. Visual-spatial exploration exercises should progress to large-print magazines, books, or newspapers. The New York Times and Reader’s Digest are both available in large print. In all training activities it is most important that before the activity begins the clients fully comprehend the total space in which they will work. It is equally important that they reorient themselves at any point where errors occur. Those who lose their place during reading will eventually lose it again if the therapist simply points to where they should be. Chances are better that they will not lose their place again if they reorient themselves to the page spatially when an error occurs.
Problems of visual-constructive disorders
Identification of clinical problems
Clients with lesions in either the right or left hemisphere may have problems when trying to “construct.” Lesions in the parietal, temporal, occipital, and frontal lobes have been documented in individuals with visual-constructive disorders.55,95 The normal ability to construct, also known as visual-constructive ability and constructional praxis, involves any type of performance in which parts are put together to form a single entity. Examples include assembling blocks to form a design, assembling a puzzle, making a dress, setting a table, and simply drawing four lines to form a square (graphic skills). The skill implies a high level of dynamic, organized, visual-perceptual processing in which the spatial relations are perceived and sequenced well enough among and within the component parts to direct higher-level processing to sequence the perceptual-motor actions so that eventually parts are synthesized into a desired whole. Visual-constructive ability may be compromised if any part of this process is disturbed.
Typical tasks used to measure this ability include building in a vertical direction, building in a horizontal direction, three-dimensional block construction from a model or a picture of a model, and copying line drawings such as of houses, flowers, and geometric designs.84
Other clients, usually those with left lesions, have an “executional” or apraxic problem; they seem to have difficulty initiating and conducting the planned sequence of movements necessary to construct the whole. The problem seems to be in planning, arranging, building, or drawing rather than in spatial concepts. This deficit in its purest form is known as constructional apraxia. Constructional apraxia lies clinically outside the category of most other varieties of apraxia and is considered a special kind of “perceptual” apraxia. It occurs frequently in aphasic individuals; therefore the underlying mechanisms of aphasia and constructional apraxia may be related.96
Assessment
Constructional abilities are generally measured through tasks that require (1) copying line drawings of, for example, a house, clock face, flower, or geometric design (drawing may also be done without copying); (2) copying two-dimensional matchstick designs; (3) building block designs by copying or from a model; or (4) assembling puzzles. Table 28-2 lists common tests. The more complex the picture or design to be copied, the more complex are the constructional tasks. The following are examples of drawing and block construction deficits:
TABLE 28-2
Common Tests Used to Assess Visuoconstructive Skills
TEST | STANDARDIZATION |
Drawing pictures or shapes with or without an example to copy | Not standardized |
Reproducing matchstick designs | Not standardized |
Assembling puzzles | Not standardized |
Bender Visual Motor Gestalt Test | Standardized for children only |
Kohs Blocks Test | Standardized for adults |
WAIS Block Design Test | Standardized for adults |
Benton’s Three-Dimensional Constructional Praxis Test | Standardized for adults |
1. Clients may crowd the drawing or design on one side of the page or in one corner of the page or available space on the working surface, usually a result of the influence of unilateral spatial inattention.
2. Lines in drawings may be wavy or broken, too long or too short.
3. One line may not meet another accurately, or lines may transect one another; in block designs, parts may not be neatly placed but rather may have small gaps.
4. There may be “overdrawing” of angles or parts of the figure because of graphic perseveration (scribble), spatial indecision, or problems with executive planning.
5. Clients may superimpose their copy on the model or superimpose one of their drawings on top of another. In block design construction, they may become confused between the model and their reproduction and use part of the model to complete their design. This has been termed the “closing-in” phenomenon, a failure to distinguish between model and reproduction.55
6. Parts of the drawing or design may be reversed. Horizontal reversals are more common than vertical reversals.
Interventions
As previously mentioned, visual-constructive disorders are thought to result from different underlying problems in different individuals (e.g., visual-spatial disorders in persons with right hemisphere lesions and executive, planning, or synthetic disorders in those with left hemisphere lesions). There are few reliable studies on treatment strategies for visual-constructive disorders. One possible treatment strategy is known as saturational cuing.97 This method involves presenting controlled verbal instruction on task analysis and sequence and presenting cues on spatial boundaries (cuing is also response related).
Setting a table for one to five people
Assembling a piece of woodwork, a toy, a tool, a motor
Organizing a shelf in a library or a kitchen
Organizing a filing system or cabinet
Putting pieces of a sewing pattern together
Rearranging furniture according to a preset plan
Assembling a craft according to a preset plan
Problems of visual analysis and synthesis disorders
Identification of clinical problems
This separate discussion of visual analysis and synthesis is arbitrary. There is never any clear demarcation among the processes of visual-spatial orientation, visual construction, and visual analysis and synthesis. Analysis of likes and differences, relationships of parts to one another, and reasoning and deduction occur simultaneously with more basic spatial and constructive percepts. The final visual concept of a task (e.g., what a place setting on a table should look like) is necessary before the task is begun. Similarly, synthesis of one part of a task may be necessary before synthesis of the entire task can occur. For example, the person who is setting a table for four people must be able to conceptualize one place setting before conceptualizing the table with four place settings. Those points during perceptual processing when there is a colligation or blending of discrete impressions into a single perception are known as synthesis. This final stage of coordination and interpretation of sensory data is thought to be deficient in many individuals with perceptual problems. Deficits may be present with either left or right hemisphere damage but are more common and more severe with right lesions.61,98
Visual-perceptual skills considered to be analytical and synthetic in nature include making fine visual discriminations, particularly in complex configurations; separating figure from background in complex configurations (figure-ground); achieving recognition on the basis of incomplete information (figure closure); and synthesizing disparate elements into a meaningful entity, as, for example, conceptualizing parts of a task into a whole.5
Assessment
Many tests have been designed to measure the capacity for analysis and synthesis. Test items include complex figures in which small parts of a figure differ from another figure. The client is asked to select the one that is different. Studies have shown that basic discrimination of single attributes of a stimulus such as length, contour, or brightness is intact in many clients.99–101 The problem appears when these individuals are asked to discriminate between more complex configurations with subtle differences. Tests also measure figure-ground ability; the client must select the embedded figure from the background. Functional examples of this problem are the inability of a client to find her or his glasses if they are lying on a figured background, to find a white shirt on a white bedspread, and to find his or her wheelchair locks. Figure closure is measured by asking the client to complete an incomplete figure, such as part of the outline of a common shape. Finally, synthesis of parts into a whole, also known as visual organization, is measured by asking the client to conceptualize and organize the whole picture by, for example, looking at separate segments of the picture (e.g., cup or key) that have been divided and placed in unusual positions. This type of synthesis is necessary for high-level constructional tasks. Table 28-3 outlines examples of tests used to evaluate visual analysis and synthesis.
TABLE 28-3
Common Tests Used to Assess Visual Analysis and Synthesis
TEST | USE |
Hooper Visual Organization Test | Standardized for adults |
Motor-Free Visual Perception Test | Standardized for adults |
Raven’s Progressive Matrices | Standardized for adults |
Embedded Figure Test | Standardized for adults |
Southern California Figure-Ground Test | Standardized for children only |
Perceptual retraining with computers
During the past 15 years, numerous computer programs have been developed for rehabilitation of brain damage symptoms, including those affecting cognition (e.g., attention, sequencing, or memory) and perception. Because the computer is so highly visual, it becomes an obvious tool for treatment of visual-perceptual dysfunction. Treatment with computers has been named computer-assisted therapy. There is a growing interest in development of and research into programs for rehabilitation, for Alzheimer’s prevention, and in normal healthy aging adults. The largest treatment study of 487 subjects was able to demonstrate statistically significant improvements in memory and attention by using a plasticity-based adaptive training program.102 A number of other studies are in progress. Advantages of computer-assisted therapy include control and flexibility of perceptual variables during treatment (e.g., number, size, speed), immediate feedback regarding performance, automatic control for learning (e.g., items are repeated if incorrect to facilitate learning), and being motivational. Visual-perceptual training with computers, if used, should be viewed as one part of a patient’s treatment program. One should always remember that the computer, monitor, and keyboard are just that: they do not require the many perceptual, vestibular, and motor responses typical of daily performance (e.g., scanning requirements may be bilateral, but they are not global and associated with head movement). A patient’s total program may include computer-assisted therapy as an additional tool; however, it should never be substituted for more significant training within the multidimensional environment. Some computer programs for visual-perceptual training are listed in Box 28-6.
*Bernell/USO, 4016 North Home Street, Mishiwaka, IN 46545; (800) 348-2225.
†Vistech Consultants, 4162 Little York Road, Dayton, OH 45414-2566.
*Bernell/USO, 4016 North Home Street, Mishiwaka, IN 46545; (800) 348-2225. Complete Visual Screening Kit: Laurie R. Chaikin, OD, OTR/L, 420 F Cola Ballena, Alameda, CA 94501; Laurie.chaikin@gmail.com.
*Bernell/USO, 4016 North Home Street, Mishiwaka, IN 46545; (800) 348-2225. Complete Visual Screening Kit: Laurie R. Chaikin, OD, OTR/L, 420 F Cola Ballena, Alameda, CA 94501; Laurie.chaikin@gmail.com.
*College of Optometrists for Vision Development has a list of behavioral doctors: 234 North Lindberg Boulevard, Suite 310, St Louis, MO 63141; or Optometric Extension Program, 1921 East Carnegie Avenue, Suite 3L, Santa Ana, CA 92705-5510. Neuro-Optometer Rehabilitation Association, www.nora.cc/index.html.