Neonates and parents: neurodevelopmental perspectives in the neonatal intensive care unit and follow-up
JANE K. SWEENEY, PT, PhD, PCS, C/NDT, FAPTA, TERESA GUTIERREZ, PT, MS, PCS, C/NDT and JOANNA C. BEACHY, MD, PhD, FAAP
After reading this chapter the student or therapist will be able to:
1. Discuss three theoretical frameworks guiding neonatal therapy services in the neonatal intensive care unit.
2. Identify the physiological and structural vulnerabilities of preterm infants that predispose them to stress during neonatal therapy procedures.
3. Outline supervised clinical practicum components and pediatric clinical experiences to prepare for entry into neonatal intensive care unit practice.
4. Describe how the grief process may affect behavior and caregiving performance of parents of low–birth-weight neonates.
5. Differentiate the developmental course and neuromotor risk signs in infants with emerging neuromotor impairment from the clinical characteristics of infants with transient movement dysfunction.
6. Identify instruments for neuromotor examination of high-risk infants in neonatal intensive care units and in follow-up clinics and compare psychometric features of the tests.
7. Describe program plans and follow-up for low–birth-weight infants in neonatal intensive care unit and home settings.
Premature birth is associated with an increased prevalence of major and minor neurodevelopmental disability. Advancements in newborn resuscitation and neonatal intensive care have contributed to greatly improved survival of infants with low birth weight (LBW), but risk of neurodevelopmental sequelae remains high.1,2
Although brain injury can be documented by ultrasonography, computed tomography (CT), and magnetic resonance imaging (MRI) in infants, prediction of subsequent neurodevelopmental outcome is still relatively unreliable.3–6 Serial clinical examinations and careful monitoring of neurodevelopmental status are therefore critical during the neonatal period and discharge from the neonatal intensive care unit (NICU) through the outpatient phase of care. Pediatric therapists with mentored, subspecialty training in neonatology and infant therapy approaches can serve these increasing numbers of surviving neonates at neurodevelopmental risk by (1) providing valuable diagnostic data through neurological and developmental examination, (2) participating in developmental and environmental interventions adapted to each infant’s physiological, motor, and behavioral needs, (3) facilitating and coordinating interdisciplinary case management for infants and parents, and (4) reinforcing preventive aspects of health care through early intervention and long-term developmental monitoring.
Theoretical framework
Dynamic systems
Dynamic systems theory applied to infants in the NICU refers first to the presence of multiple interacting structural and physiological systems within the infant to produce functional behaviors and second to the dynamic interactions between the infant and the environment. In Figure 11-1, neonatal movement and postural control are targeted as a core focus in neonatal therapy, with overlapping and interacting influences from the cardiopulmonary,7 behavioral, neuromuscular, musculoskeletal, and integumentary systems. A change or intervention affecting one system may diminish or enhance stability in the other dynamic systems within the infant. Similarly, a change in the infant’s environment may impair or improve the infant’s functional performance.
This theory guides the neonatal practitioner to consider the many potential physiological and anatomical influences (dynamic systems within the infant) that make preterm infants vulnerable to stress during caregiving procedures, including neonatal therapy. In dynamic systems theory, emphasis is placed on the contributions of the interacting environments of the NICU, home, and community in constraining or facilitating the functional performance of the infant.8
Synactive model of infant behavior
The synactive model of infant behavioral organization is a specific neonatal dynamic systems model for establishing physiological stability as the foundation for organization of motor, behavioral state, and attention or interactive behaviors in infants. Als and colleagues9–11 described a “synactive” process of four subsystems interacting as the neonate responds to the stresses of the extrauterine environment. They theorized that the basic subsystem of physiological organization must first be stabilized for the other subsystems to emerge and allow the infant to maintain behavioral state control and then interact positively with the environment (Figure 11-2).
To evaluate infant behavior within the subsystems of function addressed in the synactive model, Als and colleagues10,11 developed the Assessment of Preterm Infants’ Behavior (APIB). With the development of this assessment instrument, a fifth subsystem of behavioral organization, self-regulation, was added to the synactive model. The self-regulation subsystem consists of physiological, motor, and behavioral state strategies used by the neonate to maintain balance within and between the subsystems. For example, many infants born preterm appear to regulate overstimulating environmental conditions with a behavioral state strategy of withdrawing into a drowsy or light sleep state, thereby shutting out sensory input. The withdrawal strategy is used more frequently than crying because it requires less energy and causes less physiological drain on immature, inefficient organ systems.
Fetters12 placed the synactive model within a dynamic systems framework to demonstrate the effect of a therapeutic intervention on an infant’s multiple subsystems (Figure 11-3). She explained that although a neonatal therapy intervention is offered to the infant at the level of the person, outcome is measured at the systems level, where many subsystems may be affected. For example, the motor outcome from neonatal therapy procedures is frequently influenced by “synaction,” or simultaneous effects, of an infant’s physiological stability and behavioral state. Physiological state and behavioral state are therefore probable confounding variables during research on motor behavior in neonatal subjects. Neonatal therapists may find this combined dynamic systems and synactive framework helpful in conceptualizing and assessing changes in infants’ multiple subsystems during and after therapy procedures.
Hope-empowerment model
a major component of the intervention process in neonatal therapy is the interpersonal helping relationship with the family. A hope-empowerment framework (Figure 11-4) may guide neonatal practitioners in building the therapeutic partnership with parents; facilitating adaptive coping; and empowering them to participate in caregiving, problem solving, and advocacy. The birth of an infant at risk for a disability, or the diagnosis of such a disability, may create both developmental and situational crises for the parents and the family system. The developmental crisis involves adapting to changing roles in the transition to parenthood and in expanding the family system. Although not occurring unexpectedly, this developmental transition for the parents brings lifestyle changes that may be stressful and cause conflict.13 Because parents are experiencing (mourning) loss of the “wished for” baby they have been visualizing in the past 6 months, they often struggle with developing a bond with their “real” baby in the NICU.14
A situational crisis occurs from unexpected external events presenting a sudden, overwhelming threat or loss for which previous coping strategies either are not applicable or are immobilized.15 The unfamiliar, high-technology, often chaotic NICU environment creates many situational stresses that challenge parenting efforts and destabilize the family system.16 The language of the nursery is unfamiliar and intimidating. The sight of fragile, sick infants surrounded by medical equipment and the sound of monitor alarms are frightening. The high frequency of seemingly uncomfortable, but required, medical procedures for the infant are of financial and humanistic concern to parents. No previous experiences in everyday life have prepared parents for this unnatural, emergency-oriented environment. This emotional trauma of unexpected financial and ongoing psychological stresses during parenting and caregiving efforts in the NICU contributes to potential posttraumatic stress disorder in parents of infants requiring intensive care.17,18
The quality and orientation of the helping relationship in neonatal therapy affect the coping style of parents as they try to adapt to developmental and situational crises (see Figure 11-4). Although parents and neonatal therapists enter the partnership with established interactive styles and varying life and professional experiences, the initial contacts during assessment and program planning set the stage for either a positive or a negative orientation to the relationship.
Despite many uncertainties about the clinical course, prognosis, and quality of social support, a positive orientation is activated by validation or acknowledgment of parents’ feelings and experiences. Validation then becomes a catalyst to a hope-empowerment process in which many crisis events, negative feelings, and insecurities are acknowledged in a positive, supportive, nonjudgmental context in which decision-making power is shared.19 In contrast, a negative orientation may be inadvertently facilitated by information overloading without exploration and validation of parents’ feelings, experiences, and learning styles. This may lead to magnified uncertainty, fear, and powerlessness with the misperception of excessive complexity in the proposed neuromotor intervention activities.
In a hope-empowerment framework, parent participation in neuromotor intervention allows sharing of power and responsibility and promotes continuous, mutual setting and revision of goals with reality grounding. Adaptive power can be generated by helping parents stabilize and focus energy and plans and by encouraging active participation in intervention and advocacy activities.19 Exploring external power sources (e.g., Parent to Parent USA or other parent-to-parent support groups20) early in the therapeutic relationship may help parents focus and mobilize.20–23
Hope and empowerment are interactive processes. They are influenced by existential philosophy: the hope to adapt to what is and the hope to later find peace of mind and meaning for the situation, regardless of the infant’s outcome. In describing the effect of a prematurely born infant on the parenting process, Mercer24 related that “hope seems to be a motivational, emotional component that gives parents energy to cope, to continue to work, and to strive for the best outcome for a child.” She viewed the destruction of hope as contributing to the physical and emotional withdrawal frequently observed in parents who attempt to protect themselves from additional pain and disappointment and then have difficulty reattaching to the infant.
Hope contributes to the resilience parents need to get through the arduous 1- to 4-month NICU hospitalization period and then begin to face the future in their home and community with an infant at neurodevelopmental risk. Groopman25 proposed that hope provides the courage to confront obstacles and the capacity to surmount them. He described the process of creating a middle ground where truth (of the circumstances) and hope reside together as one of the most important and complex aspects in the art of caregiving.
Neonatal complications associated with adverse outcomes
Improvements in neonatal intensive care over the last 30 years have led to the increased survival of preterm and term infants. Specific obstetric advances include establishment of specialized tertiary care centers, earlier identification of high-risk pregnancies, improvements in prenatal diagnosis, and medications used to stabilize maternal medical conditions and enhance fetal well-being. Respiratory compromise in preterm infants has significantly decreased as a result of (1) maternal betamethasone administration to promote fetal lung maturity; (2) availability of commercial surfactant to improve pulmonary function; and (3) advances in ventilator design and capability, enhancing management of respiratory distress with significantly diminished pulmonary dysfunction. In addition, improvements in continuous monitoring of vital signs, radiological imaging techniques, delivery of medications, and maintenance of thermal stability have aided earlier identification of neonatal problems and enhanced improvements in care. Increased survival is most evident in the extremely low–birth-weight (ELBW) infant, that is, birth weight less than 1000 g. For infants born at 23 weeks of gestation, survival has increased from approximately 0% to more than 50%, and for infants born at 26 weeks of gestation, survival has increased from 25% to 85%.26 It is important to note that the incidence of severe neurological injury has decreased over time in these extremely preterm infants. However, a significant number of preterm infants will exhibit long-term neurological impairment owing to increased survival.
The long-term effect of a neurological insult on the developing brain depends on the timing of the injury, the gestational age of the infant, and the nature and duration of the insult.27 During the first month of gestation, the neural tube is formed. Neurological insult at this time leads to abnormal neural tube development, specifically anencephaly, encephalocele, or myelomeningocele. Neuronal proliferation is nearly complete by 5 months of gestation. All neurons and glial cells originate in the ventricular and subventricular zone (germinal matrix). Disorders of proliferation result in microcephaly, with either decreased size or decreased number of proliferating neuronal units or macrocephaly. Neuronal migration occurs at 3 to 6 months of gestation, and neurons are guided by glial cells to form neuronal columns. Subplate neurons, essential for correct organization of the brain, are formed at this time. Insults during this period of development result in marked disturbance of neurological structure and function with aberrations noted in gyral formation (lissencephaly, schizencephaly, and polymicrogyria) and/or absence of the corpus callosum.
The final phase of brain organization is glial maturation to astrocytes and oligodendrocytes. Astrocytes help maintain the blood-brain barrier, provide nutrient support, regulate neurotransmitter and potassium concentration, and assist in neuronal repair after injury. Oligodendrocytes produce myelin, a protective fatty sheath that surrounds axons (white matter) and facilitates nerve transmission. Myelination starts in midgestation and continues through adulthood. Oligodendrocytes are especially sensitive to hypoxia and other insults. Disruption of normal myelination results in white matter hypoplasia and periventricular leukomalacia (PVL) (see later discussion) leading to impaired motor function.27
The most common neonatal problems associated with impaired neurological functioning and long-term developmental delay are listed in Table 11-1. In addition to descriptions of neonatal neurological conditions, a discussion is provided on the impact on neonatal development of maternal medication, such as drugs of abuse and psychotropic medications. The importance of developmental follow-up for healthy “late” preterm infants born at 34 to 366/7 weeks of gestation is also discussed in this section.
TABLE 11-1
NEONATAL COMPLICATIONS AFFECTING BRAIN DEVELOPMENT
AGE | SPECIFIC INSULT |
Preterm | Intraventricular hemorrhage (IVH)Periventricular hemorrhagic infarct (PVHI) Posthemorrhagic ventricular dilatation (PHVD) Periventricular leukomalacia (PVL) Necrotizing enterocolitis (NEC) |
Term | Hypoxic-ischemic encephalopathy (HIE) |
Intraventricular hemorrhage
IVH originates in the microcirculation or capillary network of the germinal matrix.28 The germinal matrix is located adjacent to the ventricle and is a well vascularized area owing to the high metabolic demand from the rapidly proliferating neuronal stem cells. Vessels in the germinal matrix are thin walled and fragile, which predisposes them to rupture. In addition, preterm infants have impaired autoregulation—that is, the inability to maintain cerebral blood flow across a large range of blood pressures. Thus during labor, delivery, and the immediate postpartum transition period, changes in blood pressure can lead to cerebral hypoperfusion and ischemia as well as to hyperperfusion and vessel rupture. Alterations in CO2 lead to either reduced cerebral blood flow from hypocarbia or increased flow from hypercarbia. Other risk factors for IVH include asphyxia, fluid bolus infusion (especially of hypertonic solutions), anemia, and pain.29 Platelet and coagulation disturbances have been implicated as risk factors for the development of IVH. IVH is rarely seen in infants with gestational age greater than 32 weeks owing to the developmental involution of vessels in the germinal matrix.
Diagnosed by cranial ultrasound, IVH is graded in severity from 1 to 4,28 with grade 1 IVH being the most mild because the hemorrhage is confined to the germinal matrix. In grade 2 IVH, the hemorrhage extends into the ventricle (Figure 11-5). Grade 3 IVH occurs when the hemorrhage fills more than 50% of the ventricle and causes ventricular distention. Grade 4 IVH, or periventricular hemorrhagic infarct (PVHI), is a complication of IVH caused by venous congestion of the terminal veins that border the lateral ventricles leading to white matter necrosis (see later).30 It is important to note that IVH may not be apparent on cranial ultrasound in the first few days after birth. However, 90% of IVHs can be detected by day 4. In addition, the full extent of the hemorrhage may not be appreciated for several days after the initial diagnosis of IVH is made.28 The evolution of grades 3 and 4 IVH over 10 days is shown in Figure 11-6, A and B.
Many researchers have investigated the relationship of IVH grades with severity of neurodevelopment delay. In general, grades 1 and 2 IVH are not associated with a significant increase in developmental abnormalities but do not ensure normalcy. Infants with severe IVH (grade 3 and/or 4) have increased mortality and are at markedly increased risk for developmental disabilities, specifically spastic hemiplegia or diplegia affecting the lower extremities. As can be seen in Figure 11-7, motor tracts innervating the lower extremities are in close proximity to the area of the germinal matrix and the site of the origin of IVH leading to lower-extremity spastic cerebral palsy (CP). However, abnormalities visible on cranial ultrasound are not able to absolutely predict long-term outcome because the amount of cortex damaged and the neuronal tracts affected by IVH cannot be identified by ultrasound. In addition, ultrasound may not be sensitive enough to identify PVL (see later).
Periventricular hemorrhagic infarct
Grade 4 IVH was originally thought to be an extension of IVH into the parenchyma but is actually a known complication of IVH.30 PVHI is caused by venous compression of the terminal veins that border the lateral ventricles leading to impaired venous drainage and congestion and eventually hemorrhagic infarction. The usual initial distribution of PVHI seen on cranial ultrasound is fan-shaped echodensities in the periventricular location (see Figure 11-6, A2-4). Over time there is destruction of preoligodendrocytes and motor axons leading to white matter necrosis and the development of porencephalic cyst. PVHI is usually unilateral (approximately 70%), and approximately three quarters of cases are associated with severe IVH [grade 3 and 4]. Infants with small, unilateral PVHI have no increased risk of developmental delays compared with infants with grade 3 IVH. However, if the PVHI is bilateral or if multiple porencephalic cysts are present, the risk of severe motor impairment and CP is significantly increased. In addition, approximately 50% of infants with PVHI have visual field defects, probably secondary to damage to the axons of nerves carrying information to the visual cortex.30
Posthemorrhagic ventricular dilatation
Approximately 50% of infants with severe IVH will develop posthemorrhagic ventricular dilatation (PHVD) from either blockage of the normal flow of cerebrospinal fluid (CSF) or decreased absorption of CSF. Approximately 50% to 75% of these infants will develop progressive PHVD, resulting in need for treatment. The severity of ventricular dilatation can be measured via serial cranial ultrasound examinations.31,32 Severe ventricular dilatation is usually evident by 2 to 3 weeks after birth. Rapid increase in head circumference does not occur until approximately 4 weeks after birth.33 Posthemorrhagic ventricular dilatation is treated by serial removal of CSF by spinal tap, subgaleal shunt, or placement of an Ommaya reservoir. Removal of CSF has been shown to decrease intracranial pressure and improve cerebral perfusion34 and also to increase cortical gray and white matter.35 In addition, there is indirect evidence that ventricular distention itself may cause secondary brain injury through stretching and disruption of axons, gliosis, and loss of oligodendrocytes.
No consensus has been reached on the optimal management of PHVD. Ventriculoperitoneal (VP) shunt placement is necessary in infants with PHVD that does not resolve with serial removal of CSF. Significant complications of VP shunt include sepsis, specifically ventriculitis, or shunt malfunction such as blockage or failure. These complications necessitate shunt revisions, which further compromise these fragile infants. Researchers from a consortium of 17 tertiary NICUs recently published results on the neurodevelopmental outcome at 2 years of age of ELBW infants with grade 3 and 4 IVH who were born from 1993 to 2002.36 Infants who required VP shunt placement had significantly worse outcomes than infants with grade 3 or 4 IVH alone (Table 11-2). Moreover, the number of infants who were untestable (MDI or PDI = 49) because of severe neurodevelopmental handicap was significantly increased in the group of infants who received a VP shunt. In addition, CP was significantly more prevalent in infants who had a VP shunt placed than in infants with only grade 3 or 4 IVH.
TABLE 11-2
NO VP SHUNT | VP SHUNT | P | |
MDI <70 | 326/719 (45.3%) | 146/214 (68.2%) | <.0001 |
MDI = 49 | 130/719 (18.1%) | 87/214 (40.7%) | <.0001 |
PDI <70 | 263/711 (37.0%) | 163/214 (76.2%) | <.0001 |
PDI = 49 | 149/711 (21.0%) | 113/214 (52.8%) | <.0001 |
CP | 217/767 (28.3%) | 158/227 (69.6%) | <.0001 |
CP, Cerebral palsy; MDI, Mental Developmental Index; PDI, Psychomotor Development Index.
Modified from Adams-Chapman I, Hansen NI, Stoll BJ, et al: Neurodevelopmental outcome of extremely low birth weight infants with posthemorrhagic hydrocephalus requiring shunt insertion. Pediatrics 121:1167-1177, 2008.
Recent retrospective studies from the Netherlands indicated that earlier intervention when the ventricles are moderately dilated significantly decreased the need for VP shunt from 62% to 16% and trended to improve long-term developmental outcome with a decreased incidence of moderate to severe handicap.32,37 Thus, halting the progression of PHVD and decreasing the need for VP shunt is likely to improve long-term outcome in these infants. However, because PVHD can spontaneously resolve without intervention, identification of factors that can accurately predict which infant will develop persistent PHVD and consequently require VP shunt placement is needed.
Periventricular leukomalacia
PVL can be either cystic (see Figure 11-6, C) or global and may be difficult to identify on radiological images. Cystic PVL results from the focal dissolution of cellular tissue approximately 3 weeks after the insult and can be identified on ultrasound if greater than 0.5 cm in diameter.27 However, cysts visualized by cranial ultrasound may disappear over time owing to fibrosis and gliosis. Thus the incidence of cystic PVL is felt to be underestimated by cranial ultrasound examination. Global PVL results from diffuse white matter injury and myelin loss. This finding can be subtle with moderate ventricular dilatation and/or a mild increase in extraaxial fluid on cranial imaging. Infants with severe PVL have marked ventricular dilatation, increased extraaxial fluid, and decreased head growth.
MRI obtained at term is more sensitive in identifying white matter injury than cranial ultrasound and is predictive of subsequent neurosensory impairment and cognitive delay present in up to 50% of extremely preterm infants.38 Newer techniques, such as diffusion tensor imaging (DTI), functional connectivity MRI (fcMRI), and morphometry for analysis of cortical folding are being investigated as early markers of impaired neurodevelopmental outcome. Diffusion tension imaging measures the restriction of water diffusion in the myelin sheath surrounding axons and yields information at the microstructure level about axon caliber changes and aberrations in myelination. In addition, DTI allows for visualization of brain fiber tracks and neuronal connectivity. In research, fMRI is used to investigate interaction between areas of the brain at rest and during tasks by analyzing changes in blood flow. Morphometic analysis of sequential MRI scans has been used to create maps of cortical folding with quantification of surface area and degree of gyral formation. White matter injury results in delayed myelination and altered cortical folding.38 Both fMRI and morphometric analysis of cortical folding are currently available only in research studies, not in clinical management.
Necrotizing enterocolitis
Complications of NEC include sepsis, wound infection, and stricture formation (10% to 35%) requiring repeated surgery. Growth of infants with NEC can be impaired due to feeding intolerance, prolonged total parenteral nutrition (TPN), removal of significant amounts of intestine, and repeated surgeries and infections. Persistence of weight at less than 10% for age is correlated with poor neuromotor and neurodevelopmental outcome.39 Failure to achieve normalization of head growth is associated with abnormal performance at 1 year and probably reflects significant white matter injury. Infants with surgically managed NEC have been shown to have significantly increased incidence of CP (24% versus 15%), deafness (4.1% versus 1.5%), and blindness (4.1% versus 1%).39 Meta-analysis of seven studies investigating the impact of NEC on neurodevelopmental outcome showed that infants with surgically treated NEC have a statistically significant increase in cognitive, psychomotor, and neurodevelopmental impairment compared with age-matched preterm infants without NEC.40 Impaired neurodevelopmental outcome in infants with NEC is further exacerbated by associated sepsis and the release of inflammatory cytokines and mediators in addition to hypoxia, all of which contribute to further insult to preoligodendrocytes, leading to white matter injury.
Cerebellar injury
The cerebellum is essential for gross and fine motor control, coordination, and motor sequencing and plays an important role in attention and language.36 The clinical hallmark of damage to the cerebellum is ataxia. However, recent advances in functional MRI (fMRI) have demonstrated that there are interactions between the cerebellum and nonmotor areas of the brain involved in language, attention, and mental imagery. Cerebellar injury can also be noted early in neonatal development from cranial ultrasound of the posterior fossa (mastoid view). The incidence of cerebellar injury may be as high as 20% in ELBW infants.41 Although the mechanism for damage is unknown, IVH is present in more than 75% of infants with cerebellar injury, implying similar risk factors for both IVH and cerebellar hemorrhage or the possibility that IVH leads to cerebellar hemorrhage. The majority of cerebellar lesions (70%) are unilateral.
Preterm infants with isolated cerebellar hemorrhage exhibit significant neurological impairments: hypotonia (100%), abnormal gait (40%), ophthalmological abnormalities (approximately 40%), and microcephaly (17%).42 Overall, preterm infants with cerebellar hemorrhage performed significantly lower on tests of gross and fine motor skills and have deficits in vision and expressive and receptive language. Infants with both cerebellar injury and IVH have greater motor impairment than infants with isolated cerebellar hemorrhage. Socially, infants with isolated cerebellar hemorrhage exhibit delayed communication skills, decreased social skills with more withdrawn behavior, and impaired ability to attend to tasks. Thus, cerebellar injury increases the risk for poor neurodevelopmental outcome in cognition, learning, and behavior in preterm infants.42 For long-term effects of cerebellar damage, refer to Chapter 21.
Hypoxic-ischemic encephalopathy
Perinatal asphyxia, the result of a hypoxic-ischemic (HI) insult, affects three to five per 1000 live births and leads to hypoxic-ischemic encephalopathy (HIE) in 0.5 to one per 1000 live births. Impaired oxygen delivery to the fetus can result from maternal hypotension, placental abruption, placental insufficiency, cord prolapse, prolonged labor, and/or traumatic delivery. Approximately 15% to 20% of infants with HIE will die, and 25% of the surviving infants will exhibit permanent neurological sequelae. Clinical findings will vary depending on the timing and duration of the HI insult, preconditioning and fetal adaptive mechanisms, comorbidities, and resuscitative efforts. Infants who are intrauterine growth restricted are at increased risk of an HI insult due to decreased nutrient reserves.43
It is important to note that the injury from an HI insult is an evolving and progressive process that begins at the time of the insult and continues through the recovery period (Figure 11-8). The HI insult causes decreased oxygen and glucose delivery to the brain, causing a shift from aerobic to anaerobic metabolism. This causes a decrease in adenosine triphosphate (ATP) production, leading to failure of the membrane-bound Na+-K+-ATPase pump. Sodium enters the neuronal cell, causing depolarization and release of excitatory neurotransmitters, specifically glutamate. This initial phase can last several hours and is marked by significant acidosis, depletion of high-energy compounds (energy failure), cellular swelling caused by entry of sodium and water, and cellular necrosis, causing spillage of intracellular contents into the extracellular space. The degree of neuronal necrosis is directly related to the duration and severity of the HI insult. During the subsequent reperfusion phase, free radical production increases and activation of microglia from extruded intracellular contents occurs, causing release of inflammatory mediators. A second phase of energy failure ensues, but without acidosis. Calcium enters the cell and the mitochondria, which then turns on the apoptotic pathway (programmed cell death). During this second phase of energy failure, seizures are often present. Activation of the apoptotic pathway accounts for the majority of cellular death and is the target for treatment.27,28 The specific timing of the initiation of the reperfusion phase and the second phase of energy failure is unclear in the clinical setting because the actual timing of the HI insult is not well defined. In animal studies, the latency between the first and second phases of energy failure is several hours.
In term infants with HIE the cerebral damage is located in the deep structures of the brain (basal ganglia, thalamus, and posterior limb of the internal capsule) as well as the subcortical and parasagittal white matter.44 Diffusion-weighted MRI (DWI) is a very early diagnostic and sensitive technique to identify damage after the HI insult. As shown in Figure 11-8, a marked increase in signal in the subcortical and parasagittal white matter occurs as well as in the deep nuclear structures on DWI. MRI spectroscopy, localized to the basal ganglia or subcortical area, yields information about degree of secondary energy failure by analyzing for the depletion of the high-energy compound N-acetylaspartate and the presence of lactate.44,45 The degree of secondary energy failure as noted on MRI spectroscopy is predictive of death and poor neurodevelopmental outcome at 1 and 4 years of age.
Classification of the clinical signs associated with HIE is shown in Table 11-3.46 Infants with grade 1 HIE rarely have long-term sequelae. Infants with grade 2 or moderate HIE have abnormal tone and reflexes and decreased spontaneous activity, with seizures commonly present. Approximately 10% of infants with moderate HIE will die and up to 30% will have neurodevelopmental delay. Infants with severe HIE (grade 3) exhibit minimal or no spontaneous activity or reflexes. Clinically evident seizures are seldom present, but electrographically evident seizures are more common. Approximately 50% of these infants die, and of the survivors, more than 60% to 80% are profoundly impaired. Long-term consequences of HIE include bulbar palsies with difficulties in sucking, swallowing, and facial movement. These infants have difficulty with secretions and may require tube feeding owing to inability to protect the airway. Upper-extremity involvement is more prominent than lower-extremity deficits because the damage to the cerebral cortex is located in the parasagittal region (see Figure 11-7). The development of epilepsy occurs in about 30% of infants with HIE. Mental retardation and difficulties at school age occur frequently.
TABLE 11-3
SARNET SCORING SCALE FOR ENCEPHALOPATHY
STAGE 1 (MILD) | STAGE 2 (MODERATE) | STAGE 3 (SEVERE) | |
Level of consciousness | Hyperalert | Lethargy, obtunded | Stuporous |
Neuromuscular signs | |||
Muscle tone | Normal | Mild hypotonia | Flaccid |
Posture | Mild distal flexion | Distal flexion | Decerebrate |
Movement | Spontaneous | Decreased to little | Noxious stimuli |
Stretch reflexes | Overactive | Overactive | Decreased or absent |
Primitive reflexes | |||
Suck | Weak | Weak or absent | Absent gag |
Moro | Strong | Weak or incomplete | Absent |
Autonomic function | SympatheticDilated pupils Tachycardia |
ParasympatheticConstricted pupils Bradycardia Copious secretions Periodic breathing |
DepressedPupils nonreactive Variable heart rate Apnea Loss of temp regulation |
Seizures | None | CommonFocal or multifocal | Uncommon |
Modified from Sarnat HB, Sarnat MS: Neonatal encephalopathy following fetal distress. A clinical and electroencephalographic study. Arch Neurol 33:696-705, 1976.
Mild hypothermia (33.5° C) from application of cooling blankets or caps is becoming the standard of care for infants ≥ 36 weeks’ gestation who have an acute asphyxial event and moderate or severe HIE.47 Hypothermia has been shown to decrease cerebral metabolic demand and thus help preserve high-energy compounds. Hypothermia also delays membrane depolarization and decreases neuronal excitotoxicity. Free radical production and microglial activation are decreased. Most important, the activation of the apoptotic pathway is diminished. Transient side effects of hypothermia, such as bradycardia, mild hypotension, thrombocytopenia, and persistent pulmonary hypertension, can be medically treated and are usually not significant.48,49 A meta-analysis of published randomized studies comparing infants with moderate and severe HIE treated with either hypothermia or normothermia shows that hypothermic treatment significantly decreases mortality and morbidity (Table 11-4).50 Hypothermia appears to more efficacious in ameliorating brain damage in infants with mild HIE than in infants with severe HIE. It is known that hypothermia is most effective when administered before the onset of the second phase of energy failure. Because the HI insult can occur before delivery, it is postulated that hypothermia should be initiated as quickly as possible after delivery to increase the likelihood that it will diminish the neuronal damage and improve neurodevelopmental outcome.
TABLE 11-4
EFFECT OF MODERATE HYPOTHERMIA ON NEUROLOGICAL OUTCOMES AT 18 MONTHS COMPARED WITH CONTROLS
RISK RATIO (95% CONFIDENCE INTERVAL [CI]) | RISK DIFFERENCE (95% CI) | NUMBER NEEDED TO TREAT (95% CI) | P VALUE | |
Death or severe disability* | 0.81 (0.71-0.93) | −0.11 (−0.18-−0.04) | 9 (5-25) | .002 |
Survival with normal outcome† | 1.53 (1.22-1.93) | 0.12 (0.06-0.18) | 8 (5-17) | <.001 |
Mortality | 0.78 (0.66-0.93) | −0.07 (−0.12-−0.02) | 14 (8-47) | .005 |
Severe disability in survivors* | 0.71 (0.56-0.91) | − 0.11 (−0.20-−0.03) | 9 (5-30) | .006 |
Cerebral palsy in survivors | 0.69 (0.54-0.89) | −0.12 (−0.20-−0.04) | 8 (5-24) | .004 |
Severe neuromotor delay in survivors‡ | 0.73 (0.56-0.95) | −0.10 (−0.18-−0.02) | 10 (6-71) | .02 |
Severe neurodevelopmental delay in survivors§ | 0.71 (0.54-0.92) | −0.11 (−0.19-−0.03) | 9 (5-39) | .01 |
Blindness in survivors | 0.57 (0.33-0.96) | −0.06 (−0.11-0.00) | 17 (9-232) | .03 |
Deafness in survivors | 0.76 (0.36-1.62) | −0.01 (−0.05-0.03) | NA | .47 |
*Severe disability was defined in the CoolCap and TOBY trials as the presence of at least one of the following impairments: Mental Development Index score of less than 70 (2 standard deviations below the standardized mean of 100) on the Bayley Scales of Infant Development; gross motor function classification system level 3 to 5 (where the scale is from 1 to 5, with 1 being the mildest impairment); or bilateral cortical visual impairment with no useful vision. The NICHD trial defined disability as a Mental Developmental Index score of 70 to 84 plus one or more of the following impairments: gross motor function classification system level 2; hearing impairment with no amplification; or a persistent seizure disorder.
†Survival with normal outcome was defined as survival without cerebral palsy and with a Mental Developmental Index score of more than 84, a Psychomotor Developmental Index score of more than 84, and normal vision and hearing.
‡Severe neuromotor delay was determined on the basis of a Psychomotor Developmental Index score of less than 70 in survivors.
§Severe neurodevelopmental delay was determined on the basis of a Mental Developmental Index score of less than 70 in survivors.
From Edwards AD, Brocklehurst P, Gunn AJ, et al: Neurological outcomes at 18 months of age after moderate hypothermia for perinatal hypoxic ischaemic encephalopathy: synthesis and meta-analysis of trial data. BMJ 340:c363, 2010.
Maternal medication
The impact of maternal medications on the developing fetal brain depends on the specific drug as well as on the timing and duration of the drug exposure. Whereas the insults discussed previously cause predominantly cellular necrosis and apoptosis, medications given to the fetus and preterm infant cause alterations in the structure and function of genetic material as well as activation of the apoptotic pathway. The hypothesis that factors acting early in life have a long-lasting impact on development is called the Barker hypothesis or the fetal origins of adult disease. It is proposed that the biological value of this reprogramming is to prepare the fetus for maximal adaptation through methylation and deacetylation of histones, thereby determining the quantity of specific proteins that are produced. This topic is too extensive to be covered here and has been previously reviewed.51,52 This section will focus on heroin, methadone, cocaine, methamphetamine, and selective serotonin reuptake inhibitors (SSRIs) used in the treatment of maternal depression.
Cocaine
It is difficult to ascertain the exact frequency of cocaine use during pregnancy, but reports indicate that 1% to 45% of females have used cocaine during their pregnancy.28 Cocaine is extracted from the leaves of the coca plant and can be smoked, inhaled, or injected into the bloodstream. Cocaine induces an intense and immediate euphoric state and can be very addictive. Unlike with opioids, physical dependence does not occur, but severe and intense cravings last for several months and can recur for years after cessation of cocaine use.
Cocaine negatively affects neuronal proliferation, migration, growth, and connectivity, which distorts neuronal cortical architecture. However, the effects of intrauterine exposure to cocaine are difficult to determine because cocaine use is frequently associated with abuse of other illicit drugs, cigarettes, and alcohol. Other confounding variables include poor nutrition and limited prenatal care. In a large prospective blinded study, more infants exposed to cocaine in utero were delivered prematurely and exhibited decreased weight, length, and head circumference compared with matched controls.53 However, cocaine exposure did not affect the incidence of congenital abnormalities.
The vasoconstrictive properties of cocaine increase the risk for HI injury and middle cerebral artery stroke. Neonates with prenatal cocaine exposure demonstrate tremors, hypertonia, irritability, and poor feeding ability. Cocaine-exposed infants have abnormal sleep patterns and are at a threefold to sevenfold increased risk of sudden infant death syndrome (SIDS). No difference was found in developmental testing54 between cocaine-exposed infants and matched controls, but the tests did not effectively evaluate arousal, emotional control, and social interaction.55 In utero cocaine exposure has been linked to increased incidence of behavioral problems and special education referrals in school-aged children. On fMRI, differences in the right frontal cortex and caudate nucleus are evident and indicate abnormalities in regulation of attention and cognitive abilities referred to as executive function.
Opioids
Opioid use during pregnancy has been associated with tubal pregnancies, premature rupture of membranes, uterine irritability, preterm labor, and preeclampsia. Infants exposed to opioids in utero are intrauterine growth retarded at birth, but methadone has a less severe impact on fetal growth. Infants exposed to opioids in utero are noted to have a decreased incidence of respiratory distress related to enhanced surfactant production56 and decreased hyperbilirubinemia as a result of induction of the enzyme glucuronyl transferase used in the metabolism of bilirubin.
Withdrawal from narcotics occurs 2 to 3 days after delivery, but signs can be evident as long as 2 weeks after delivery. Methadone withdrawal usually occurs later than withdrawal from morphine or heroin related to its long half-life. Infants in withdrawal (neonatal abstinence syndrome [NAS]) exhibit gastrointestinal symptoms of vomiting and watery stools; neurological signs such as tremors; hypertonicity; high-pitched and incessant cry; hyperalert state; and sweating and fever. Infants with NAS have decreased ability to nipple feed despite excessive sucking on a pacifier. Seizures can be present in 2% to 11% of infants with NAS. Two commonly used scoring methods for severity of NAS are the Lipsitz57 and the Finnegan58 scales. The Lipsitz scale has 11 components that are scored from 0 to 3, with any score over 4 necessitating treatment.57 The Finnegan scale is a more comprehensive assessment, with more than 30 elements, and treatment is recommended if the score is greater than 8.58 A quiet, dimly lighted environment, decreased auditory stimulation, and swaddling or holding have been used to decrease neonatal irritability and pharmacotherapy. About 30% to 80% of in utero opioid-exposed infants will require medical treatment for NAS with morphine and either clonidine or phenobarbital. The goal of treatment is to decrease irritability, improve nippling efforts, and decrease vomiting and diarrhea.
Infants exposed to opioids in utero continue to demonstrate tremulousness, hypertonicity, irritability, and increased crying episodes. In addition, they are less able to interact with people, demonstrate decreased age-appropriate free play, and have delayed fine motor coordination. The incidence of apnea and SIDS is increased in opioid-exposed infants. An appropriate and nurturing home environment is essential after discharge from the hospital to maximize neurodevelopmental outcome.59,60
Selective serotonin reuptake inhibitors
SSRI medications such as fluoxetine (Prozac, Fontex, Seromex, Seronil), sertraline (Zoloft, Lustral, Serlain, Asenta), paroxetine (Paxil, Seroxat, Sereupin, Paroxat), fluvoxamine (Luvox, Favoxil), escitalopram (Lexapro, Cipralex, Esertia), and citalopram (Celexa, Seropram, Citox, Cital) are commonly prescribed to treat depression and anxiety disorders. The SSRI drugs inhibit serotonin reuptake, potentiating serotonergic neurotransmitter signaling. At least 600,000 infants are born yearly to mothers who have a major depressive disorder during their pregnancy.61 Medical therapy is the most common form of treatment for depression during pregnancy. Approximately 6% of pregnant woman use SSRIs during pregnancy, and almost 40% of depressed women have been reported to use antidepressants at some time during pregnancy.62 The serotonergic system is present early in gestation and is important in brain development. Perturbations in this system are associated with alterations in somatosensory processing and emotional responses.
The SSRI medications readily cross the placenta and are linked to an increased risk of spontaneous abortion but not an increased incidence of malformations.61 A recently published meta-analysis found that maternal depression was significantly associated with an increased incidence of preterm labor and neonatal birth weight of less than 2500 g but not intrauterine growth retardation of the fetus.63 Unfortunately, this study was unable to evaluate the effect of SSRI therapy on these outcomes. Infants exposed to SSRIs in the third trimester have symptoms similar to withdrawal from opioid exposure (irritability, tremors, jitteriness, agitation, and difficulty sleeping). Neonatal feeding difficulties are common, and seizures and abnormal posturing are occasionally present. These symptoms are transient, appearing 2 to 4 days after birth and disappearing by the second week of life.64 It is difficult to identify any specific adverse neurodevelopmental outcomes in infants exposed prenatally to SSRIs from published studies because of the variability in the specific SSRI taken, the duration and timing of SSRI use, and the confounding factors of maternal depression and the use of multiple medications.65
Late preterm birth
Preterm births have increased over the last 10 years and now constitute about 13% of all births. Late preterm infants—that is, infants born at 34 to 366/7 weeks’ gestation—make up approximately 70% of preterm births.66 Many factors are implicated in the early delivery of late preterm infants, including preterm labor, preeclampsia, premature rupture of membranes, sepsis, and multiple gestation pregnancies. The late preterm infant is at increased risk of respiratory distress from insufficient surfactant production, transient tachypnea of the newborn from decreased pulmonary water absorption, persistent pulmonary hypertension, and complications of mechanical ventilation (pneumothorax). Hospital stay is prolonged in the late preterm infant compared with the infant born at term gestation owing to the increased difficulty with oral feeding, need for phototherapy for hyperbilirubinemia, and continuation of antibiotic therapy for suspected sepsis.
Recent evidence has supported the concept that even healthy late preterm infants are at higher risk for neurodevelopmental delay compared with infants at term gestation. The late preterm infant’s brain is vulnerable to injury because a significant portion of brain development and maturation occurs during the last 2 months of pregnancy.67 Recent studies have shown that late preterm infants tested lower in reading skills in kindergarten and first grade, but not in math skills, than infants born at term gestation.68 However, kindergarten and first-grade teachers rated late preterm infants as not as competent as term infants in math and reading ability. Significantly more late preterm infants required special education in kindergarten and first grade compared with control infants. A trend toward increased enrollment in special education in the third and fourth grade was reported.68 Late preterm infants were considered at increased risk for (1) developmental delay at 3 and 4 years of age, (2) retention in kindergarten, and (3) referral for special education.69 However, maternal age and education were significantly decreased in late preterm infants compared with infants born at term gestation. In addition, the use of Medicaid, insufficient medical care, and maternal tobacco use were higher in the mothers of late preterm infants. These multiple factors, as well as the home environment, are significant risk factors in determining the effects of late preterm delivery on long-term outcome. Regardless of the specific insult, late preterm infants are at increased risk of neurodevelopmental disabilities and should receive timely developmental follow-up to identify potential underachievement and behavioral problems.
Clinical management: neonatal period
Educational requirements for therapists
Examination of and intervention for neonates are advanced-level, not entry-level, clinical competencies. Neonatology is a recognized subspecialty within the specialty areas of pediatric physical therapy70 and pediatric occupational therapy.71 No amount of literature review, self-study, or experience with other pediatric populations can substitute for competency-based, clinical training with a preceptor in an NICU. The potential for causing harm to medically fragile infants during well intentioned intervention is enormous.72–74 The ongoing clinical decisions made by neonatal therapists in evaluating and managing physiological and musculoskeletal risks while handling small (2 or 3 lb), potentially unstable infants in the NICU should not be a trial-and-error experience at the infant’s expense. Therapists with adult-oriented training and even those with general pediatric clinical training (excluding neonatal) are not qualified for neonatal practice without a supervised clinical practicum (2 to 6 months). The NICU is not an appropriate practice area for physical therapy assistants, occupational therapy assistants, or student therapists on affiliations for reasons outlined by Sweeney and colleagues8: “handling of vulnerable infants in the NICU requires ongoing examination, interpretation, and multiple adjustments of procedures, interventions, and sequences to minimize risk for infants who are physiologically, behaviorally, and motorically unstable or potentially unstable.” The physical or occupational therapy assistant and student therapist are not prepared, even with supervision, to “provide moment-to-moment examination and evaluation of the infant and have the ability to modify or stop preplanned interventions when the infant’s behavior, motor, or physiological organization begins to move outside the limits of stability with handling or feeding.”8 Appropriate nonhandling experiences for physical therapist or occupational therapist students in the NICU are delineated by Rapport and colleagues,75 with a wide range of observational learning experiences with a preceptor recommended in this specialized practice environment. Refer to Box 11-1 for appropriate nonhandling experiences for entry-level students.
Delineation of advanced-level roles, competencies, and knowledge for the physical therapist75–77 and the occupational therapist71 in the NICU setting have been described separately by national task forces from the American Physical Therapy Association and the American Occupational Therapy Association. These practice guidelines provide a structure for assessing competence of individual therapists working in NICU settings and offer a framework for designing clinical paths for specific neonatal therapy services.
A gradual, sequential entry to neonatal practice is advised by building clinical experience with infants born at term gestation as well as with physiologically fragile older infants and children and their parents. The experience may include managing caseloads of hospitalized children on physiological monitoring equipment, external feeding lines, and supplemental oxygen or ventilators. Participating in discharge planning and in outpatient follow-up of high-risk neonates are other options for providing exposure to examination, intervention, and family issues when the infants and parents are more stable. This clinical experience and a competency-based, precepted practicum in the NICU offer the best preparation for appropriate, accountable, and ethical practice in neonatal therapy.76–78 In-depth study of perinatal and neonatal medicine and related obstetrical, neonatal nursing, high-risk parenting, and neonatal therapy literature is recommended before pediatric therapy clinicians begin to participate on the intensive care nursery team.
Indications for referral
Tjossem’s79 categories of biological, established, and social risk combined with risk factors for adverse neurodevelopmental outcome80 provide a framework for categorizing indicators for neonatal therapy referral. An overview of developmental risk categories and risk factors for neonatal therapy referral is listed in Box 11-2 to assist clinicians in developing a referral mechanism for a clinical protocol based on risk categories.
Biological risk
Biological risk refers to neurodevelopmental risk attributable to medical or physiological conditions in the prenatal, perinatal, or neonatal period.79–81 Biological risks include placental abnormalities, labor and delivery complications, prenatal infection, and teratogenic factors. Examples of biological risk factors include asphyxia, neonatal seizures, prenatal exposure to drugs or alcohol, and the brain lesions previously described. Birth weight is a strong predictor of outcome; in general, lower birth weight is associated with greater risk of adverse developmental outcomes.82,83
Respiratory disease is generally considered an important risk factor for motor and cognitive disability in infants born preterm (Table 11-5).84 Although the presence of respiratory disease alone does not appear to be predictive of neurodevelopmental outcome, severity of disease does appear to be related to long-term outcome.82 Infants with chronic lung disease or bronchopulmonary dysplasia have been found to be at increased risk for CP and other neurodevelopmental abnormalities compared with preterm infants without bronchopulmonary dysplasia.85,86 Prolonged mechanical ventilation and duration of supplemental oxygen were associated with increased risk of neurodevelopmental disability.87 Administration of surfactant in the neonatal period has reduced the incidence and severity of respiratory disease in very low–birth-weight infants but has not been associated with a decline in neurodevelopmental disability.85
TABLE 11-5
FACTORS CONTRIBUTING TO PULMONARY DYSFUNCTION IN PRETERM NEONATES
Anatomical | Capillary beds not well developed before 26 weeks of gestationType II alveolar cells and surfactant production not mature until 35 weeks of gestation Elastic properties of lung not well developed Lung space decreased by relative size of the heart and abdominal distention Type I, high-oxidative fibers compose only 10% to 20% of diaphragm muscle Highly vascular subependymal germinal matrix not resorbed until 35 weeks of gestation, increasing infant’s vulnerability to hemorrhage Lack of fatty insulation and high surface area/body weight ratio |
Physiological | Increased pulmonary vascular resistance leading to right-to-left shuntingDecreased lung compliance Diaphragmatic fatigue; respiratory failure Decreased or absent cough and gag reflexes; apnea Hypothermia and increased oxygen consumption |
Modified from Crane L: Physical therapy for the neonate with respiratory disease. In Irwin S, Tecklin JS, editors: Cardiopulmonary physical therapy, ed 2, St Louis, 1990, Mosby.
Pain, gestational age, and neurological examination
Multiple neonatal neurological and neurobehavioral examinations have been developed to assess the integrity and maturation of the nervous system88–91 and to describe newborn behavior.9,89 Most of these tests offer information on the quality of motor performance, attention, and interaction. Because these assessments are based on gestational age, an accurate calculation of gestational age is necessary at the time of the testing.92,93
Pain assessment
Despite immature myelinization, premature infants definitely perceive pain and retain the memory of painful experiences. Skin receptors are developed by 14 to 16 weeks’ gestation. In addition, the density of pain receptors in the skin of neonates at 28 weeks of gestation is considered similar to and even exceeds adult density during maturation from birth to 2 years of age.94–96 Blackburn97 explained that although pain transmission in neonates occurs mainly through the slower, unmyelinated C fibers, the shorter distance in neonates that impulses travel to reach the brain compensates for the slower rate of transmission and creates substantial pain reception. Early pain experiences may create later increased sensitivity to pain and vulnerability to stress disorders.98–100 If neonatal therapy assessment or intervention procedures immediately follow a noxious procedure in the NICU, handling techniques may need to be modified or therapy session rescheduled to avoid contributing to a cascade of aversive experiences for the infant.
Psychometric data and clinical use of the pain tools are described for infants as early as 28 weeks of gestation. Many elements in the pain assessments101 have been identified by Als (the Neonatal Individualized Developmental Care and Assessment Program [NIDCAP]) as signs of excessive stimulation and stress in the preterm infant. Specific extremity movements, such as hand to face, elevated leg extension, salute, lateral extension of arms, finger splay, and fisting, have been proposed as indicators of stress and/or pain.102
The Premature Infant Pain Profile (PIPP)103 assigns points for changes in three facial expressions (brow bulge, eye squeeze, and nasolabial fold), heart rate, and oxygen saturation. Gestational age and pre-procedural behavioral state are included in the assessment. The maximal PIPP score is 21; the higher the score, the greater the pain. A score of 0 to 6 points indicates minimal or no pain, whereas a score of 12 or more indicates moderate to severe pain.103
The Face, Legs, Activity, Cry, and Consolability Behavioral tool (FLACC) uses grades of 0 to 2 for facial expression, leg activity, general activity, cry nature, and ability to be consoled and has been used in pediatric and adult settings. This test is capable of assessing pain in normal as well as cognitively impaired children, thus giving it a high degree of versatility and usefulness.104 Change in FLACC score has been used to demonstrate that the use of sucrose and a pacifier during venipuncture is more effective in consoling infants younger than 3 months of age than infants older than 3 months of age.105
The Neonatal Pain, Agitation, and Sedation Scale (N-PASS) uses five indicators: (1) cry and irritability, (2) behavioral state, (3) facial expression, (4) extremity movement and tone, and (5) vital signs. As with the PIPP scale, additional points are added for decreasing gestational age.106 There was good correlation between the N-PASS and the PIPP assessments during routine heelstick in infants younger than 1 month old born at 23 to 42 weeks’ gestation.107
Clinical assessment of gestational age in the newborn infant
A method for clinical assessment of gestational age in the newborn infant was developed by Dubowitz and colleagues92 from data derived from a total of 167 preterm and term infants (28 to 42 weeks’ gestation) tested within 5 days of birth. The tool focuses on criteria for calculation of gestational age from a composite of 10 neurological and 11 external (physical) characteristics.
Calculation of gestational age is an important adjunct to all other neonatal assessment tools. It guides practitioners in interpreting neurological and behavioral findings relative to the expected performance of neonates at various gestational ages. Additional guidelines on gestational differences in neurological, physical, and neuromuscular maturation can be found in the work of French pediatric neurologist Amiel-Tison.88,89,108
Newborn maturity rating—ballard score
Ballard and colleagues109–111 designed a simplified modification of the Dubowitz gestational age tool. It has been widely adopted because of the time efficiency (3 to 4 minutes versus 10 to 15 minutes) and the elimination of active tone items, which are difficult to evaluate reliably in physiologically unstable newborns. The Ballard instrument involves only six physical and six neurological criteria, with a 0 to 5 scale and a maturity rating. It is designed to be used for neonates (20 to 44 weeks gestation) from birth through 3 days of age and has demonstrated concurrent validity with the Dubowitz gestational age calculation tool. The gestational age of the infant is based on the obstetrical dating criteria unless the clinical assessment of the infant deviates more than 2 weeks from the obstetrical calculation.
Neurological examination of the full-term infant
The Neurological Examination of the Full-Term Infant was designed by Prechtl112 to identify abnormal neurological signs in the newborn period. The examination was developed from an investigation of more than 1350 newborns and was standardized on infants born at the gestational age of 38 to 42 weeks. If the test is used in premature infants who have reached an age of 38 to 42 weeks of gestation, lower resistance to passive movements (lower tone) may be expected. Delay of testing until a minimum of 3 days of age is advised to maximize the stability of behavioral states and neuromotor responses for improved reliability and validity of results.
The pattern of examination includes periods of both observation and examination. A 10-minute screening test is offered to determine if the full 30-minute examination of posture, tone, reflexes, and spontaneous movement is required. Although specific requirements for examiner training are not addressed, Prechtl offers a flow diagram (Figure 11-9) to assist clinicians with organizing the neurological examination process. Significant findings from the examination are summarized in the following categories: (1) quality of posture, spontaneous movement, and muscle tone (consistency and resistance to passive movement); (2) presence of involuntary or pathological movements (clonus, tremor, athetoid postures or movements); (3) behavioral state changes and quality of cry; and (4) threshold or intensity of responses to stimulation. (Because of the transient pattern of neurological signs and rapid changes in the developing nervous system, Prechtl advised repeated examinations to monitor neurological status.)
Neonatal behavioral assessment scale
To document individual behavioral and motor differences in infants at term gestation to 2 months of age, Brazelton and Nugent113 developed a neonatal behavior scale to assess neuromotor responses within a behavioral state context. The 30- to 45-minute examination consists of observing, eliciting, and scoring 28 biobehavioral items on a 9-point scale and 18 reflex items on a 4-point scale. This is an interactive test and assesses the infant’s ability to recover from stimuli and return to an alert state. The reflex items are derived from the neurological examination protocol of Prechtl and Beintema.114
The scale was designed to assess newborn behavior in healthy 3-day-old term (40 weeks of gestation) white infants whose mothers had minimal sedative medication during an uncomplicated labor and delivery. Use of this examination with infants born preterm requires modification of the examination procedure to the environmental constraints of an intensive care nursery and interpretation of findings relative to the gestational age and medical condition of the infant. For preterm infants approaching term gestation (minimum of 36 weeks of gestation), nine supplementary behavioral items are offered. Many of these items were developed by Als9 for use with preterm and physiologically stressed infants (see discussion of the APIB, later). In the manual,113 methods of adapting the Neonatal Behavioral Assessment Scale (NBAS) for preterm neonates with accompanying case scenarios are described to illustrate use of the findings to enhance parent-infant interaction and guide developmental interventions.
Motor behavior refers to the ability to modulate muscle tone and motor control for the performance of integrated motor skills, such as the hand-to-mouth maneuver, pull-to-sit maneuver, and defensive reaction (e.g., removal of cloth from face). In the assessment of behavioral state organization, the infant’s ability to organize behavioral states when stimulated and the ability to shut out irritating environmental stimuli when sleeping are analyzed. Physiological organization is evaluated by observing the infant’s ability to manage physiological stress (changes of skin color, frequency of tremulous movement in the chin and extremities, number of startle reactions during the assessment). For analysis, the information is divided into seven clusters: habituation, orientation, motor, range of state, regulation of state, autonomic stability, and reflexes. The cluster systems are highly useful for clinical interpretation and for data analysis in clinical research. Performance profiles of worrisome or deficient interactive-motor and organizational behavior are identified by clusters of behavior associated with potential developmental risk.115
Participation of the parent in the newborn assessment may yield long-term positive effects on infant-parent interaction and later on cognitive and fine motor development. Widmayer and Field116reported significantly better face-to-face interaction and fine motor-adaptive skills at 4 months of age and higher mental development scores at 12 months of age when teenage mothers of preterm infants (mean gestational age at birth, 35.1 weeks) were given demonstrations of the NBAS. These demonstrations were scheduled when the premature infants had reached an age equivalence of 37 weeks of gestation.
Nugent117,118developed parental teaching guidelines for using the NBAS as an intervention for infants and their families. Published by the March of Dimes birth defects foundation, the guidelines offer strategies for interpreting each item according to its adaptive and developmental significance, descriptions of the expected developmental course of the behavior (item) over several months, and recommendations for caregiving according to the infant’s response to the items.
A three-step examiner training involving self-study, practice, and certification phases is coordinated through the Brazelton Institute, Children’s Hospital, Boston, Massachusetts.119,120 Wilhelm115 recommended NBAS training for clinicians beginning to develop competence in examining at-risk infants. She explained that it provides a system for developing basic handling skills with healthy, term infants without concerns of stressing medically fragile preterm infants during the training period. Learning the NBAS in term infants before entering NICU practice provides familiarity with similar testing and scoring procedures for preterm infants.115
Newborn behavioral observations system
The Newborn Behavioral Observations (NBO) system, developed from the pioneering work and philosophy of Brazelton, is an interactive, observational tool for use with infants and parents in hospital, clinic, and home settings.121 The focus is on prematurely born infants and at-risk infants, with emphasis on cultural competence, family-centered care, and infant development. The NBO system helps determine the behavioral profile of the infant and allows the practitioner to provide parents with individualized and unique information about their infant. This behavioral information promotes positive parent-infant interaction and also a positive partnership between parents and practitioners.
Certification in administering, interpreting, and scoring the 18-item NBO assessment is arranged through the Brazelton Institute in a 2-consecutive-day format. The training encompasses the following observation categories: (1) habituation to external light and sound; (2) muscle tone and motor activity level; (3) behavioral self-regulation (crying and consolability); and (4) visual, auditory, and social-interactive abilities.119,120
Neurological assessment of the preterm and full-term newborn infant
The Neurological Assessment of the Preterm and Full-Term Newborn Infant is a streamlined neurological and neurobehavioral assessment designed by Dubowitz and colleagues122 to provide both a systematic, quickly administered newborn examination applicable to infants born preterm or at term gestation and a longer infant examination for children to 24 months of age. A distinct advantage of this tool is the minimal training or experience required by the examiner and the ease of adapting it to the infant and the environment. The adaptability of the test and use of the scoring form with stick figure diagrams have made it useful for implementation in developing countries where English is not widely spoken.
The 15 items that assess movement and tone and the six reflex items evolved from clinical trials on 50 term infants using the clinical assessment of gestational age by Dubowitz and colleagues,92 the neurological examination of the newborn by Parmelee and Michaelis,123 and the neurological examination of the full-term newborn infant by Prechtl.112 The examination format was then used during a 2-year period on more than 500 infants of varying gestational ages. After 15 years the authors revised the assessment in the second edition by eliminating seven items, expanding the tone pattern section, and developing an optimality score. Reliability data are not reported, but modification of examination procedures occurred during the pilot phase that promoted objectivity in scoring and a high interrater reliability among examiners, regardless of experience level.
Evolution of neurological patterns in infants with IVH, PVL, and HIE is described in the test manual and correlated with brain imaging. Abnormal neonatal clinical signs associated with long-term neurological sequelae were persistent asymmetry, decreased lower-extremity movement, and increased tone. Infants with IVH had significantly higher incidence of abnormally tight popliteal angles, reduced mobility, decreased visual fixing and following, and roving eye movements. The authors cautioned that early signs of motor asymmetry in neonates with cerebral infarction may be associated with normal outcome, but normal neonatal neurological examinations after cerebral infarction do not exclude the possibility of later hemiplegia.124
Long-term follow-up data beyond 1 year have not been reported with this examination. Dubowitz and colleagues125 reassessed 116 infants (27 to 34 weeks of gestation) at 1 year of age. Of 62 infants assessed as neurologically normal in the newborn period, 91% were also normal at 1 year of age. Of 39 infants assessed as neurologically abnormal in the newborn period, 35% were found to be normal at 1 year of age. According to Wilhelm,115 the predictive value of a negative test result with this instrument was 92%, but the predictive value of a positive test result was only 64%.
Interpretations of evaluative findings from the Neurological Assessment for Preterm and Full-Term Newborn Infants for neonatal therapy practice are comprehensively described in a case study format by Heriza126 and Campbell.127 Dubowitz128 discussed the clinical significance of neurological variations in infants and offered decision guidelines to clinicians on when to worry, reassure, or intervene with developmental referrals.
Assessment of preterm infants’ behavior
Als9 designed the APIB to structure a comprehensive observation of a preterm infant’s autonomic, adaptive, and interactive responses to graded handling and environmental stimuli. It involves six maneuvers with increasing challenging and complex interactions with a highly structured format. As previously described in the theoretical framework section of this chapter, this assessment is derived from synactive theory and is focused on assessing the organization and balance of the infant’s physiological, motor, behavioral state, attention and interaction, and self-regulation subsystems. The APIB has testing sequences and a scoring format similar to those used in Brazelton’s NBAS, with increased complexity and expansion for premature infants.
Neonatal individualized developmental care and assessment program
Als11 and Als and colleagues11,129 developed NIDCAP to document the effects of the caregiving environment on the neurobehavioral stability of neonates. This naturalistic observation protocol includes continuous observation and documentation at 2-minute intervals of an infant’s behavioral state and autonomic, motor, and attention signals, with simultaneous recording of vital signs and oxygen saturation. Documentation occurs before, during, and after routine caregiving procedures. The infant’s strengths, weaknesses, and coping skills are identified. A narrative description of the infant’s responses to the stress of handling by the primary nurse and to auditory and visual stimuli in the NICU environment is provided to assist caregivers and parents in identifying the infant’s behavioral cues and providing appropriate interaction. Options are described in the care plans for reducing aversive environmental stimuli and modifying physical handling procedures. This clinical tool allows neonatal therapists to determine the infant’s readiness for assessment and intervention by observing the baseline tolerance of the infant to routine nursing care before superimposing neonatal therapy procedures.130 Sequential observations occur weekly or biweekly. Parental involvement is strongly encouraged and instrumental in facilitating a smooth transition to home. Examiner training in the NIDCAP may be coordinated through the National Training Center at Children’s Hospital Boston, Massachusetts, where priority is now given to training NICU teams rather than individuals.10
Nicu network neurobehavioral scale
Lester and Tronick131 developed a tool for preterm and drug-exposed infants from 30 weeks of gestation to 6 weeks postterm. The test includes items from the NBAS, APIB, Finnegan abstinence scale, and other neurological assessments and consists of 115 items in general categories of neurological and neuromotor integrity (tone, reflexes, and posture), behavioral state and interaction (self-regulatory competence), and physiological stress abstinence signs (drug-exposed infants). This test is state dependent and gives a comprehensive and integrated picture of the infant that is not divided into clusters. More than half118 of the test items are infant observations, and 45 items require physical handling of the infant. Test-retest reliability of preterm infants indicated correlations of 0.30 to 0.44 at 34, 40, and 44 weeks of gestation. This test is useful for management of drug-exposed infants but may have limited predictive value. Training and certification in administration and scoring of the test are coordinated through Brookes Publishing Company and available in the United States and internationally with use of videoconferencing for lectures and demonstrations.
Test of infant motor performance
Developed by Campbell and colleagues,132 the 42-item Test of Infant Motor Performance (TIMP) is focused on evaluating postural control, spontaneous movement, and head control for neonates at 32 weeks of gestation to 16 weeks postterm. Functional motor performance is assessed through observation of infant movement and through responses to various body positions and to visual or auditory stimuli. Psychometric qualities of the test include (1) construct validity133 and ecological validity,134 (2) concurrent validity at 3 months of age with the Alberta Infant Motor Scale (AIMS),135 and (3) predictive validity at 5 to 6 years of age with the Bruininks-Oseretsky Test of Motor Proficiency136 and at 4 to 5 years of age with the Peabody Developmental Motor Scales and Home Observation for Measurement of the Environment: Early Childhood.137 Training on test procedures is available through 2-day workshops or through a self-guided training method with a CD-ROM from the test developer.138
Neurobehavioral assessment of the preterm infant
The Neurobehavioral Assessment of the Preterm Infant (NAPI) was developed by Korner as a developmental test to assess medically stable infants from 32 weeks to term gestation using a sequence of specific movements. This test focuses on tone, reflexes, movement, response to visual and auditory stimulation, and observation of cry and state. This tool does not require a specific preassessment state as is required by the previously mentioned tests, but starts with the infant asleep. It does not take as long to administer (less than ½ hour) than the previously described tests and is easy to analyze. The data are categorized into seven clusters and compared with standardized scores. With repeated examinations over time, persistent deviations from the normative scores indicate that the infant is at risk for developmental delays and is in need of close follow-up. In addition, the NAPI has been shown to be predictive of short-term and long-term neurodevelopmental outcomes.139
Qualitative assessment of general movements
The assessment tools reviewed so far in this chapter require direct handling of the infant. Infants born preterm are particularly vulnerable to developing physiological stress during the maneuvers required by most tools available for infant assessment. Instead, noninvasive, repeated longitudinal observation and assessment are needed to accommodate the concurrent motor variability, immature nervous system, and physiological vulnerability of the young, preterm infant.140 Based on the pioneering work of Prechtl examining the continuity of prenatal to postnatal fetal movement,141 this criterion-referenced test focuses on evaluating the quality of spontaneously generated movements in preterm, term, and young infants until 16 weeks postterm. A wide repertoire of endogenously generated spontaneous motility in the fetus including isolated limb movements, stretches, hiccups, yawning, and breathing movements can be identified as early as 9 weeks.141–143
General movements (GMs) are spontaneously generated complex movements involving the trunk, limbs, and neck. They vary in speed and intensity with a gradual onset, increase in speed and intensity, and a gradual end. These movements are among the number of movement patterns that emerge during fetal life and continue until approximately 16 weeks postterm, when goal-oriented and voluntary movements appear.144 The quality of movement is assessed through observation and scoring of videotaped spontaneous movement of an infant in supine position without stimulation or handling.144 A distinct difference occurs between the GMs in the preterm infant and those in the term and postterm infant. GMs in the term infant, and for the first 8 weeks, change in amplitude and speed, taking on a writhing quality. The writhing movements gradually give way to the fidgety movements, which are present in awake infants between 9 and 16 to 20 weeks postterm. Fidgety movements are small, circular movements of small amplitude and varying speed involving the neck, trunk, and extremities.140 Other approaches to neurological assessment can be found in additional references.140,144,145
This neonatal and young infant assessment instrument has gained substantial attention in the past 20 years for its high reliability, sensitivity, and predictive validity. In a comprehensive review of the psychometric qualities of neuromotor assessments for infants, the GM assessment was rated among the tools with the highest reliability, averaging interrater and intrarater correlation coefficient, or k, greater than 0.85.146 Multiple studies have corroborated the predictive validity and sensitivity of this method. The sensitivity is lower during the preterm period and during the writhing movements, improving during the fidgety movement period. Sensitivity as high as 95% has been reported.140,147
Testing variables
Neuromuscular and behavioral findings in the newborn period may be influenced by several variables. Increased reliability in examination results and in clinical impressions may occur when these variables are recognized. Medication may produce side effects of low muscle tone, drowsiness, and lethargy. Such medications include anticonvulsants, sedatives for diagnostic procedures (CT scan, electroencephalography, electromyography), and medication for postsurgical pain management. Intermittent subtle seizures may produce changes in muscle tension and in the level of responsiveness. Mild, ongoing seizures may occur in the neonate as lip smacking or sucking, staring or horizontal gaze, apnea, and bradycardia. Stiffening of the extremities occurs in neonatal seizures more frequently than clonic movement. Fatigue from medical and nursing procedures can result in decreased tolerance to handling, decreased interaction, and magnified muscle tone abnormalities. Fatigue may also result when neurodevelopmental assessment is scheduled immediately after laboratory (hematologic) procedures, suctioning, ultrasonography, or respiratory (chest percussion) therapy. Tremulous movement in the extremities may be linked to conditions of metabolic imbalance (hypomagnesemia, hypocalcemia, hypoglycemia), and low muscle tone may be associated with hyperbilirubinemia, hypoglycemia, hypoxemia, and hypothermia.148,149
Summary
Practitioners must be aware of the normative and validation data and of the predictive characteristics of the test(s) administered to allow appropriate interpretation of the results. Specific clinical training with a preceptor is essential to administer, score, and interpret neonatal assessment instruments accurately; to establish interrater reliability; and to plan treatment based on the evaluative findings. Even low-risk, healthy preterm infants are vulnerable to becoming physiologically and behaviorally destabilized during neurological assessment procedures.150–152 This risk is reduced with precepted, competency-based clinical training in the NICU.
Intervention planning
Level of stimulation
Physiological and musculoskeletal risk management
Many maturation-related anatomical and physiological factors predispose preterm infants to respiratory dysfunction (see Table 11-5). For this reason many preterm neonates require the use of a wide range of respiratory equipment and physiological monitors (Table 11-6). Pediatric therapists preparing to work in the NICU and those involved with designing risk management plans are referred to the neonatal nursing literature for evidence and perspectives on assessing and managing neonatal stressors during interventions in the NICU.153–155 Because infants born prematurely or experiencing critical illness communicate via subtle behavioral cues, their understated language is “not easily interpreted unless caregivers understand how infants’ ability to respond to stress reflects their maturation and neurodevelopment.”156 Their behavioral cues are considered more subtle and more likely to be disregarded than those of infants born at term gestation.
TABLE 11-6
EQUIPMENT COMMONLY ENCOUNTERED IN THE NEONATAL INTENSIVE CARE UNIT (NICU)
EQUIPMENT | DESCRIPTION |
Thermoregulation radiant warmer | |
Unit composed of mattress on an adjustable tabletop covered by a radiant heat source controlled manually and by servocontrol mode. Unit has adjustable side panels.Advantage: provides ready access to infant and increases area for equipment. Disadvantage: leads to convective heat loss, increases insensible fluid loss, and encourages stimulation (excessive). |
|
Double-walled Isolette | Enclosed unit of transparent material providing a heated and humidified environment with a servocontrol incubator system of temperature monitoring. |
Access to infant through side portholes or opening side of unit.Advantage: barrier to tactile stimulation, decreased convective losses. Disadvantage: more difficult to get to infant, does not decrease noise from NICU, radiant heat loss if Isolette is single walled. |
|
Thermal shield | Clear acrylic dome or plastic wrap placed over the trunk and legs of an infant in an Isolette to reduce radiant heat loss. |
Respiratory assistance | |
Conventional pressureVolume ventilator | Delivers positive-pressure ventilation; pressure limited, with volume delivered dependent on the stiffness of the lung.Delivers positive-pressure ventilation; volume limited, delivering same tidal volume with each breath, potentially decreasing barotraumas, as most use minimal pressure required to deliver a set tidal volume; common ventilator in use. |
High frequency ventilator | Ventilator that delivers short bursts of air at high rates of flow (240-600 breaths/min). |
Jet | Active inhalation with passive exhalation; requires conventional ventilator, noisy. |
Oscillatory | Piston driven; active inhalation and exhalation. |
Continuous positive airway pressure (CPAP) device | Nasal prongs of varying lengths provide CPAP and controlled oxygen delivery. Using bubble CPAP, positive pressure is adjusted by altering the depth of the expiratory tubing, which is under liquid. CPAP prongs can also be connected to a mechanical ventilator to deliver adjustable pressure and a breath rate if required. |
Nasal cannula | Specific concentration of oxygen is delivered via soft nasal cannula, usually less than 1 L/min. High-flow nasal cannula delivers humidified oxygen at flows up to 6 L/min and a variable amount of distending pressure to help with alveolar inflation. |
Oxyhood | Clear acrylic plastic hood fitting over the infant’s head to provide an environment for delivering controlled oxygen and humidification delivery. |
Monitors | |
Cardiac, respiratory | One unit will display heart rate, respiratory rate, and blood pressure. High and low alarm limits can be set. |
Oxygen saturation | Measures peripheral oxygen saturation and pulse from a light sensor secured to the infant’s skin. Values can be displayed on the monitor. |
Transcutaneous | Noninvasive method of monitoring partial pressure of O2 and CO2 from arterialized capillaries through the skin through the use of a heated sensor. |
Cerebral oxygenation | Noninvasive method to measure regional oxygen saturation, usually cerebral and somatic, to ensure adequate oxygen delivery. |
Amplitude-integrated electroencephalography (aEEG) | Continuous recording of cerebral electrical activity used to evaluate presence of seizures, baseline brain activity, and brain maturation. |
Intravenous catheter | Used to deliver intravenous fluids, intralipids, and medications at a specific rate and to assist in obtaining blood for analysis. Specific catheters include arterial and venous umbilical lines, peripherally inserted central catheters (PICCs), surgically placed central catheter (Broviac, Cook), and peripheral intravenous catheter. |
Extracorporeal membrane oxygenation (ECMO) | Heart-lung-kidney machine used for term infants with severe respiratory or cardiac failure. |
Areas of particular concern during neonatal therapy activities include potential incidence of fracture, dislocation, or joint effusion during the management of limited joint motion; skin breakdown or vascular compromise during splinting or taping to reduce deformity; apnea or bradycardia during therapeutic neuromotor handling with potential deterioration to respiratory arrest; oxygen desaturation or regurgitation and aspiration during feeding assessment or oral-motor therapy; hypothermia from prolonged handling of the infant away from the neutral thermal environment of the incubator or overhead radiant warmer; and propagation of infection from inadequate compliance with infection control procedures in the nursery. Signs of overstimulation may include labored breathing with chest retractions, grunting, nostril flaring, color changes (skin mottling, paleness, gray-blue cyanotic appearance), frequent startles, irritability or drowsiness, sneezing, gaze aversion, bowel movement, and hiccups. Signals of overstimulation expressed through infants’ motor systems are finger splay (extension and abduction posturing), arm salute (shoulder flexion with elbow extension), and trunk arching away from stimulation.11 Harrison and colleagues154 found that motor activity cues of preterm infants were correlated with low oxygen saturation and should be carefully monitored during caregiving procedures to minimize physiological instability.
Even a baseline neurological examination, usually presumed to be a benign clinical procedure, may be destabilizing to the newborn infant’s cardiovascular and behavioral organization systems. The physiological and behavioral tolerance of low-risk preterm and term neonates to evaluative handling by a neonatal physical therapist was studied in 72 newborn subjects.151 During and after administration of the Neurological Assessment of the Preterm and Full-Term Newborn Infant, preterm subjects (30 to 35 weeks of gestation) had significantly higher heart rates; greater increases in blood pressure; decreased peripheral oxygenation inferred from mottled skin color; and higher frequencies of finger splay, arm salute, hiccups, and yawns than in term subjects. Neonatal practitioners must examine the safety of even a neurological examination and weigh the risks and anticipated benefit of the procedure given the expected physiological and behavioral changes in low-risk, medically stable neonates.150,151
High-risk profiles
The first high-risk profile involves the irritable, hypertonic infant. These infants classically have a low tolerance level to handling and may frequently reach a state of overstimulation from routine nursing care, laboratory procedures, and the presence of respiratory and infusion equipment. They may express discomfort when given quick changes in body position by caregivers and when placed in any position for a prolonged time. Predominant extension patterns of posture and movement are associated with this category of infants. Quality of movement may appear tremulous or disorganized, with poor midline orientation and limited antigravity movement into flexion as a result of the imbalance of increased proximal extensor tone. Visual tracking and feeding may be difficult because of extension posturing or the presence of distracting, disorganized upper-extremity movement. In addition, increased tone with related decreased mobility in oral musculature may complicate feeding behavior. Hypertonic infants frequently demonstrate poor self-quieting abilities and may require consistent intervention by caregivers to tolerate movement and position changes. These temperament characteristics and the signs of neurological impairment previously discussed may place infants at considerable risk for child abuse or neglect as the stress and fatigue levels of parents rise and as coping strategies wear thin during the demanding care required by irritable, hypertonic infants.7,157 Hypertonic, irritable infants constituted large percentages of neonatal therapy caseloads in the 1970s through the 1990s, but advances in neonatal pulmonary management have now decreased the numbers of infants matching this neurobehavioral profile.
Timing
Neonatal therapists should not interrupt infants in a quiet, deep sleep state but instead wait approximately 15 minutes until the infant cycles into a light, active sleep or semiawake state. Higher peripheral oxygen saturation has been correlated with quiet rather than with active sleep in neonates. Preterm infants reportedly have a higher percentage of active sleep periods in contrast to the higher percentage of quiet sleep observed in term infants.158 Allowing the preterm infant to maintain a deep, quiet sleep by not interrupting is a therapeutic strategy for enhancing physiological stability.
Treatment strategies
This section addresses components of treatment for enhancing movement, minimizing contractures and deformity, promoting feeding behaviors appropriate to corrected age, developing social interaction behaviors, and fostering attachment to primary caregivers. Management approaches to body positioning, extremity taping, graded sensory and neuromotor intervention, neonatal hydrotherapy, and oral-motor and feeding therapy are presented; parental teaching is discussed here. Evidence-based practice recommendations for neonatal therapy are outlined in Table 11-7 and Box 11-3. In managing an intensive care unit caseload, the constant physiological monitoring; modification of techniques to adapt to the constraints of varying amounts of medical equipment; scheduling of interventions to coincide with visits of the parents and peak responsiveness of the infants; and ongoing coordination and reevaluation of goals, plans, and follow-up recommendations with the nursery staff create many interesting challenges and demand a high degree of adaptability and creativity from the clinician. Willingness to change an established assessment plan, treatment strategy, or therapy schedule to meet the immediate needs of the infant, parents, or nursery staff is paramount. For some infants with prolonged periods of only borderline stability with handling, a discharge examination with recommendations for follow-up care may be the best practice. Productivity standards of billable hours used for other caseloads of stable pediatric or adult clients in the hospital are not appropriate for the NICU setting and necessitate negotiation and reinterpretation with rehabilitation or therapy department managers to protect both the infant and the neonatal therapist. Tolley159 reported an expected mean productivity of 5.0 billable hours (range 4 to 6.5 hours) for hospital-based pediatric physical therapists among hospitals surveyed across 32 states and the District of Columbia.93
TABLE 11-7
EVIDENCE-BASED RECOMMENDATIONS FOR NEONATAL PHYSICAL THERAPY
TYPE | RECOMMENDATIONS | LEVEL OF EVIDENCE | REFERENCES |
Prevention | Collaborate with caregivers to reduce risk of skull deformity, torticollis, and extremity malalignment through diligent positioning for symmetry and neutral alignment | Level II | Van Vlimmeren et al, 2007244 |
Level II | Vaivre-Douret et al, 2004303 | ||
Level II | Monterosso et al, 2003169 | ||
Examination | Conduct baseline observation to determine physiological and behavioral stability (readiness) for evaluative handling | Level II | Sweeney, 1986150 |
Provide continuous physiological and behavioral monitoring during and after evaluative handling to determine adaptation to evaluative handling and to signal the need for modification of pace and sequence, given expected physiological changes, particularly during neuromotor test procedures | Level II | Sweeney, 1989151 | |
Intervention | Collaborate with caregivers to create a developmentally supportive environment with modulated stimulation from light, noise, and handling | Level I | Symington and Pinelli, 2006304 |
Level II | Westrup et al, 2004305 | ||
Level I | Peters et al, 2009306 | ||
Support body position and extremity movement—(1) supine: semiflexed, midline alignment using blanket for swaddling containment or “nest” of positioning rolls; (2) prone: vertical roll under thorax; horizontal roll under hips | Level II | Vaivre-Douret et al, 2004303 | |
Level II | Monterosso et al, 2003169 | ||
Level II | Short et al, 1996307 | ||
Level II | Ferrari et al, 2007166 | ||
In selected neonates with movement impairment or disorganization consider therapeutic handling carefully graded in intensity and paced to facilitate head and trunk control, antigravity movement, and midline orientation | Level II | Girolami and Campbell, 1994308 | |
Consider gradual exposure to multimodal stimuli for stable neonates approaching hospital discharge | Level I | Symington and Pinelli, 2006304 | |
Provide opportunities for independent oral exploration through positioning with hands to face, and for nonnutritive sucking to improve state organization and readiness to feed | Level I | Pinelli and Symington, 2005197 | |
Determine readiness for and advancement of oral feeding trials using infant behavioral cues | Level II | Kirk, Alder, and King, 2007198 | |
Level II | McGrath and Medoff-Cooper, 2002309 | ||
Encourage parental involvement with feeding while providing interventions for physiological stability (pacing and slowed flow rate) | Level III | Law-Morstatt et al, 2003206 | |
Level II | Chang et al, 2007207 | ||
Consider hydrotherapy before feeding for stable infants with movement impairment | Level IV | Sweeney, 2003191 | |
Education | Educate parents on behavioral cues and developmental status to mitigate parental stress and improve parental mental health outcomes | Level II | Kaaresen et al, 2006310 |
Level I | Melnyk et al, 2006311 | ||
Implement multiple methods of instruction for parents and caregivers (demonstration, discussion, video, and written materials) | Level V | Dusing, Murray, and Stern, 2008212 |
From Sweeney JK, Heriza CB, Blanchard Y, Dusing SC: Neonatal physical therapy. Part II: Practice frameworks and evidence-based practice guidelines. Ped Phys Ther 22:2-16, 2010.
Positioning
Imbalance of excessive extension may occur in preterm infants with prolonged mechanical ventilation who appear to gain postural stability in the nonfluid extrauterine environment by leaning into or stabilizing against a firm mattress while in the supine position. De Groot160 explained the postural behavior of preterm infants as an imbalance between low passive muscle tone and active muscle power. She theorized that because preterm neonates have prolonged periods of immobility (often in the supine position), exaggerated active muscle power may be observed in the extensor musculature, particularly in the trunk and hips. This imbalance of extension is viewed as nonoptimal muscle power regulation that may negatively influence postural stability, coordinated movement, and later hand and perceptual skills.160
Some neonates, especially those born at less than 30 weeks of gestation, may attempt to posturally stabilize by hyperextending the neck in supine or side-lying positions to compensate for maturation-related hypotonia.161 Neck hyperextension posturing, without a balance of movement into flexion, may trigger later development of a host of related abnormal postural and mobility patterns to compensate for inadequate proximal stability.160 In some infants, excessive postural stabilizing into neck hyperextension may contribute to sequential blocking of mobility in the shoulder, pelvis, and hip regions. The potential components of this high-risk, hypertonic postural profile appear in Box 11-4.
Shaping of the musculoskeletal system occurs during each body position experienced by neonates in the NICU. A variety of positional deformities in the extremities and skull can result from inattention to alignment. In Table 11-8, common neonatal positional deformities, musculoskeletal consequences, and functional limitations are outlined. Supporting the skeletal integrity of infants born prematurely is challenging in the midst of numerous equipment obstacles, restricted physical handling because of physiological instability, and limited spontaneous movement. Infants with gastroesophageal reflux are frequently positioned on wedges that make symmetrical, midline postures difficult to maintain. Skull flattening may continue to evolve after NICU discharge from overuse of infant seats, reflux wedges, and limited prone play experiences. Plagiocephaly (asymmetrical occipital flattening) and a secondary torticollis may emerge when a strong head turn preference remains and parents do not vary the direction of head turn for sleeping and infant seat use.
TABLE 11-8
MUSCULOSKELETAL MALALIGNMENT AND FUNCTIONAL LIMITATIONS IN NEONATES
POSITIONAL DEFORMITY | CONSEQUENCES | FUNCTIONAL LIMITATIONS |
Plagiocephaly | Unilateral, flat occipital region; head turn preference; high risk for torticollis | Limited visual orientation from asymmetrical head position; delayed midline head control |
Scaphocephaly | Bilateral, flat parietal and temporal regions | Difficulty developing active midline head control in supine position from narrowing of occipital region |
Hyperextended neck and retracted shoulders | Shortened neck extensor muscles; overstretched neck flexor muscles; excessive cervical lordosis; shortened scapular adductor muscles | Interferes with head centering and midline arm movement in supine position; interferes with head control in prone and sitting positions; limits downward visual gaze |
“Frog” legs | Shortened hip abductor muscles and iliotibial bands; increased external tibial torsion | Interferes with movement transitions into and out of sitting and prone positions; interferes with hip stability in four-point crawling; prolonged wide-based gait with excessive out-toeing |
Everted feet | Overstretched ankle invertor muscles; altered foot alignment from muscle imbalance | Pronated foot position on standing; retained, immature foot-flat gait with potential delay in development of heel-to-toe gait pattern from excessive pronation |
Adapted from Sweeney JK, Gutierrez T: The dynamic continuum of motor and musculoskeletal development: implications for neonatal care and discharge teaching. In Kenner C, McGrath JM, editors: Developmental care of newborns and infants, ed 2, Glenview, Ill., 2010, National Association of Neonatal Nurses.
Retracted shoulder posture (scapular adduction with shoulder elevation and external rotation) may accompany excessive neck and trunk extension posture in preterm infants. This abnormal posture can interfere with later reaching, shoulder stability in the prone position, and rolling during the first 18 months of life.162,163 Excessive tibial torsion and out-toeing gait were reported in preterm infants at 3 to 8 years of age and traced to prolonged “frog leg” (excessive abduction and external rotation with foot eversion) in the NICU.164,165
Goals of neonatal positioning procedures include the following:
Optimize alignment toward neutral neck-trunk position, semiflexed, midline extremity posture, and neutral foot position
Support posture and alignment within “containment boundaries” of rolls, swaddling blanket, or other positioning aids; avoid creating a barrier to spontaneous movement, and allow space for controlled extremity movement
Create positions that promote alert states for enhanced short-duration interaction and sleep states that promote comfort and physiological stability
Offer positions that allow controlled, individualized exposure to proprioceptive, tactile, visual, or auditory stimuli while monitoring signs of behavioral and physiological stress from potential overstimulation
The use of blanket or cloth diaper rolls or customized foam inserts in a neonatal positioning program may modify increasing imbalance of extension in selected preterm or chronically ill infants and promote movement and postural stability from positions of flexion. After the infant is facilitated into a flexed posture in the side-lying position, posterior rolls behind the head, trunk, and thighs provide a surface against which the infant can posturally stabilize while a flexed midline posture is maintained (Figure 11-10). An additional anterior roll between the extremities and the use of a pacifier may promote further midline stabilization in flexion (Figure 11-11). Small neonates can be maintained in a flexed, symmetrical posture in a circular nest formed from a long blanket roll. Cloth buntings with circumferential body straps and a foot roll (Figure 11-12) provide positioning support and containment of extremity movement. Ferrari and colleagues166 found increased frequency of extremity movements across midline and fewer stiff postures when neonates (25 to 31 weeks of gestation) were positioned in supine position in a circular nest compared with supine position without a nest.
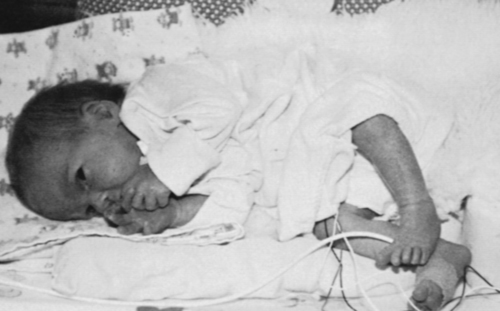

Endotracheal tube placement frequently contributes to the neck hyperextension posture in infants who require mechanical ventilation (Figure 11-13). This iatrogenic component can be avoided by repositioning the ventilator hoses to allow enough mobility for slightly tucked chin and partially flexed trunk posture. For neurologically impaired infants with severe pulmonary disease necessitating prolonged ventilatory support, inattention to the alignment of the neck and shoulders may lead to the development of a contracture in the neck extensor muscles (Figure 11-14).
During the hospital stay, infants on ventilators (Figure 11-15) are now routinely positioned prone to enhance extremity and trunk flexion in the prone position, improve oxygenation, and decrease irritability.167,168 Monterosso and colleagues169 reported that using a small vertical roll along the torso (sternum to pubis) decreased scapular retraction in the prone position. Although placing infants on a sheepskin surface (see Figure 11-12) offers increased tactile input and has been correlated with increased weight gain in LBW infants compared with a matched group of infants on standard cotton sheets,170 concern regarding the inhalation of microfibers from sheepskin has limited widespread use unless the sheepskin is covered by a thin blanket and used only to midchest level.171
After the infant has been moved from intensive care to intermediate care, transition to a standard mattress without positioning aids is recommended to allow time for adaptation to the type of mattress likely to be used at home. Infants are also transitioned to the supine sleeping position during the week before hospital discharge to reduce the risk of sudden infant death associated with the prone sleeping position and other factors.171
Preliminary evidence has been reported to support neonatal positioning programs emphasizing postures of extremities in flexion and head in midline.166,172 Clinicians are referred to the work of Hunter173,174 for detailed positioning techniques in the NICU. Continued research efforts are needed to measure effects of positioning and the risk-benefit effects of other neonatal therapy interventions to guide future directions of neonatal practice.
Extremity taping
The presence of perinatal elasticity encourages early management of congenital musculoskeletal deformities in the neonatal period (birth to 28 days of age). A temporary ligamentous laxity is presumed to be present in the neonate because of transplacental transfer of relaxin and estrogen from the mother. In addition to the influence of maternal hormones, the rapid growth of the neonate can foster correction of malalignment if the deforming forces are expeditiously managed. This peak period of hyperelasticity offers pediatric therapists with advanced orthopedic expertise many opportunities to manage congenital joint deformities.175
Intermittent taping of foot deformities (Figure 11-16) has been more adaptable to the nursery setting than either casts or splints and is more effective in achieving mobility than range-of-motion exercises. Access to the heel for drawing blood, inspection of skin and determination of vascular status, and placement of intravenous lines can be accomplished with the tape in place or by temporary removal of the tape as needed. Therapists without a sound knowledge of arthrokinematic principles and techniques should not attempt the taping procedure, which involves articulation of the joint(s) into a corrected position before taping. Other components of the taping process include application of an external skin protection solution under the tape, application of an adhesive removal solution when removing the tape, observance of skin condition and vascular tolerance, development of a taping schedule beginning with 1 hour and increasing by 1-hour intervals as tolerated, and clinical teaching with selected neonatal nurses for continuation of the taping if needed on night shifts and weekends. Infants with congenital foot deformities required shorter periods of casting in the outpatient period after taping of the extremity (Figure 11-17) was implemented during the inpatient phase. Taping is not appropriate for medically fragile infants on minimal handling protocols or for infants younger than 30 to 32 weeks of gestation because of potential epidermal stripping from tape removal or vascular compromise from inadvertent, excessive compression by either the tape or the underwrap layer.
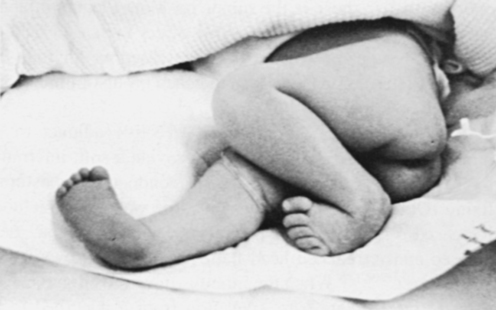

The availability of thin, self-adherent foam material now allows taping on an underwrap (bandage) layer rather than on the infant’s skin (Figure 11-18). Although this method creates a definite advantage in skin protection, it may cover the calcaneal region for blood drawing. Compromise in alignment may occur if the underwrap layer is applied loosely; conversely, restriction in circulation may be observed by edema or purple-blue color changes in the toes if the underwrap is excessively tight around the foot or ankle.
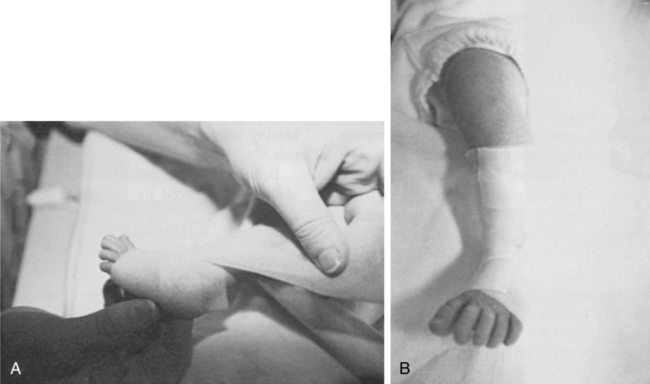

Infants with wrist drop from radial nerve compression related to intravenous line infiltration also benefit from the use of taping (Figure 11-19). The wrist is supported in a functional position of slight extension. As muscle function returns, the taping is used intermittently to reduce fatigue and overstretching of the emerging, but still weak, wrist extensor musculature.
Soft hand-wrist splint
Instead of using rigid, thermoplastic materials or taping, soft foam straps with Velcro closures provide an alternative method of support for wrist drop or hand-wrist malalignment (Figure 11-20). The splinting material is illustrated in Figure 11-21 with a small notch for the thumb on the longer strap placed across the palm and the shorter strap for the wrist band (longer strap attaches to posterior wrist band by Velcro). The wearing time is increased by 1-hour intervals to a 3-hour total wearing time and is often synchronized with nurse caregiving schedules (approximately 3-hour intervals) for alternate on-off application. Collaboration with neonatal nurses on wearing schedule, skin and vascular tolerance, alignment, and parental teaching is critical for successful integration of soft splints into the care plan.
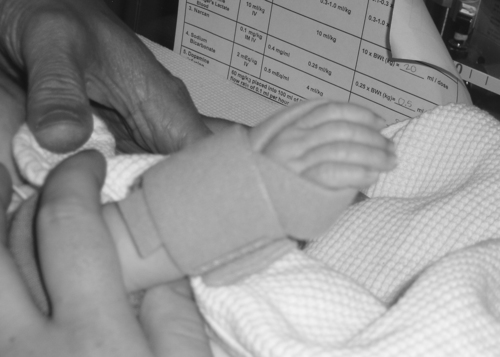

Therapeutic handling
Use of tactile, vestibular, proprioceptive, visual, and auditory stimuli to facilitate infant development has been reported and reviewed by many authors.176–184 Selection and application of the sensory or neuromotor treatment options in neonatal therapy must occur with judicious attention to the prevention of sensory overload and related physiological consequences. Decision making on the type, intensity, duration, frequency, and sequencing of intervention within the context of infant physiological and behavioral stability can be learned only in a mentored clinical practicum in the NICU setting. The current general guidance on intervention is more observation, less handling, protection from bright lights and loud conversation, and readiness for handling determined on the basis of behavioral and physiological cues of the infant.11,177,183,184
Primary aims of therapeutic handling include assisting the newborn to achieve maximal interaction with parents and caregivers and facilitating the experience of postural and movement patterns appropriate to the infant’s adjusted gestational age. Helping infants reach and maintain the quiet, alert behavioral state and age-appropriate postural tone appears to enhance opportunities for visual and auditory interaction and for antigravity movement experiences. The typical early movement experiences include hand-to-mouth movement, scapular abduction and adduction, anterior and posterior pelvic tilt, free movement of the extremities against gravity, and momentary holding of the head in midline.127,185
Behavioral state and some movement abnormalities can be modified by creative swaddling and gentle weight shifts and nesting in the caregiver’s lap. Swaddling the infant in a blanket with flexed, midline extremity position appears to promote flexor tone, increase hand-to-mouth awareness, inhibit jittery or disorganized movement, and elicit quiet, alert behavior. These effects can also be accomplished in skin-to-skin holding of infants against the parent’s chest, a procedure now commonly adopted in NICUs in North America.186,187 Application of neonatal therapy techniques must be contingent on both the infant’s readiness for interaction and the need for a recovery break in interaction because of sensory overload. Teaching parents and caregivers to read and respond to the infant’s motor cues for interaction, feeding, change of body position, and rest breaks is a critical quality-of-life component of the infant’s NICU therapy program.
A semi-inverted supine flexion position (Figure 11-22) with preterm neonates should be used with caution. This position may be used with older infants (6 months old) to facilitate elongation of neck extensor muscles and decrease the neck hyperextension posture, but with neonates the position may compromise breathing from positional compression of the chest and from potential airway occlusion associated with maximal flexion of the neck. The use of cardiorespiratory and oxygen saturation monitors during therapeutic handling activities is recommended for objective measurement of physiological tolerance. Although the peripheral oxygen saturation values from monitors may be intermittently unreliable because of motion artifacts from either the infant’s spontaneous movement or the therapist’s handling of the infant, reliable readings of oxygen saturation may be taken approximately 1 minute after the infant’s body is not moved.
Easily overstimulated preterm infants may not tolerate multimodal sensory stimulation but may instead respond to a single sensory stimulus.79,181 Implementation of a positioning program, oral-motor therapy, and environmental modifications and reinforcement of developmental activities with parents can be instituted only in collaboration with the shifts of bedside nurses who are in charge of the infant’s 24-hour day in the NICU. Collaboration with nurses is a major component of precepted neonatal therapy training and requires integration into and valuing of the unique culture of the NICU.130 Part of NICU culture is the unique ecology of environmental light and sound modifications, medical procedures, equipment, and caregiving patterns. Observing and analyzing the effects of the environment on an infant’s behavior, physiological stability, postural control, and feeding function are critical elements to establish a prehandling baseline status before each neonatal therapy contact.130
Neonatal hydrotherapy
Modified for use in an intensive care nursery setting, the traditional physical therapy modality of hydrotherapy has been adapted and implemented into neonatal therapy programs in some NICUs. Neonatal hydrotherapy was conceptualized in 1980 at Madigan Army Medical Center in Tacoma, Washington, and results of a pilot study of physiological effects were first reported in 1983.188
Indications for referral of medically stable infants to the hydrotherapy component of the neonatal therapy program include (1) muscle tone abnormalities (hypertonus or hypotonus) affecting the quality and quantity of spontaneous movement and contributing to the imbalance of extension in posture and movement (Figure 11-23); (2) limitation of motion in the extremities related to muscular or connective tissue factors; and (3) behavioral state abnormalities of marked irritability during graded neuromotor handling or, conversely, excessive drowsiness during handling that limits social interaction with caregivers and lethargy that contributes to feeding dysfunction.
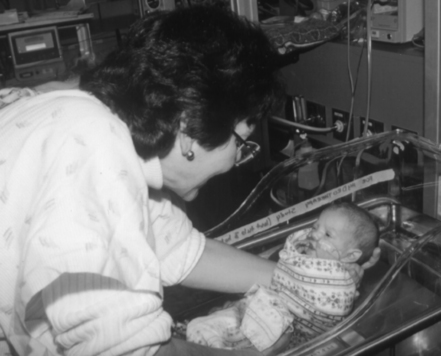

After medical clearance and individualized criteria for the maximum acceptable limits of heart rate, blood pressure, and color changes during hydrotherapy have been received from the neonatal staff, the baseline heart rate and blood pressure values are recorded and pretreatment posture and behavioral states are observed. The undressed infant is swaddled and moved into a semiflexed, supine position. The blood pressure cuff is placed around the distal tibial region to continuously measure heart rate and blood pressure at 2-minute intervals during the 10-minute water immersion period. After being lifted into the water, the swaddled infant is given a short period of quiet holding in the water without body movement or auditory stimulation to allow behavioral adaptation to the fluid environment (Figure 11-24). A second caregiver (e.g., nurse or parent) is recruited to stabilize the infant’s head and shoulder girdle region while the neonatal therapist provides support at the pelvis (Figure 11-25).
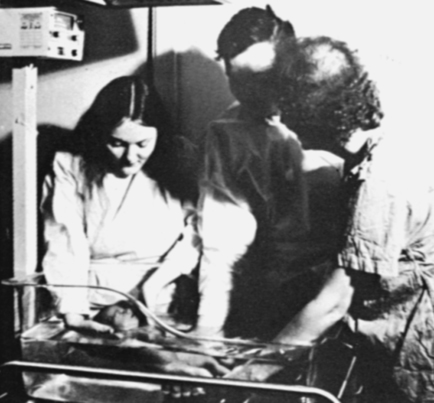

Within the loosened boundaries of the swaddling blanket, the movement techniques involve midline positioning of the head and slow, graded movement incorporating slight flexion and rotation of the trunk, followed (if tolerated) by progression distally to the pelvic girdle region and finally to the shoulder girdle region. After guided trunk extensor flexion with partially dissociated movement at the shoulder or pelvic girdle, most infants will demonstrate active extremity movement in the water and the swaddling blanket is adjusted (or removed) to allow more movement or more stability depending on the response of the infant. The improved range and smoothness of spontaneous extremity movement is facilitated by the buoyancy and surface tension of the water. Movement experiences in the supine, side-lying, and prone positions are offered as tolerated. If the movement therapy becomes stressful, with agitation or crying by the infant, body movement is stopped immediately, and the infant is either consoled or removed from the water and held with warmed towels. Compromise in hemodynamic stability (increased heart rate, increased blood pressure, decreased respiratory rate) and decrease in arterial oxygen tension during crying have been well documented in neonates.189 Careful monitoring of behavioral tolerance to hydrotherapy (with avoidance of crying) is considered critical for reducing physiological risk with hydrotherapy.
Improved sleep quality and behavioral organization were documented in 12 preterm infants (less than 36 weeks of gestation) after a 10-minute hydrotherapy session in a NICU in Brazil.190 In this study the infants were used as their own controls, and responses were measured by a Neonatal Facial Coding System scale and by sleep-wake cycles from an adapted NBAS.
Feeding performance may improve when hydrotherapy is scheduled 30 minutes before feeding to prepare the infant for arousal to the quiet, alert state and for flexed, midline postural changes for optimal feeding. A sample of 31 preterm infants received both a 10-minute hydrotherapy session and a 10-minute rest period control condition (crossover design) before bottle feeding by a nurse blinded to the order of the treatment phase. Mean duration of feeding was significantly decreased (P < .004) after hydrotherapy compared with the rest condition. Mean daily weight gain after hydrotherapy was significantly higher (P < .026) than after the rest condition. All infants consumed 100% of the required feeding volume after the 10-minute hydrotherapy session, indicating that potential overstimulation or fatigue did not occur. On a short-term basis, weight gain was enhanced.191
When oriented to treatment goals and trained in specific hydrotherapy techniques for individual infants, the nursing staff can effectively carry on the hydrotherapy program established by the neonatal therapist. This release of the neonatal therapist’s role to nurses allows additional use of hydrotherapy on evening and night shifts and continued teaching and supervision of parents during evening and weekend visits (see Figure 11-25).
Although many clinical benefits may be obtained by judicious use of hydrotherapy in the newborn nursery, pilot study data obtained on physiological changes in high-risk infants during hydrotherapy clearly indicate a physiological risk.188 This risk (7% increase in blood pressure and heart rate in the pilot sample) must be carefully evaluated relative to each infant’s general medical stability and baseline heart rate and blood pressure status before hydrotherapy can be included safely in a neonatal therapy program. In collaboration with the neonatology and nursing staff, the therapist must use established criteria for general medical stability and the maximal limits during hydrotherapy for blood pressure, heart rate, and acceptable color changes; this step is essential for risk management. Physiological monitoring of mean blood pressure and heart rate with a neonatal vital signs monitor during aquatic intervention is recommended. The blood pressure cuff is a pneumatically driven device that is not electronically connected to the infant and can be safely immersed in water. Because hypothermia is a recognized risk with hydrotherapy, body temperature should be routinely measured before and after the hydrotherapy session by using a thermometer with a digital display. A risk-benefit analysis of the potential physiological risk to each infant and the expected therapeutic benefits is strongly advised before hydrotherapy techniques are incorporated into a neonatal therapy program.
Oral-motor therapy
Feeding difficulties in preterm neonates may be related to neurological immaturity, depressed oral reflexes, prolonged use of an endotracheal tube for mechanical ventilation and subsequent oral tactile hypersensitivity, or insufficient postural tone. Because behavioral state affects the quality of feeding behavior, feeding performance may be significantly improved by specific arousal or calming procedures before feeding. Other variables influencing feeding may include decreased tongue mobility, presence of tongue thrusting, decreased lip seal on nipple, nasal regurgitation, tactile hypersensitivity in the mouth, inefficient and uncoordinated respiratory patterns, insufficient proximal stability from hypotonic neck and trunk musculature, and hypertonic posturing of the neck and trunk in extension.192,193
Three instruments for assessing oral-motor and feeding behaviors in the nursery are the Neonatal Oral-Motor Assessment Scale (NOMAS),194 the Nursing Child Assessment of Feeding Scale (NCAFS),195 and the Early Feeding Skills (EFS) Assessment for preterm infants.196 The NOMAS is used to evaluate the following oral-motor components during sucking: rate, rhythmicity, jaw excursion, tongue configuration, and tongue movement (timing, direction, and range). Tongue and jaw components are analyzed during nutritive and nonnutritive sucking activity. Cutoff scores were derived from a pilot study with the instrument: a combined score of 43 to 47 indicated “some oral-motor disorganization”; a score of 42 or less indicated oral-motor dysfunction.194 The absence of a category to evaluate breathing pattern, work of breathing or respiratory exertion, and physiological variables during feeding limits the use of this instrument to low-risk, healthy neonates.
The NCAFS is used to analyze parent-infant interaction during feeding. It provides a method for evaluating the responsiveness of parents to infant cues, signs of distress, and social interaction opportunities during the feeding process. In concurrent validity studies, NCAFS scores were positively correlated with findings of the Home Observation for Measurement of the Environment inventory at 8 months (r = 0.72) and at 12 months (r = 0.79).195
The EFS Assessment is a 36-item observational measure of oral feeding readiness, feeding skill, and feeding recovery. The assessment tool includes examination of physiological and behavioral stability, behavioral feeding readiness cues, oral-motor coordination and endurance, coordination of breathing and swallowing, and postfeeding alertness, energy level, and physiological state. Preliminary content validity and intrarater and interrater reliability procedures were described as “stable and acceptable” (correlation data not reported) with predictive, concurrent, and construct validity testing in process.196 This tool is specifically designed for feeding examinations in the NICU environment and with expanded psychometric testing will be a relevant instrument in managing neonates with feeding impairment.
General strategies during feeding may include semiflexed, upright positioning with light support under the chin (Figure 11-26). Techniques such as tactile facilitation of the facial muscles, use of a pacifier during gavage feedings, light manual support to the jaw or lip, and thickening of formula are frequent components of oral-motor therapy programs.193,197 Scheduling of feeding based on the infant’s readiness (behavioral cues of hunger and alertness) is usually implemented by neonatal nurses and shown to be effective in helping infants advance the frequency of oral (rather than gavage) feedings.198,199
For some infants, oral intake by bottle may be improved by individualizing nipple selection according to contour, length, hole size, texture, and compression resistance. Wolf and Glass193,200 advised evaluation of the flow rate of liquid from various types of nipples and analysis of the effects of nipple size, shape, and consistency on an infant’s sucking proficiency. Feeding infants in the side-lying position may improve tongue position, particularly if marked tongue retraction is present (Figure 11-27). The timing of movement therapy or neonatal hydrotherapy 30 minutes before feeding may improve performance by preparing postural tone, facilitating oral musculature, and enhancing alertness.
Infants with orofacial anomalies (e.g., cleft palate, hypoplastic mandible) often respond to bottle feeding with a Haberman feeding system (Medela, McHenry, Illinois), which allows control of the flow rate through a valve in the bottle and manual compression of the nipple. The Haberman feeder is an ideal option for infants with large bilateral cleft lip and palate because of the long, flexible nipple, which allows formula to be released from manual compression of the nipple by caregivers instead of requiring negative pressure for suction by the baby (Figure 11-28). Placement of the nipple on the middle of the tongue is advised regardless of the location of the palate or lip defect.201 The feeding performance of infants with severe cleft palate deformities may be improved by a dental obturator. This custom-fabricated prosthesis is inserted before feeding to cover the defect in the palate.
Expected outcomes of oral-motor intervention supported by clinical research include (1) increased number of nutritive sucks after perioral stimulation,202,203 (2) increased volume of fluid ingested during nipple feedings,204 (3) decreased number of gavage feedings and earlier bottle feeding,203,205 (4) accelerated weight gain,205 and (5) earlier hospital discharge.205 Research evidence has emerged on the benefits of interventions to assist pacing to allow breathing pauses206 and to provide slower flow rate with specialized nipples207 for preterm infants during the process of learning to bottle feed.
Monitoring the infant’s physiological tolerance, breathing pattern, and work of breathing is critical during oral-motor examination, intervention, and feeding trials.193 Heart rate values may be monitored from either the cardiorespirograph or with peripheral oxygen saturation from a pulse oximeter. Color changes, diminished tone in facial muscles, and behavioral stress cues (e.g., restlessness, trunk arching) must be carefully monitored to allow appropriate response to early signs of fatigue, overexertion, and potential airway difficulty. Regurgitation with aspiration of milk or formula into the lungs may occur during feeding trials, with complications of pneumonia, cardiopulmonary arrest, and associated asphyxia. Because of these risks, feeding trials should not be attempted by neonatal therapists untrained in managing the respiratory and general physiological monitoring components of neonatal feeding.200
Success during feeding activities enhances parent-infant interaction and perceived competency in parenting. Parents of infants with feeding dysfunction describe higher stress than that reported by parents of infants who are gaining weight and feeding satisfactorily. Oral-motor dysfunction has been reported as an early functional deficit in infants at high risk for later neuromotor sequelae208; early support for parents coping with a challenging feeding situation builds competence in caregiving and also commitment to continuity in outpatient developmental monitoring.
Parental support
Grief process
Strong, continuous support is essential to help parents through perhaps the most frightening crisis in their adult lives—the potential death or disability of their infant.209,210 Although touching and holding infants contribute to infant-parent attachment,211 parents may initially establish emotional and physical distance from the infant “s” as they cope with the knowledge that the infant may die. During this time of anticipatory grief, peer group support from other parents of prematurely born children can be of immeasurable value. Actively listening to the parents’ feelings and concerns and providing support without judgment through their episodes of detachment and anger are critical. Although long-range plans include participation of parents in all aspects of the developmental program, the timing and amount of initial teaching must be individualized to the levels of stress and acute grief present.
Parent/caregiver teaching
Teaching strategies are most effective when they are adapted to the learning style of the parents. This adaptation may involve more demonstrations and an increased opportunity for supervised practice for some parents, particularly those with reading or language difficulties that limit use of a written instructional packet. Parents have shown preference for combined educational methods including demonstration, video, and written materials rather than one single method.212,213 Cultural caregiving practices of the family may necessitate elimination of common procedures such as use of pacifiers for nonnutritive sucking or hand-to-mouth engagement.
With consultation from and in collaboration with the neonatal therapist, neonatal nurses can incorporate recommendations to support skeletal and motor development into their routine discharge teaching activities. General considerations for discharge teaching by nurses may include the following214:
Varying the direction of head turn for sleeping in the supine position to prevent plagiocephaly
Placing the head in midline with lateral rolls extending along the side of the head and trunk for car seats and swings
Limiting the use of infant seats and encouraging the use of prone play on the floor with a roll under the arms and upper chest to assist in head lifting and weight bearing on the arms
Highlighting the importance of the prone play position for strengthening the neck, trunk, and arm musculature to prepare for sitting and rolling
Reinforcing the value of interdisciplinary follow-up for musculoskeletal and neurodevelopmental monitoring
Recommending expedient follow-up if parents notice signs of head flattening, persistent lateral head tilt, strong asymmetrical head turn preference, or asymmetrical arm use